Introduction
Materials and Methods
Source of Samples
Handling of Samples
Reagents
Determination of the Starch Content
Determination of the Moisture Content
Determination of the Crude Fat Content
Determination of the Crude Fiber Content
Determination of the Polysaccharides Content
Determination of the Leachate Content
Determination of the Ash Content
Determination of the Soluble Protein Content
Determination of the Content of Macamides Using HPLC
Determination of the Content of Alkaloids
Determination of the Content of Flavonoids
Determination of the Content of Glucosinolates
Data Analysis
Results
Effects of Different Varieties on the Quality of Maca
Effects of Different Processing Methods on the Quality of Black Maca
Effects of Different Harvesting Times on the Quality of Black Maca
Effects of Different Storage Periods on the Contents of Bioactive Components of Black Maca
Principal Component Analysis of Quality Indicators of Black Maca according to the Processing Method
Discussion
Conclusions
Introduction
Maca (Lepidium meyenii Walpers), is an annual or biennial plant of the genus Lepidium of the Cruciferae family, native to the Andes Mountains of South America at an altitude of 3,500 meters in Peru (Xia et al., 2018). In the mid-to-late 20th century, the Food and Agriculture Organization of the United Nations called for the widespread cultivation of maca around the world. In 2002, China allowed maca to be introduced for planting. At present, maca has been successfully introduced in Yunnan, Guizhou, Qinghai, Xinjiang, Tibet, Sichuan, Jilin, Shanxi and other Chinese provinces (Sun et al., 2011). In 2011, the Ministry of Health approved maca as a new resource food. In 2015, the planting area of maca in China exceeded 600,000 acres. The geographical location and climatic conditions of Yunnan Province are suitable for the growth of maca. At present, maca is planted on a large scale in Lijiang, Qujing, Zhaotong and other areas. Maca is rich in nutritional components such as polysaccharides, soluble protein, starch, crude fat, as well as bioactive components, including macaene, macamides, glucosinolates, alkaloids, and sterols (Carvalho and Ribeiro, 2019; You et al., 2021). These components display a series of biological activities both in single and combination model systems of maca, promoting reproductive health, anti-oxidation, anti-fatigue, endocrine regulation, fertility and immunity enhancements, and nervous system protective and blood sugar regulation activities (Zha et al., 2021; Fei et al., 2022; Ragab et al., 2023). These benefits are due to the synergistic interaction of the nutritional and bioactive components of maca.
Different drying methods can have varying effects on the appearance and active ingredients of maca. There are currently three commonly used drying methods for maca: Natural air-drying, hot air-drying and vacuum freeze-drying. Natural air-drying is a simple and cost-effective method that uses low drying energy levels and causes little change in appearance, but it can be restricted by external conditions. Hot air-drying and vacuum freeze-drying are more convenient and can maintain the color of maca during the drying process, though researchers have found that the types and content of macamides and fatty acids were higher in naturally air-dried maca compared to freeze-dried maca (Esparza et al., 2015; Xia et al., 2021). Other work has shown that different drying methods have different effects on the contents of medicinal extracts and the active ingredients of medicinal herbs (Chen et al., 2021). The content of macamides was found to be highest in the naturally air-dried samples. It was suggested that natural air-drying can enrich more macamides (Esparza et al., 2015; Xu et al., 2021).
The thickness of maca slices can affect the drying rate as well as the content of the bioactive compounds in maca. Research shows that the thicker the slice, the slower the drying process, and relatively more rosmarinic and salvianolic acids are found when the slice is 0.2–0.3 cm thick. Thicker slices tend to have slower drying rates, with thinner slices tending to dry rapidly (Wang et al., 2016). However, thin slices can result in low-quality products due to breakage during slicing and drying.
In order to improve the efficiency and quality of maca, this study will investigate the effects of different varieties, processing methods, harvesting times and storage periods on the active components of maca using when dried hot air-drying and vacuum freeze-drying methods. The results here can provide insight into how to produce high-quality maca products.
Materials and Methods
Source of Samples
The samples used in this study were collected from the south-western region of China at 26°N latitude, where maca is transplanted with a plant spacing of 17–30 cm. During the growth period, the plants are watered in a timely manner and fertilized with nitrogen, phosphorus, and a potassium compound fertilizers. Mid-tillage and soil loosening are carried out 3–4 times, and weeds are removed manually. The samples used in this study were black maca collected at different storage periods, in this case zero, one, two and three years, harvested in 2019, 2018, 2017 and 2016, respectively. Some black maca samples were collected on November 26, December 12, and December 27, 2016 (representing different traditional Chinese solar terms of light snow, heavy snow and winter solstice), and some other samples were collected on November 29, December 13 and December 25, 2018 (representing the four traditional Chinese solar terms of light snow, heavy snow, winter solstice and small cold). Black maca and yellow maca were planted at corresponding altitudes of 3,000 m and 2,540 m, and all samples were collected after one year of culturing. They identified as maca by researcher Liu Cong of the Institute of Medicinal Plants at the Yunnan Academy of Agricultural Sciences.
