Introduction
Materials and Methods
Plants and treatment
Observation of grapevine buds’ anatomical structure
Soluble sugar, starch, and protein content measurements
DNA extraction and Whole Genome Bisulfite Sequencing (WGBS)
WGBS methylation libraries
Data analysis of whole-genome methylation
Data processing
Results
Effects of cyanamide treatment on grapevine sprouting
Effects of cyanamide treatment on DNA methylation features of grapevine buds
Cyanamide effects on whole-genome DMRs of grapevine buds
Cyanamide effects on DEGs related to DNA methylation of grapevine buds
Discussion
Effects of cyanamide on flower bud differentiation and grape nutrients during dormancy
Effects of cyanamide on DNA methylation features and level of grapevine buds during dormancy release
Effects of cyanamide on gene expression of grapevine buds during dormancy release
Conclusions
Introduction
Deciduous fruit trees’ sprouting, blossoming, and fruiting processes are intricate and require physiological synchronization. Exposure to cold temperatures is essential to effectively transition from dormancy to flowering and fruiting (Guo et al. 2019). Grape is a deciduous woody fruit liana belonging to the Vitaceae family. Its hibernacle requires a certain amount of cold exposure before successful germination. With climate warming in recent years, insufficient low-temperature conditions have caused incomplete and irregular sprouting, leading to the reduction in yield and quality as well as other issues, which have become the bottleneck affecting the development of the grape planting industry (Luedeling et al. 2011). Consequently, there has been a significant rise in interest in the induction of grapevine dormancy by various methods and tools.
Using a dormancy breaker in grapevine buds has been a hot topic over the past decades and is one of the most effective dormancy release methods. Although di-nitrobenzophenol, salicylic acid, gibberellin, and potassium nitrate have positive effects, cyanamide remains the most effective and widely used dormancy breaker (Petri et al. 2014). Despite its toxicity to the human body, Cyanamide possesses both cyanide and amino groups, which exhibit potent properties in ending dormancy, stimulating germination, and insecticidal sterilization. Additionally, it has minimal residue and is readily degradable (Rademacher 2015). Cyanamide has been widely utilized to promote the release of dormancy. It has been commonly used in deciduous fruit trees such as grape, sweet cherry, blackberry, and blueberry (e.g., grape, sweet cherry, blackberry, and blueberry) (Segantini et al. 2015; Ionescu et al. 2017; Wang et al. 2021). Although there are some low-toxic sleep breaking agents, their sleep-breaking effect is far less than Cyanamide, so there are no better low-grade harmless products to replace it. Cyanamide treatment can significantly increase turkey fig yield (Badii et al. 2017) and promote hibernacle sprouting (Petri et al. 2014). However, the underlying epigenetic mechanisms have not been previously investigated.
Epigenetics affects plant growth and development, and DNA methylation is one of the most studied epigenetic modifications (Harris et al. 2018). DNA methylation and demethylation are essential in plant growth, development, vernalization, flowering, and stress resistance (Ma et al. 2018). Epigenetics can be affected by time, cell and tissue differentiation processes, and environmental factors (Hauser et al. 2011; Bilichak et al. 2012). Sokolova et al. (2014) identified that DNA methylation is a factor that directly affects seed germination rate. Moisa et al. (2016) also found that the germination rate of various seedlings is influenced by DNA methylation, which controls gene expression and suppresses transposons in cytosine environments containing CpG, CHH, and CHG. CpG is maintained by MET1 methyltransferase (Jones et al. 2001), while CHG and CHH are regulated by demethylation of the methyltransferase family (Lindroth et al. 2001). However, few studies have been reported on the effects of DNA methylation on the dormancy of grape hibernacles.
This study treated grapevine bud cuttings with 2.5% cyanamide (mass fraction, w%) and clear water. The study aimed to investigate the epigenetic mechanisms causing the release of sprout dormancy in grapevines. This was done by analyzing the whole-genome DNA methylation using techniques such as observing bud germination, examining anatomical morphology, assessing physiological indicators, and using WGBS technology. WGBS was used to detect and analyze the DNA methylation of grapevine buds. The results of this study will be of great significance for the facilitation of grape cultivation in warmer temperate regions.
Materials and Methods
Plants and treatment
Vitis vinifera×labrusca ‘Shuijing’ from the Dongfeng Farm (24.4°N, 103.4°E) in Mille City, Yunnan Province (China) was used in this study. The grapevines of the same age and robust growth were selected. The branches with well-developed bud eyes from the third to seventh segment of one-year-old plants were cut and pruned again into 220 branches with 2 to 3 hibernacles each. The morphological upper end of each branch segment was cut into a flat mouth, and the morphological lower end into an oblique mouth. The 20 vine branches before treatment (BT) were cut and recorded. Eight relatively complete buds were fixed in FAA (formaldehyde: glacial acetic acid: glycerol: 65% ethanol = 5:5:5:85 ). The remaining about 50 buds were flash-frozen in liquid nitrogen and stored at ‒80°C until further use. Then, 2.5% cyanamide (mass fraction, w%) (Cyanamide, CH2N2, purity: Analytically pure, 95%, supplier: RHAWM, Art. No. RH213867) was applied to 100 grapevines (CY) focusing on the bud. The other 100 grapevines were treated with water as the control (CK). The bottoms of the treated grapevine were individually inserted into beakers containing distilled water. Ten grapevine segments were inserted into each beaker, incubated, and sampled in a light incubator with alternating light and dark photoperiods (28/18°C and 90/95% relative humidity with 16/8 h light/dark). November 21, 2022, until December 11, 2022, is the observation period, which lasts for 21 days. The latent phenology of grapevine buds was ascertained by counting the number of buds during this phase, which developed independently one day apart (Sprouting: The scales of the covered bud break, and the immature green leaves stretch) (Lin et al. 2017). The germination rate of 50% decides to break the latency standard, and the germination rate (GR) is the percentage of the number of break buds (B) to the total number of buds (TB). On the seventh day in the incubator (November 27, 2022), the germination of the cyanamide group (CY1) and the water group (CK1) were observed and sampled. On the fourteenth day (December 4, 2022), the germination of the cyanamide group (CY2) and the water group (CK2) were observed and sampled again. During the sampling process, 20 grapevines were chosen randomly from each treatment group, and the buds were removed. Eight buds still in good condition were collected and preserved in FAA solution.
The remaining 50 buds were frozen immediately using liquid nitrogen and stored at ‒80°C. The samples were utilized to ascertain the anatomical structure of the bud, quantify the physiological indicators, and conduct DNA methylation sequencing. This experiment was conducted using three biological replicates. Each biological replicate consisted of twenty grapevines that were sampled. Approximately 50 buds were combined within each set of biological replicates.
Observation of grapevine buds’ anatomical structure
Extract the grapevine bud samples of BT, CY1, CY2, CK1 and CK2 that have been fixed in FAA for 48 h. Proceed with dehydration by rinsing with water for 10 minutes, boiling for 30 minutes, and then dehydrating with 75%, 75%, 85%, 85% and 95% ethanol. Finally, the samples were stored in 1/2 xylene and 1/2 absolute ethanol for 12 h. Transparent (pure xylene). Soak wax (place the small bottle of xylene and material in a 40°C constant temperature water bath, add the broken wax pieces of paraffin: beeswax =20:1 to the vial for several times, keep 40°C constant temperature water bath for more than 24h. Then, pour the sample into a gauze bag and place it in different proportions of wax and xylene mixture in a 58°C ovenEmbedding (quickly remove the sample from the gauze bag and put it into the mold for cooling). Section, spread, and adhesive piece (samples into thin slices on microtome with a thickness of 8–10 µm and dried in a 40°C incubator for 2–3 d). Dewaxed (dip slides in pure xylene, 1 / 2 xylene + 1 / 2 absolute ethanol, 95%, 85%, 75% ethanol for 10min).Staining (the slides were successively immersed in 1% red dye solution for 60h, 5min in 85% ethanol, 0.2% solid green dye solution for 10s, 5min in absolute ethanol, 1 / 2 xylene + 1 / 2 anhydrous ethanol for 10min, and 5min in pure xylene). Seal plate (use a neutral gum sealing plate and put it in a 30–35°C incubator for 3–5d; it shall be permanently sealed for later use). These samples were photographed under an OLYMPUS BH-2 microscope.
Soluble sugar, starch, and protein content measurements
The grapevine bud samples of BT, CY1, CY2, CK1 and CK2 stored at ‒80°C were used to measure soluble sugar, starch, and soluble protein content (Li and Zhang 2016). Soluble sugars were determined using the anthrone-sulfuric acid method. Briefly, the grape sprout samples were ground and dried. Fifty grams of the sample were aliquoted into a centrifuge tube, heat mixed, and centrifuged in 80% ethanol. Then, 10 mg of supernatant was added to a total volume of 10 mL after decolorization using activated carbon. The absorption was measured using a spectrophotometer at 625 nm wavelength, according to the standard curve of the soluble sugar content. Starch content was determined using HPLC evaporation light scattering. For measuring soluble sugar residue, 0.5 g of the sample was mixed with NaOH in an Erlenmeyer flask. HCl was added, and the samples were then boiled. Ba(OH)2 was added to precipitate the sugars completely. One drop of phenolphthalein indicator was stirred, and ZnSO4 solution was added until the solution turned red. Finally, Ba(OH)2 solution was added to restore the reddish color. After filtration, the mixture was diluted to 250 mL, and the amylum content was determined. Protein content was determined using the Coomassie brilliant blue dye-binding method. After placing the ground samples into a centrifuge tube containing 0.5 mL of protease inhibitors in an ice bath for 5–10 min, the samples were centrifuged at 4°C for 5 min at 13,200 r/min. The supernatant was removed and centrifuged repeatedly until no residue was detected. The absorbance value of the supernatant was measured using a spectrophotometer at 620 nm, and the protein content was detected from the standard curve.
DNA extraction and Whole Genome Bisulfite Sequencing (WGBS)
The BT, CY2, and CK2 grapevine bud samples stored at ‒80°C were used for DNA methylation sequencing. Total DNA was extracted from BT, CK2, and CY2 grapevine buds using the QIAamp Fast DNA Tissue Kit (Qiagen, Dusseldorf, Germany). The total amount and mass of DNA in each set of samples were determined using a NanoDrop spectrophotometer (Thermo Fisher Scientific, Waltham, MA). Samples were then fractionated using ultrasound to 300–400 bp before end repair and adenylation. The ultrasonic working frequency is 20–35 KHz, the output power is 2500W, the ultrasonic pulse time is set for 15 cycles (run 30 seconds, stop 30 seconds), and the control sample temperature is 4°C. The DNA fragments (LC Beads 0.7–1.2 ) were then attached to the adapters using the manufacturer’s Accel-NGS methylseq DNA Library Kit (Swift, MI, USA). The bisulfite-treated DNA fragments were subjected to PCR expansion of single-stranded DNA fragments to acquire DNA libraries. Additionally, 2 × 150-bp paired-end sequencing was performed using the Illumina Hiseq 4000 platform (LC Sciences Hangzhou, China). Three biological replicates were conducted for each group (About 50 buds cut from 20 grapevine buds were mixed into a biological repeat).
WGBS methylation libraries
The methylation libraries required the addition of λDNA as a quality control at an incorporation ratio of 5%. WGBS-DNA fragmentation, 3' linker ligation, linker ligation, product purification, library amplification (introduction index) and purification, and library Qubit quantification were performed sequentially.
Data analysis of whole-genome methylation
Clean data were generated after processing the raw WGBS data with cutadapt and Perl scripts to eliminate adapter-containing and low-quality reads with a fraction of N (i.e., unknown base information) greater than 5%. FastQC reads quality software validation was used on the data, followed by WALT for QC validation of the reads in the genome database. After removing duplicate reads, the mapped reads were merged, and the DNA methylation levels were calculated. Differentially methylated region (DMR) analysis was performed using R package-methylKit, and the differentially expressed genes (DEGs) were annotated according to DMRs. Gene Ontology (GO) analysis was then performed using KEGG Orthology-Based Annotation System (KOBAS) software.
Data processing
Data on DNA methylation on every chromosome were derived from genome-wide comparative analyses. The SPSS 19.0 software was utilized to conduct Duncan’s new complex range test (p < 0.05), and Prism8 was employed for graphing.
Results
Effects of cyanamide treatment on grapevine sprouting
Through an examination of the inactive time of grapevine buds, we may make a reasonable assessment of the impact of cyanamide on controlling the growth and development of grapes (Table 1). Compared with the water treatment, the average germination rate of the CY group was 51.24% on day 14 (December 4, 2022) and had passed the latent period entirely, and reached 71.55% by day 21 (December 11, 2022). However, the buds of CK showed no signs of germination on day 14, and the germination rate was only 11.72% on day 21. It can be seen that cyanamide treatment can advance the onset of grapevine bud germination by 8 to 9 d. Grapevine buds are borne in the leaf axils, which are transitional organs of new branches, leaves, and flowers. Outer scales wrap buds and gradually emerge with hairs on the scales during the latent period (Fig. 1A). The axillary bud structure comprises the bud axis, growth point, leaf primordium, young leaf, leaf bud primordium, and scale. Scales enclosed the BT buds and showed no indications of growth, indicating a dormant state (Fig. 1A). From a morphological perspective, CK1 did not exhibit any indications of sprouting.
Table 1.