Handling of Samples
The samples were washed and dried to remove surface water before being sliced according to the specific slicing requirements of each processing method. The drying process was carried out at a constant temperature until the samples were fully dry, following the temperature requirements outlined in Table 1.
Table 1.
Different maca processing methods
Reagents
Acetonitrile is a chromatographic grade reagent. All other chemical reagents used are of analytical grade.
Determination of the Starch Content
Each maca sample was washed with ether to remove fat and 85% ethanol to eliminate soluble sugar. The residue was gelatinized by heating the samples in a boiling water bath for 15 minutes and then cooling them to below 60°C. An amylase solution was added and stirring was then conducted for one hour at 55–60°C. To check for completion of the reaction, a drop of the solution was combined with a drop of iodine solution, with the absence of blue color indicating that the reaction was finished. If blue color appeared, the solution was once again heated to gelatinize it and additional amylase solution was added until the blue color disappeared. The solution was then mixed with alkaline copper tartrate solutions A and B, and 0.1 mol potassium permanganate standard solution was titrated until a reddish endpoint was reached. The same method was used for the reagent blank experiment by measuring a certain amount of water and the same amount of amylase solution used during the processing of the samples.
Determination of the Moisture Content
The crucibles used were washed and dried at 100°C for 1 h. Each sample was weighed and placed in the crucible before drying at 100°C. The weight before and after drying was recorded to calculate the moisture content.
Determination of the Crude Fat Content
The sample was extracted with a fat solvent (i.e., ether or petroleum ether) using a Soxhlet extractor. The extracted substances were a mixture of different fat compounds, including free fatty acids, phospholipids/esters, sterols, pigments of aromatic oils, and organic acids. Then, the crude fat content can be obtained through calculating.
Determination of the Crude Fiber Content
The sugar, starch, pectin and hemicellulose in the sample were hydrolyzed and removed with H2SO4. The remaining residue was treated with alkali to remove protein and fatty acids, leaving behind crude fiber. Finally, the content of crude fiber in the sample was calculated.
Determination of the Polysaccharides Content
Maca powder was accurately weighed at 5.0 g with a material-liquid ratio of 1:20, an extraction temperature of 45°C and an extraction time of one hour to extract the polysaccharides. This was repeated three times. Polysaccharides are determined by phenol sulfuric acid colorimetry (Dubios et al., 1956). The content of maca polysaccharide is determined by phenol sulfate colorimetry. After weighing the glucose when dried to a constant weight, a glucose standard solution was prepared. Different volumes of standard solutions were added to test tubes with stoppers and distilled water was added up to 2.0 mL. Then, 1.0 mL of freshly prepared 6% phenol was added, followed by the slow addition of concentrated sulfuric acid. After reacting for 15 min and boiling for 10 min then measuring the absorbance value at 490 nm. The absorbance values were plotted to obtain a standard curve. The absorbance value of maca polysaccharide was measured at 490 nm according to the standard curve determination method, and the content of maca polysaccharide was then calculated.
Determination of the Leachate Content
First, an accurate quantity of the maca powder was weighed and placed in a ground-mouth conical flask or round-bottomed flask. Then, 50% ethanol was added and the bottle was sealed and allowed to stand for one hour at room temperature. The solution was condensed and refluxed for one hour and was then cooled to room temperature. The weight of the bottle before and after the addition of ethanol was compared to make sure it was appropriate. The solution was then centrifuged and 25 mL of the supernatant was taken and put into a constant-weight petri dish, which was dried in a constant-temperature blast drying oven and weighed after cooling. Finally, the leachate content of the maca sample was calculated based on the weight of the petri dish.
Determination of the Ash Content
A preheated and cooled crucible was weighed. The sample was charred on a Bunsen flame in a fume cupboard. The charred sample was placed into a muffle furnace set to 550°C for two hours until white or light gray ash was obtaining. The sample was then removed, cooled in a desiccator, and then weighed to calculate the ash content.