Observation of grape germination rate after treatment with cyanamide
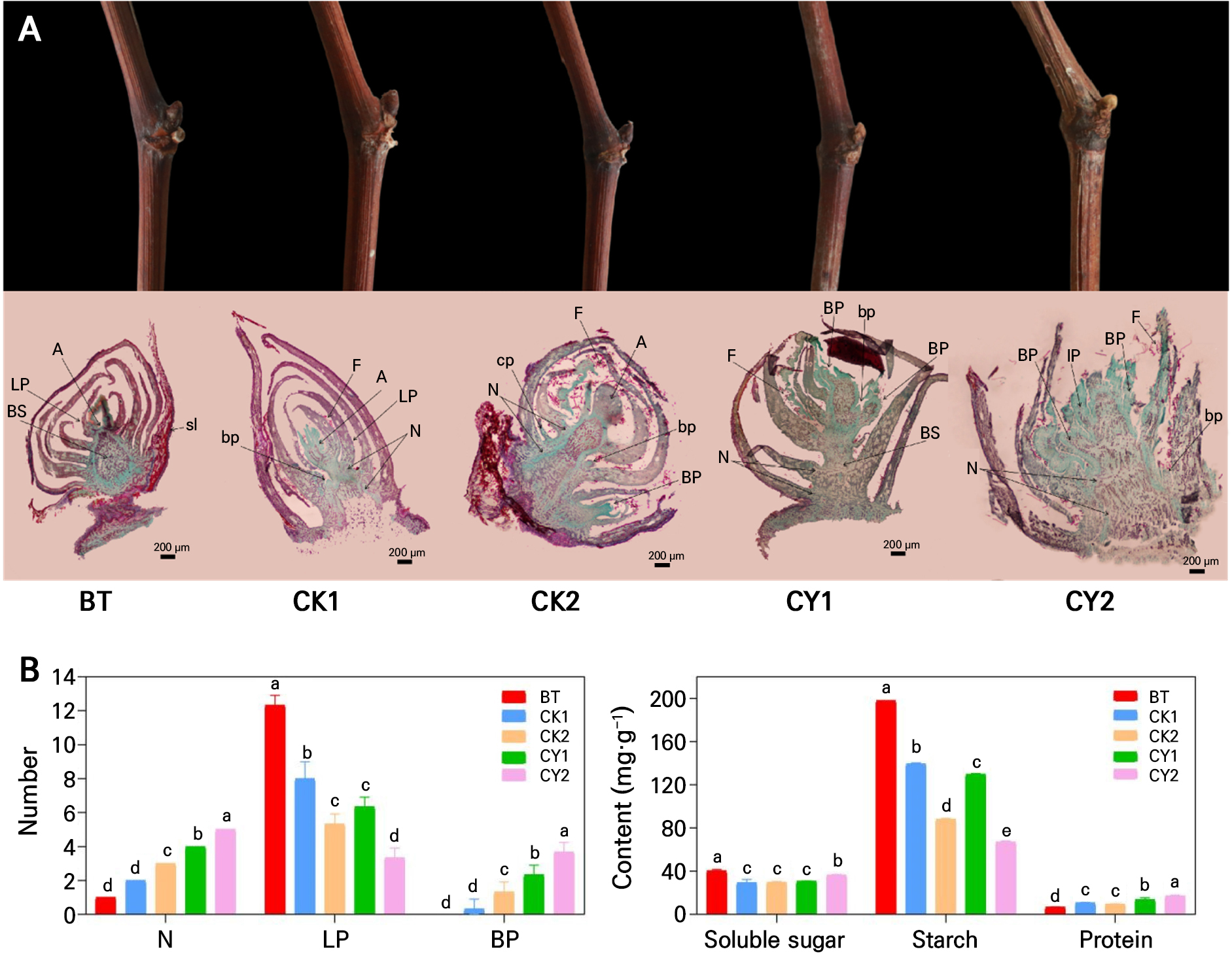
Fig. 1.
Anatomical and physiological characteristics of grape sprouts at different stages of cyanamide treatment. (A): Emergence and anatomical characteristics of grape sprouts; LP - leaf primordium, BP - branch primordium, bp - axillary bud primordium, A- growth point, F - villus, BS - bud axis, cp - tendril primordium, sl - outer scale, N - node of shoot primordium. (B): The effect of cyanamide on the anatomical and physiological characteristics of grape sprouts.
Nevertheless, the paraffin sections revealed the conspicuous growth point and stretched bud axis. CK2 likewise did not exhibit evidence of sprouting. However, the paraffin sections revealed the emergence of lateral bud and branch primordia and the extension of the bud axis and growth points. However, although CY1 did not show signs of visible emergence, the villi at the bud tip began to loosen, and the paraffin section demonstrated the appearance of branch and leaf bud primordia. The outer scales of CY2 had been penetrated, the villi on the bud tip had been fully detached, and clear indications of sprouting were evident. The paraffin sections revealed an enlargement of the bud axis, along with many leaf buds and branching primordia.
Further observation of the anatomical structure of grapevine buds (Fig. 1A and 1B) demonstrated that the bud axis of BT and CK1 did not significantly change. At the same time, CK2 became thicker, and the number of nodes of the embryonic shoots increased from 1 to 3. The CY1 bud axis was significantly longer than in CK2, while the CY2 bud axis was substantially thicker than in CK2. There were up to 6 bud nodes in CY1, which was 2 times higher than the control. Over time, the axillary bud and branch primordia in grapevine buds gradually increased. However, the CY increased significantly more than the CK, suggesting cyanamide promoted grapevine bud development and differentiation. The flower bud primordia demonstrated more branching in the group treated with cyanamide than in the control group. Therefore, it is suggested that cyanamide may accelerate the growth of the bud axis shaft, leading to an increase in the number of flower buds and the overall integrity of the inflorescence. The soluble sugar had a pattern of initial decline followed by subsequent increase. At the same time, starch content gradually reduced. Protein content gradually increased in BT to CK1 and then to CK2 or in BT to CY1 and then to CY2 (Fig. 1B). These findings suggested that during the sprouting of grapevine buds, the production of energy required either the ingestion of carbohydrates or a reduction in their concentration. Furthermore, numerous enzymes were needed for grapevine buds to sprout to catalyze the physiological and biochemical events, increasing protein content. Cyanamide treatment can significantly promote the process of this reaction, thereby expediting grapevine buds sprouting.
Effects of cyanamide treatment on DNA methylation features of grapevine buds
For the reference genome sequence (genome database: ftp://ftp.ensemblgenomes.org/pub/release-54/plants/fasta/vitis_vinifera/dna/) alignment, the ratios of mCG in the BT, CK2, and CY2 groups were 44.47, 43.79, and 44.96%, respectively. The ratios of mCHG in the BT, CK2, and CY2 groups were 20.47, 20.01, and 19.88%, respectively. There were no discernible statistically significant differences (p < 0.05). Compared to the BT and CK1 groups, the mCHH ratios in the CY2 group were considerably (p < 0.05) lower by 59.36 and 48.27%, respectively. Consequently, cyanamide treatment decreased the CHH mC levels, which in turn reduced the overall DNA methylation levels of grapevine buds (Fig. 2A). Additionally, BT, CK2, and CY2 under three kinds of processing grapevine buds (CpG, CHG, and CHH) three types of mCs ratio in the DMR, in turn, exhibited the methylation environment CpG (65.5, 65.7, and 67.8%) > CHG (29, 30, and 30%) > CHH (5.5, 4.3, and 2.3%) (Fig. 2B), respectively. The CHH proportion of CY2 was significantly lower than in BT and CK2. These results suggest that cyanamide treatment reduced mCs proportion in CHH.
The start and stop heterochromatic gene areas with high density were substantially methylated based on the average methylation level of the 19 grape chromosomes (Fig. 2C and Supplementary Table 3). On the other hand, BT > CK2 > CY2 was the general sequence of high to low DNA methylation levels. The methylation levels of CpG and CHG in each of the 19 chromosomes in the three treatments did not significantly differ. However, CHH methylation levels were significantly different, with BT (1.89–4.82%) > CK2 (1.54–3.75%) > CY2 (0.98–1.84%). The CHH methylation levels of the CY2 treatment were 0.91–2.98% lower than in BT, especially on chromosomes 2–5, 10, 13, 15, and 17–19. The CHH levels in CY2 were 0.56–1.90% lower than in CK2. These results suggested cyanamide treatment may break latent grapevine buds by reducing chromosomal CHH methylation levels.
Statistical analysis was performed on the average methylation levels of genes and the flanking regions (2kb upstream of the transcription start site TSS to 2kb downstream of the transcription termination site TES) for BT, CK2, and CY2 groups. The DNA methylation levels of CpG, CHG, and CHH context in the 2kb upstream and downstream regions were significantly higher than within the gene region (Fig. 2D). The genes displayed decreased methylation levels compared to the surrounding areas. Furthermore, BT demonstrated significantly elevated levels of DNA methylation. The methylation levels in the transcript start site (TSS) and transcript end site (TES) regions decreased considerably after cyanamide therapy. These findings showed that cyanamide therapy would significantly lower grapevine buds’ DNA methylation levels, which would mainly impact the CHH context. However, there was no impact on the grapevine buds’ whole-genome methylation mode.
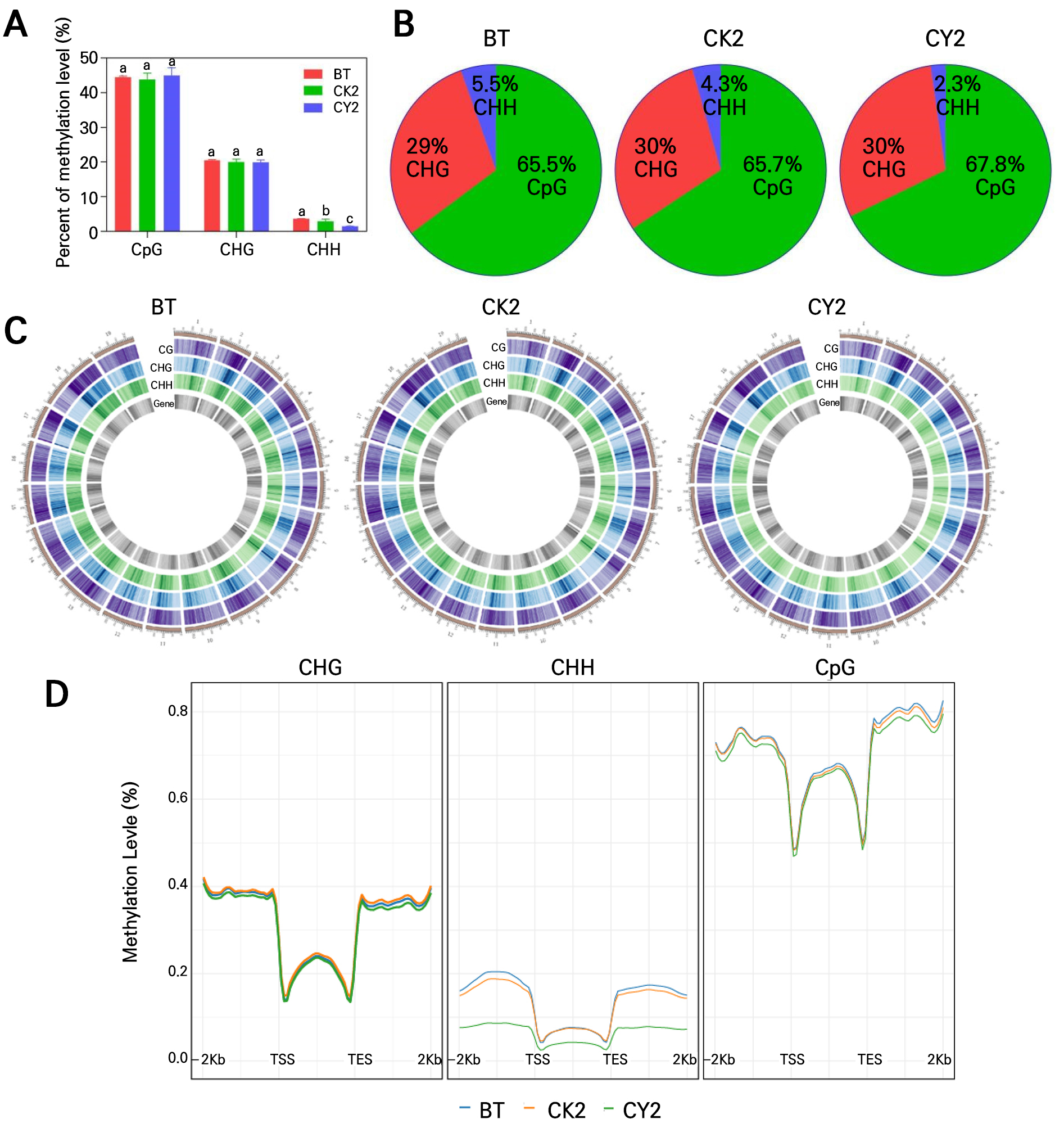
Fig. 2.
Single-base resolution and DNA methylation features in grape sprouts. (A) Cytosine ratio of mCG, mCHG, and mCHH methylation. (B) Relative proportions of mCs of three types (CG, CHG, and CHH methylation) in grape sprouts under different treatments. (C) DNA methylation levels in the 19 chromosomes of grape. The outermost circle is scaled according to the length of the corresponding chromosome. The next three circles denoted the methylation levels of CG, CHG, and CHH in the corresponding chromosome interval (purple, blue, and green, respectively), with darker colors representing increased methylation levels. The innermost circle indicates the number of TE genes in the corresponding interval, with darker colors representing a greater number of TE genes within the region. (D) DNA methylation patterns of the three types of genes and flanking regions in CG, CHG, and CHH.
Cyanamide effects on whole-genome DMRs of grapevine buds
Previous research has demonstrated that cyanamide alleviates dormancy in grapevine buds by lowering DNA methylation levels in their dormant hibernacles. DMRs between BT, CK2, and CY3 were examined to learn more about how cyanamide affected the whole-genome methylation levels of grapevine buds. In CK2 vs BT, CY2 vs BT, and CY2 vs CK2, 873,687, 867,812, and 869,735 DMRs were identified, respectively. The three treatments were ranked as follows:no_diff-DMRs (774,220, 655,042, and 713,331) > down-DMRs (57,310, 193,030, and 139,733) > up-DMRs (42,157, 19,740, and 16,671). CK2 vs BT exhibited no significant differences in the down-regulated or up-regulated genes. At the same time, CY2 vs BT and CY2 vs CK showed significantly more down-regulated than up-regulated genes (Fig. 3A). Among the CpG and CHG context methylation types, the total DNA methylation levels of BT were slightly higher than those of CK2 and CY2. However, the variations were not significant (Fig. 3B). The CHH context methylation type demonstrated that CY2 was significantly higher than CK2 (p < 0.05) and extremely significantly higher than BT (p < 0.01). Cyanimide treatment significantly increased hypo-DMRs but decreased hyper-DMRs. The quantities of hyper and hypo-DMRs in CK2 vs BT, CY2 vs BT, and CY2 vs CK2 were also investigated (Fig. 3C). There were 211,493 hyper-DMRs and 224,587 hypo-DMRs in CK2 vs BT2 and 106,923 and 618,342 hyper-DMRs in CY2 vs BT, respectively. Approximately 85,449 hyper-DMRs and 515,841 hypo-DMRs were present in CY2 vs CK2, respectively. For each of the three treatments, the distribution of hyper- and hypo-DMRs in the promoter, exon, intron, and intergenic regions was examined independently. The DMRs were mostly found in the intergenic regions in all three treatment groups, with promoter, exon, and intron regions following. Hypo-DMRs of CY2 vs BT and CY2 vs CK2 were higher than hyper-DMRs in different areas. There were 97,745, 28,747, 35,101, and 349,826 DMRs for CY2 vs BT and 98,252, 43,338, 39,384, and 249,418 DMRS for CY2 vs CK2 in the promoter, exon, intron, and intergenic regions, respectively. CK2 vs BT showed 1,122 and 29,726 more DMRs in the promoter and intergenic regions (more hypo-DMRs than hyper-DMRs) and 10,740 and 7,014 fewer DMRs in exons and introns, respectively. According to these findings, the hypo-DMRs in the promoter region of dormant grapevine buds were inhibited, causing the plant to enter the dormant state. Treatment of grapevine buds with mono-cyanine prevented gene regulation by increasing hypo-DMRs and decreasing hyper-DMRs; thus, the dormancy of the buds was alleviated. Additionally, among the 19 chromosomes in BT (except for chromosome 10), the methylation levels were significantly higher than those of CK2 and CY2. In contrast, the methylation ratio of CY2 was the lowest (Fig. 3D). Consequently, these findings provide evidence that cyanamide alleviated dormant grapevine buds by enhancing hypo-DMRs.