Determination of the Soluble Protein Content
A 1.0 mg/mL standard protein solution was prepared with bovine serum albumin which distilled water. A specified amount of Coomassie brilliant blue G-250 reagent was weighed, dissolve in 95% ethanol, 85% phosphoric acid and dilute with a specified amount of distilled water was added to prepare the G-250 solution. The final concentrations of the three solutions are 0.01%, 4.7% and 8.5% (w/v) respectively. Six different volumes (0, 0.2, 0.4, 0.6, 0.8, 1.0 mL) of standard protein solutions were placed in stopper tubes, after which distilled water was added in each case up to 1.0 mL. Next, 5 mL of the G-250 solution was added to each tube, followed by thorough mixing. After reacting for two minutes, the absorbance value was measured at 595 nm, and this value was input to obtain the standard curve.
0.2 g of sample was weighed and placed in a pre-cooled mortar, after which distilled water was added in three portions and was quickly ground to a homogenate in the same direction in an ice bath. It was then poured into a 2 mL centrifuge tube. The benchtop high-speed refrigerated centrifuge was set to 4°C at 12000 rpm for 20 minutes. The supernatant, the solution to be tested, was then taken out. Measuring the absorbance value of the maca sample at 595 nm. The soluble protein content in maca was then calculated according to the previously obtained standard curve.
Determination of the Content of Macamides Using HPLC
In this step, the chromatographic conditions were as follows: column (Agilent 5 TC-C18(2), 250 mm×4.6 mm, 5 µm); mobile phase: acetonitrile (B) –0.05% aqueous phosphoric acid solution (A); gradient elution: 0–15 min, 90%–95%B; 15–50 min, 95%B. In addition, the detection wavelength was 208 nm, the flow rate was 1.0 mL/min, and the column temperature was 30°C. The maca powder was placed in a conical flask with a stopper and 10 mL of anhydrous ethanol was added, after which this was weighed. Extraction was then done with ultrasound for 45 minutes and the sample was left at room temperature. Subsequently, it was weighed again and anhydrous ethanol was added to compensate for the weight loss. Finally, it was subject to shaking and was then filtered through a 0.45 µm microporous membrane. The resulting filtrate is the sample solution, which can be directly analyzed by HPLC.
Determination of the Content of Alkaloids
The alkaloids in the maca were extracted by Soxhlet extraction. First, the dried maca powder was weighed and 0.5% of a hydrochloric acid-ethanol solution was added. Extraction was then done in a water bath at a temperature of 90–100°C. The extraction was repeated three times. Bromophenol blue developer (2.0×10-4 mol/L) and pre-prepared chloroform (pH7.0) were added to the extract. The chloroform layer was removed, and anhydrous sodium sulfate was added. After shaking the sample and letting it stand for ten minutes, the absorbance was measured at the maximum absorption wavelength. A standard curve was prepared using berberine hydrochloride as a control substance, and the alkaloid content in the maca was calculated.
Determination of the Content of Flavonoids
The flavonoids were determined by the aluminum chloride colorimetric method. First, we accurately weighed the maca and extracted it with chloroform and methanol in sequence until it became colorless. We then evaporated the extract and dissolved it in dilute ethanol, after which we added a 5% NaNO2 solution and a 10% Al(NO3)3 solution for six minutes. NaOH solution was then added for 15 minutes and the absorbance was measured at 500 nm using a UV-visible spectrophotometer. The content of the flavonoids was determined using rutin as the standard substance.
Determination of the Content of Glucosinolates
In this step, we weighed a certain amount of maca powder and placed it in a beaker, added 20 mL of methanol, and extracted it at room temperature for ten minutes. We then centrifuged the water extract at 8000 rpm for the minutes, removed the supernatant, and filtered it. We subsequently concentrated the filtrate using a rotary evaporator and dissolved the dried solid in water. Next, we accurately pipetted 2.0 mL of the solution into a test tube and added 4.0 mL of a 0.15% CMC solution. After shaking well, we added a palladium chloride solution and continued to shake the sample for four hours at 25°C. We used a H2O-PdCl2-CMC blank solution set to zero and measured the absorbance at a wavelength of 540 nm. A standard curve was prepared using sulforaphane standard and the content of sulforaphane was calculated in the different maca extracts.
Data Analysis
All experimental data were organized and analyzed using SPSS 19.0 software for standard deviation, and Duncan’s multiple range test was used to analyze the significance of the differences between the values. The mean with a confidence limit of 95% (p < 0.05) was analyzed using GraphPad Prism 8 software for plotting. SPSS 19.0 statistical software was used to standardize the original data of the nutritional components and bioactive components of maca, and then principal component analysis(PCA) was conducted.