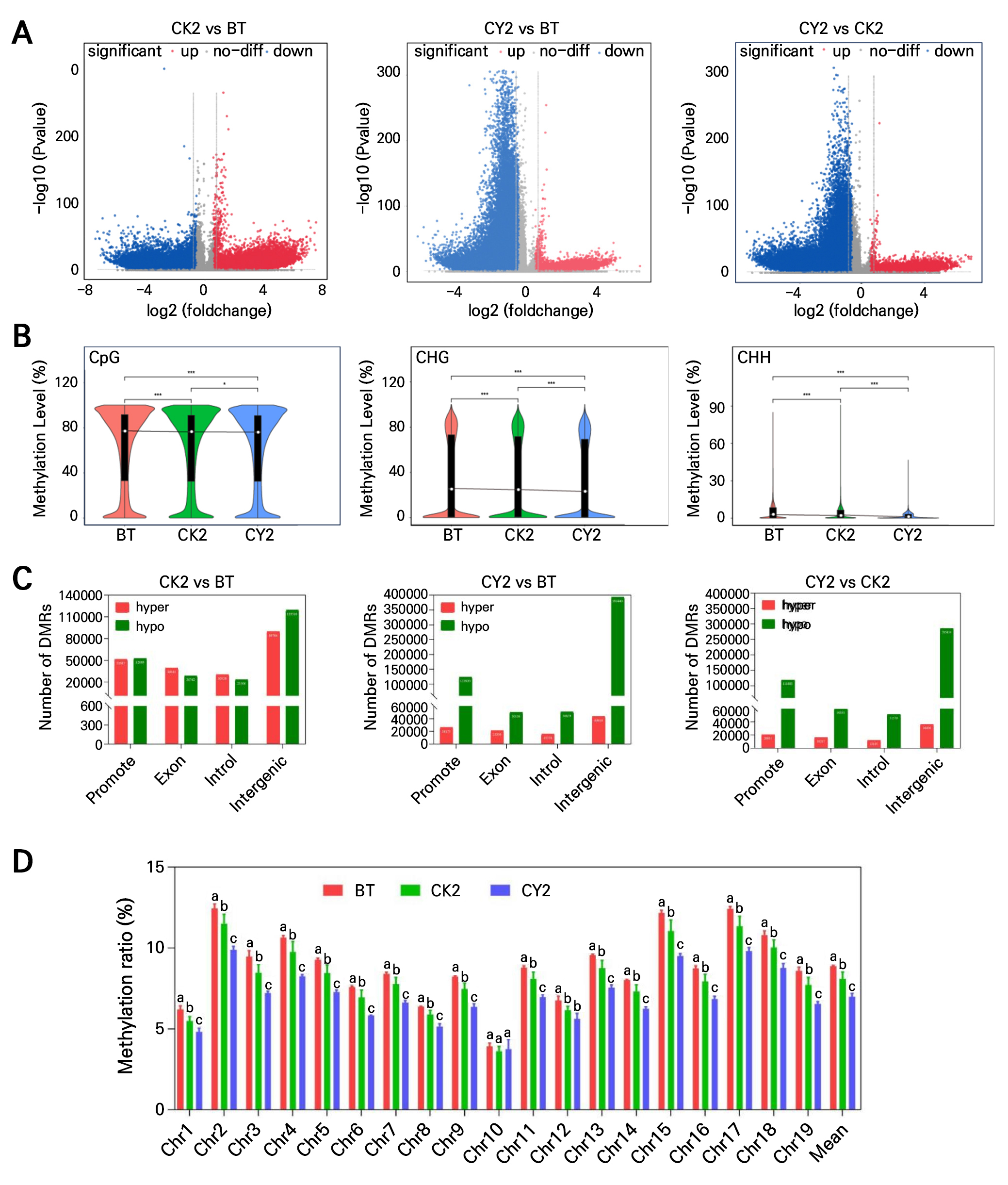
Fig. 3.
Effects of cyanamide on whole-genome methylation DMRs of grape. (A) Volcano plots of DMRs. (B) Distribution of differential DNA methylation genes in CG, CHG, and CHH methylation. (C) The promoter, exons, introns, and gene in the interval DMRs number. (D) DNA methylation ratios on the 19 chromosomes.
The impact of cyanamide on the dynamic variation of DNA methylation levels and the DEGs in grapevine buds was analyzed using GO. A Venn analysis examined the differences in DNA methylation, and overlapping levels of DMR-related genes expressed between CK2 and BT, CY2 and BT, and CY2 and CK2 (Fig. 4A). A total of 44,982, 21,807, and 18,203 hyper-methylated genes were identified from CK2 vs BT, CY2 vs BT. and CY2 vs CK2, respectively. Furthermore, 59,755, 196,720, and 142,912 hypo-methylated genes and 104,647, 218,527, and 161,115 DMRs were obtained. A total of 11,630 DMRs were identified, of which 529 were hyper-methylated. There were 11,101 hypo-methylated genes, accounting for 4.55% and 95.45% of the total DMRs, respectively. Cyanamide, therefore, primarily increases hypo-methylated genes in grapevine buds to relieve dormancy.
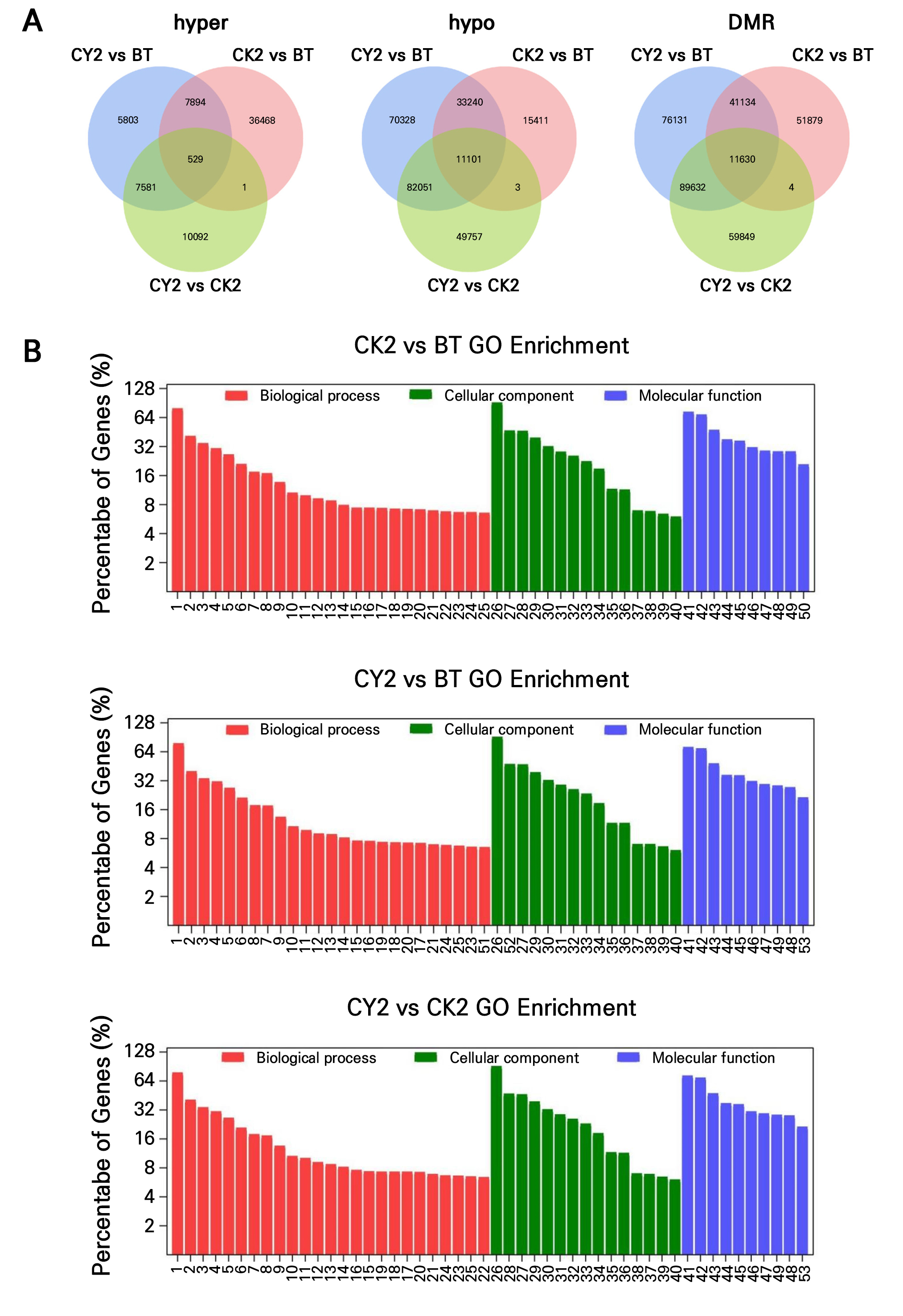
Fig. 4.
Effects of cyanamide on dynamic variation of the DNA methylation level of grape hibernacle and the DEGs. (A) Gene chip common genes, hypo-methylated and hyper-methylated patterns between CY2 vs BT, CK2 vs BT, and CY2 vs CK2, correlation between differential DNA methylation and expression levels of overlapping DMR-related genes. (B) GO enrichment of DMR-related genes in CY2 vs BT, CK2 vs BT, and CY2 vs CK2 (Remarks: 1. biological_process, 2. regulation of transcription, DNA-templated, 3. transcription, DNA-templated, 4. defense response, 5. protein phosphorylation, 6. oxidation-reduction process, 7. secondary metabolite biosynthetic process, 8. signal transduction, 9. positive regulation of transcription by RNA polymerase II, 10. response to salt stress, 11. production of siRNA involved in RNA interference, 12. response to abscisic acid, 13. multicellular organism development, 14. embryo development ending in seed dormancy, 15. flavonoid biosynthetic process, 16. protein ubiquitination, 17. proteasome-mediated ubiquitin-dependent protein catabolic process, 18. translation, 19. proteolysis, 20. response to cadmium ion, 21. response to cold, 22 cell differentiation, 23. response to water deprivation, 24. transmembrane transport, 25. flavonoid glucuronidation, 26. nucleus, 27. plasma membrane, 28. cytoplasm, 29. integral component of membrane, 30. chloroplast, 31. mitochondrion, 32. membrane, 33. cytosol, 34. extracellular region, 35. Golgi apparatus, 36. plasmodesma, 37. endoplasmic reticulum, 38. cell wall, 39. intracellular membrane-bounded organelle, 40. chloroplast stroma, 41. molecular_function, 42. protein binding, 43. ATP binding, 44. DNA binding transcription factor activity, 45. DNA binding, 46. protein serine/threonine kinase activity, 47. RNA binding, 48. sequence-specific DNA binding, 49. metal ion binding, 50. kinase activity, 51. defense response to bacterium, 52. cytoplasm, 53. zinc ion binding).
According to the data provided, it is suggested that cyanamide may alleviate the dormancy of grapevine buds by regulating genes through a process called hypo-methylation. GO enrichment analysis identified 23,373, 25,316, and 24,659 TS genes for CK2 vs BT, CY2 vs BT, and CY2 vs CK2, respectively. In the biological processes, the number of TS genes enriched in CK2 vs BT, CY2 vs BT, and CY2 vs CK2 was higher in the six subclasses of biological process (GO:0008150), regulation of transcription, DNA-templated (GO:0006355), transcription, DNA-templated (GO:0006351), defense response (GO:0006952), protein phosphorylation (GO:0006468), oxidation-reduction process (GO:0055114). The subclasses with the lowest enrichment were response to cold (GO:0009409), transmembrane transport (GO:0055085), response to water deprivation (GO:0009414), and flavonoid glucuronidation (GO:0052696). Cell differentiation (GO:0030154) showed the least enrichment in the CK2 vs BT and CY2 vs CK2 comparisons, with 235 and 234 significantly expressed genes (S genes), respectively. No enrichment was seen in the comparison between CY2 and BT. However, the identification of the defense response to the bacterium (GO:0042742) containing S genes was made instead (Fig. 4B and Supplementary Table 4).
In the cellular components, the top three enriched subclasses of CK2 vs BT, CY2 vs BT, and CY2 vs CK2 were nucleus (GO:0005634), plasma membrane (GO:0005886), and cytoplasm (GO:0005737). The comparisons between CY2, BT, and CY2 and CK2 revealed the presence of at least three subclasses. These subclasses include cell wall (GO:0005618), internal membrane-bounded organelle (GO:0043231), and chloroplast stroma (GO:0009570).
In molecular functions, CK2 vs BT, CY2 vs BT, and CY2 vs CK2 were enriched in ten subclasses of molecular function (GO:0003674), protein binding (GO:0005515), ATP binding (GO:0005524), DNA binding transcription factor activity (GO:0003700), DNA binding (GO:0003677), protein serine/threonine kinase activity (GO:0004674), RNA binding (GO:0003723), sequence-specific DNA binding (GO:0043565), metal ion binding (GO:0046872), and kinase activity (GO:0016301). Therefore, the GO enrichment of CK2 vs BT, CY2 vs BT, and CY2 vs CK2 only demonstrated differences in gene numbers in some pathways, but the overall S gene number showed CY2 vs BT > CY2 vs CK2 > CK2 vs BT. Genes related to molecular functioning, cell composition, and biological processes were activated by prolonging the dormant period. In grapevine buds, however, cyanamide decreased DNA methylation, enhancing the enrichment of associated genes.
Cyanamide effects on DEGs related to DNA methylation of grapevine buds
The methylation-enriched pathways were significantly different (p < 0.1) in different comparison groups (Fig. 5). The biological processes identified in CY2 vs BT include the regulation of transcription, DNA-templated, production of siRNA involved in RNA interference, and positive regulation of transcription by RNA polymerase II. The molecular functions detected include DNA binding transcription factor activity, sequence-specific DNA binding, and ribonuclease III activity (GO:0004525). The cellular component identified is the RISC complex (GO:0016442). Significant enrichment was observed in both CK2 vs BT and CY2 vs CK2. However, lipid catabolic process and SNARE binding were significant in CY2 vs BT. At the same time, the content was minimal in CY2 vs CK2 and CK2 vs BT (Fig. 5). In addition, phospholipid binding and endomembrane system in CY2 vs BT and CY2 vs CK2 showed significant enrichment in CK2 vs BT with low content (Fig. 5). Additionally, only CK2 vs BT was found to be associated with protease activity GO enrichment, such as chitinase activity and protein glycosylation. CY2 vs CK2 almost did not contain lipid catabolic process, an integral component of membrane protein glycosylation. In summary, the findings indicated that cyanamide treatment caused damage to the cell membrane, lipid structure, and protease activity of grapevine buds. This damage facilitated the escape of dormancy and resulted in the early appearance of grapevine bud sprouting.
Discussion
Effects of cyanamide on flower bud differentiation and grape nutrients during dormancy
Perennial deciduous fruit vines require low-temperature exposure for bud break in the spring. However, the warm winters lead to poor flower bud development and negatively impact yield (Shim et al. 2014). DNA methylation changes that are epigenetic can control gene expression and react to external stimuli without changing the DNA sequences (Bond and Finnegan 2007). This work examined the processes by which cyanamide relieves dormancy by lowering DNA methylation levels in grapevine buds using WGBS for the first time.
Sheard et al. (2009) investigated the dormancy of sweet cherries. They found that utilization of dormancy breakers should not only consider the time of cold exposure but also combine this treatment with the appropriate stage of bud development. Cyanamide partially replaced the low-temperature requirement and released grapevine buds dormancy. Flower bud dormancy development has a significant influence (Shim et al. 2014). Cyanamide can be induced by ROS enzyme activity to promote temperate fruit crops to sprout (Pe’rez et al. 2008; Ionescu et al. 2017; Beauvieux et al. 2018), also by rapid regulation of signaling molecules to promote bud sprouting (Lin and Agehara 2021). A thorough investigation has not been conducted into the consequences of the dormancy agent’s sprouting quality. Bound and Jones (2004) state cyanamide encouraged apple flower buds to open early. These results align with the study’s findings, which showed that grapevine buds appeared faster in the treated group after cyanamide therapy than in the control group.
Furthermore, cyanamide treatment promoted the differentiation of grapevine buds, as previously demonstrated in other studies (Ophir et al. 2009; Mohamed et al. 2012). Cyanamide may promote the bud axis, increasing the differentiation of grapevine buds and the integrity of the inflorescence to improve sprouting quality. Previous research has demonstrated that cyanamide improves fruit quality by encouraging consistent bud differentiation and increasing the number of flowers (Sudawan et al. 2016; de Almeida et al. 2017).
The accumulation of soluble sugars in the pentose phosphate pathway promotes dormancy release during the dormancy phase (Beauvieux et al. 2018). Increasing the concentration of soluble sugars in grapevine buds treated with cyanamide can signal bud development, releasing bud dormancy (Roitsch and González 2004; Lin and Agehara 2021). In this study, soluble sugar content in grapevine buds first decreased and then increased at different treatment stages, suggesting that grapevine buds gradually released dormancy over time. These effects were more evident following cyanamide treatment. Couturier et al. (2010) found that aspartic acid, glycine, and other amino acids that synthesize proteins accumulate during poplar bud dormancy, increasing their concentrations at the sprouting stage. In addition, cyanamide promotes the increase of proline, histidine, and other amino acids in the buds of kiwi (Walton et al. 1991) and apple (El-Yazal et al. 2012) to promote sprouting. The current study identified an increase in protein content in grapevine buds following cyanamide treatment, further confirming previous studies’ results. The data suggest that grapevine buds metabolize sugars to generate energy for emergence, leading to a decrease in carbohydrate contents and an increase in protein content. Applying cyanamide treatment facilitated grape sprout germination and efficiently promoted this process.
Effects of cyanamide on DNA methylation features and level of grapevine buds during dormancy release
DNA methylation is one of the most abundant epigenetic modifications in higher plants and plays a vital role in regulating flowering time and other developmental processes (Rodrigues and Zilberman 2015). DNA methylation maintains the epigenetic modifications (Bitonti et al. 2002) and regulates germination and fruit maturity (Narsai et al. 2017). Nevertheless, the methods of DNA methylation in the ‘Shuijing’ grapevine have not been documented. WGBS has been extensively utilized to analyze the methylation of individual bases across the whole genome of tea (Wang et al. 2018), apple (Xu et al. 2018), tomato (Lang et al. 2017), and other plants. Therefore, whole-genome DNA methylation patterns of ‘Shuijing’ grapevine buds were characterized in this study using WGBS. We obtained the DNA methylation profile of the ‘Shuijing’ grapevine buds at the resolution of individual DNA bases. Further investigation was conducted to examine the correlation between the release of dormancy in grapevine hibernacles and the presence of 5-methylcytosine.
Hasbun et al. (2005) analyzed high methylation levels of methylated cytosines in dormant buds. Basdeki and Hagidimitriou (2019) also detected high methylation rates and decreased bud differentiation rates during dormancy. The study found that the methylation level of grapevine winter buds was considerably higher before treatment than after, further confirming prior findings. The whole-genome methylation levels of grapevine buds following cyanamide treatment were significantly reduced, consistent with the results in chestnut (Santamaria et al. 2011), apple (Kumar et al. 2016), almond (Ángela et al. 2018), and poplar (Chen et al. 2021). These findings show that the entire genome has elevated methylation levels during dormancy, which inhibits the production of genes related to sprouting. Conversely, after dormancy is released, the overall low DNA hypo-methylation increases the expression of genes related to germination, which in turn stimulates the sprouting process (Douet et al. 2008).
There are three sequence environments where DNA methylation (mC) can occur: CpG, CHG, and CHH. DNA methyltransferase 1 (MET1) keeps CpG methylation stable during DNA replication. A reinforcing loop of the histone mark (H3K9) and chromatin methylase 3 (CMT3) maintains CHG methylation. RNA-directed DNA methylation (RdDM) and domain-arranged methyltransferase 2 (DRM2) maintain CHH methylation (Rothkegel et al. 2020). In this pathway, non-coding RNAs target homologous DNA sequences for CHH cytosine methylation and CpG and CHG methylation (Law and Jacobsen, 2010).
CHH methylation significantly varies in the biological processes (Li et al. 2019; Guo et al. 2020). Previous studies have reported a gradual increase in CHH methylation levels during development in the seeds of Arabidopsis (Bouyer et al. 2017; Grover et al. 2020), soybean (Lin et al. 2017), and chickpea (Rajkumar et al. 2020). Other studies have found that CHH methylation is high in dormant fruit trees and decreases during germination, including apples (Kumar et al. 2016), almonds (Prudencio et al. 2018), and others. This was further supported by the current study that CHH methylation was significantly reduced among the 19 chromosomal DNA methylation levels in grapevine buds treated with cyanamide. From the overall methylation analysis, the most methylated CpG was detected in this study, followed by CHG and CHH, consistent with previous studies in plum (Rothkegel et al. 2020), apple (Daccord et al. 2017), and sweet cherry (Rothkegel et al. 2020). No significant differences between mCpG and mCHG were detected during seed development. The results demonstrate that, on the one hand, single cyanide ammonia controls grape sprout integrity and stability of the genome via the dynamic regulation of CHH methylation to break dormancy in longan (Chen et al. 2020) and the results of (Ji et al. 2019). Conversely, cytosine methylation leads to gene silence and decreases meristem activity, whereas cyanamide therapy mainly reduces cytosine methylation levels in the CHH setting. The findings indicated that DRM2 and RdDM-regulated genes might significantly impact temperature and the administration of external agents.
Although DNA methylation is primarily observed in transposons, promoters, and transcriptional regions are also frequently methylated and associated with transcriptional activity (Cokus et al. 2008), while gene expression with hyper-methylation in the promoter region is suppressed (Tang et al. 2021). The analysis of DMRs in the current study revealed that the down-regulated genes of CY2 vs BT and CY2 vs CK2 were significantly higher in number than the up-regulated genes. The context methylation patterns of CHH treated with CY2 were considerably more prominent than in CK2 and BT. CY2 significantly increased hypo-DMRs and decreased hyper-DMRs. This is consistent with the findings reported in previous studies on almonds (Prudencio et al. 2018) and sweet cherries (Rothkegel et al. 2020). Hypo-DMRs in CY2 vs BT and CY2 vs CK2 were higher than hyper-DMRs in different regions. Overall, grapevine buds in a dormant state under low expression patterns in the suppressed promoter region result in dormancy. By raising the hypo-DMRs, the single amine reduced the hyper-DMRs to break down gene regulation barriers, thus effectively breaking grapevine buds dormancy. From the lowest methylation rate of CY2 in chromosome 19 in this study, it was further confirmed that cyanamide plays a crucial role in the demethylation and dormancy release of grapevine buds.
Effects of cyanamide on gene expression of grapevine buds during dormancy release
Plant dormancy involves a significant epigenetic modification called DNA methylation. This modification plays a vital role in regulating gene expression, silencing transposons, facilitating chromosome contact, and ensuring the inheritance of traits (Zhang et al. 2016). Xu et al. (2018) found that CHH-type DMRs were enriched in starch and sucrose metabolism, phytohormone signal transduction, and phenylpropanoid biosynthesis when studying apple epigenomic differences. That study further confirmed that methylation status was closely related to gene expression. The current study detected DEGs in grape hibernacles for GO enrichment. Hypo-methylated and hyper-methylated DMRs were further analyzed. The DMRs of CY2 vs BT and CY2 vs CK2 were more differentially expressed than CK2 vs BT (hypo-methylated DMRs specifically), suggesting that DNA methylation plays an essential role in repressing specific genes. Therefore, along with the grapevine buds’ dormancy release, the related biological processes (biological process, regulation of transcription, DNA-templated, transcription, DNA-templated, defense response, protein phosphorylation, and oxidation-reduction process), cellular components (nucleus, plasma membrane, and cytoplasm), and molecular functions (molecular function, protein binding, ATP binding, DNA binding transcription factor activity, and DNA binding) were significantly correlated. The differentially methylated gene expression obtained in this study was consistent with the results of studies in almond (Prudencio et al. 2018). In the meantime, research indicates that DNA methylation plays a role in the development of gene regulation through DMRs and DEG overlap (Bitonti et al. 2002). These findings are similar to the GO enrichment results of this study.
The cluster heatmap (p < 0.1) of GO enrichment genes was used to analyze the differences in the accumulation patterns of different pathways. Lipid catabolic process and SNARE binding were highest in CY2 vs BT. Phospholipid binding and endomembrane system were significantly enriched in CY2 vs BT and CY2 vs CK2. GO enrichment related to protease activity was found only in CK2 vs BT, including chitinase activity and enriched terms for protein glycosylation. However, the lipid catabolic process, a necessary membrane and protein glycosylation component, was nearly missing in CY2 vs CK2. These genes are implicated in phytohormone signal transduction, starch, sucrose metabolism, and phenylpropanoid production, implying that cyanamide can stimulate the release of grapevine buds from dormancy via modulating CHH methylation. This result is consistent with previous studies (Chen et al. 2021). This study further confirms the argument of Ding et al. (2019) that the cell membrane is the first barrier to sprouting. The low-temperature exposure alters protein stability and increases oxidative stress. The break dormancy of methylation in the reduction process was substantially correlated with the phospholipid binding, enrichment of the endomembrane system, and lipid catabolic process following a single chemical treatment of grapevine buds. Early bud break was caused by the single chemical processing that degraded the grapevine bud’s lipid structure, cell membrane, and protease activity.
Conclusions
This study comprehensively analyzed the anatomical, physiological, and epigenetic changes in the ‘Shuijing’ grapevine buds during cyanamide-induced dormancy release. This is the first study to construct single-base resolution DNA methylation profiles of the ‘Shuijing’ grapevine buds induced by cyanamide using WGBS. The findings demonstrated that a 2.5% concentration of cyanamide successfully stimulates the initial growth of grapevine buds during their dormant period. During this process, there was a rise in the levels of starches, sugar, and protein, which facilitated the germination of buds at an early stage and enhanced the quality of flower buds. Cyanamide treatment also primarily reduced grapevine bud’s genome CHH methylation levels, decreasing DNA methylation of the hyper-DMRs and increasing hypo-DMRs, thereby inhibiting the regulation of gene expression. Additionally, GO enrichment analysis identified gene expression of defense response, protein phosphorylation, oxidation-reduction process, cytoplasm, an integral membrane component, protein binding, ATP binding, and DNA binding transcription factor activity, which strongly release grapevine buds dormancy. This study elucidated the underlying epigenetic mechanisms via which cyanamide facilitates the release of dormancy latency, which holds significant implications for grape agriculture in temperate regions.
Supplementary Material
Supplementary materials are available at Horticultural Science and Technology website (https://www.hst-j.org).
- HORT_20250022_Table_S1.pdf
Supplementary Table 1. Summary of whole genome bisμlfate sequencing data
- HORT_20250022_Table_S2.pdf
Supplementary Table 2. Comparison of reference genome data
- HORT_20250022_Table_S3.pdf
Supplementary Table 3. The mean methylation level of the whole genome and each chromosome (%)
- HORT_20250022_Table_S4.pdf
Supplementary Table 4. Number of genes with significant co-enriched pathway differences
- HORT_20250022_Figure_S1.pdf
Supplementary Fig. 1. Replicate and differential principal component analysis of samples from different treatments.