Results
Effects of Different Varieties on the Quality of Maca
Effects of different varieties on nutritional components of maca
At the same altitude, yellow maca contains relatively high contents of polysaccharides, starch, crude fiber, leachate and soluble protein, while black maca has higher contents of crude fat and moisture. For the same variety, the contents of polysaccharides, starch, crude fiber, leachate and soluble protein were higher in maca from an altitude of 3,000 m as compared to those from plants at an altitude of 2,540 m, while the contents of moisture and ash were lower. The contents of polysaccharides, starch, crude fiber, leachate, and soluble protein of yellow maca at an altitude of 3,000 m were highest at 8.26%, 19.87%, 11.81%, 37.06% and 2.3%, respectively. The contents of crude fat and moisture of black maca at an altitude of 2,540 m were highest at 2.75% and 8.51%, respectively. The highest ash content of 6.04% was found in yellow maca at an altitude of 2,540 m (Table 2).
Table 2.
Effects of different varieties and altitudes on the nutritional components of maca (%) (n = 6)
Effects of different varieties on the bioactive components of maca
Yellow maca has higher contents of N-benzyl linoleamide, N-benzyl linolenamide and N-benzyl hexadecanamide compared to black maca, with these rates being 0.053%, 0.03% and 0.032%, respectively. The contents of flavonoids, glucosinolates and alkaloids are also higher in yellow maca than in black maca, but without significant differences; these contents are 0.590%, 0.666% and 0.184%, respectively. It is interesting to note that the contents of alkaloids, glucosinolates, flavonoids and macamides show a decreasing trend in turn (Fig. 1).
Effects of Different Processing Methods on the Quality of Black Maca
The effect of different processing methods on the nutritional composition of black maca
According to the three drying conditions and the temperature (pressure) and thickness specifications, different processing methods are adopted. The dried sections of maca that underwent different processing methods are shown in Fig. 2.
Under the same temperature, the nutritional composition of black maca varies with the thickness of the dried sections. When comparing the thickness, the ash and moisture contents are found to be lowest in the 2 mm sliced dried black maca, while the crude fat and leachable contents are highest in the 4 mm sliced dried black maca. In the 6 mm sliced dried black maca, the crude fiber content is highest and the crude fat content is lowest. The highest contents of polysaccharides and starch are in the 8 mm sliced dried maca, and the highest contents of soluble protein, moisture, and ash are in whole black maca. However, the lowest contents of starch and crude fiber are found in whole black maca. When the thickness is the same, a lower drying temperature leads to decreases in the contents of polysaccharides, starch, leachate and soluble protein, while the contents of crude fat, crude fiber, moisture and ash show increases. When comparing the drying temperature, with the same thickness, black maca dried at 55°C has the highest contents of starch, polysaccharides, leachate and soluble protein, while that dried at 35°C has the highest contents of crude fat, crude fiber, moisture and ash (Table 3). Finally, when comparing the pressure, an increase in the pressure leads to increases in the contents of polysaccharides, crude fat, leachate and ash, whereas the contents of starch, crude fiber, and soluble protein decrease. At 0 Pa, the contents of starch, crude fiber, and soluble protein are highest; at 20 Pa, the moisture content is highest, and at 40 Pa, the contents of polysaccharides, crude fat, leachate and ash are highest (Table 4).
Table 3.
Nutrient contents of different maca processing methods (%) (n = 6)
Table 4.
Contents of various nutrients in maca when the temperature and slice thickness remain unchanged (%) (n = 6)
Effects of different processing methods on the contents of the main bioactive components of black maca
Specifically, at a temperature of 55°C, the whole dried black maca has the highest contents of flavonoids and alkaloids, while the contents of macamides and glucosinolates have the lowest levels. Increasing the slice thickness tends to increase the contents of flavonoids and alkaloids, though it reduces the content of glucosinolates. The content of black macamides increases first and then decreases with an increase in the slice thickness at 55°C, reaching the maximum value at a slice thickness of 8 mm. The contents of flavonoids and alkaloids are highest in the whole dried black maca at 55°C. Additionally, the contents of N-benzyl hexadecanamide and N-benzyl linoleamide are the highest in the whole black maca dried at 35°C, while glucosinolates is the highest in the 2 mm sliced maca. Vacuum freeze-drying tends to reduce the contents of alkaloids and flavonoids, but this method increases the content of glucosinolates in the 8 mm sliced black maca compared to hot air-drying (Fig. 3).
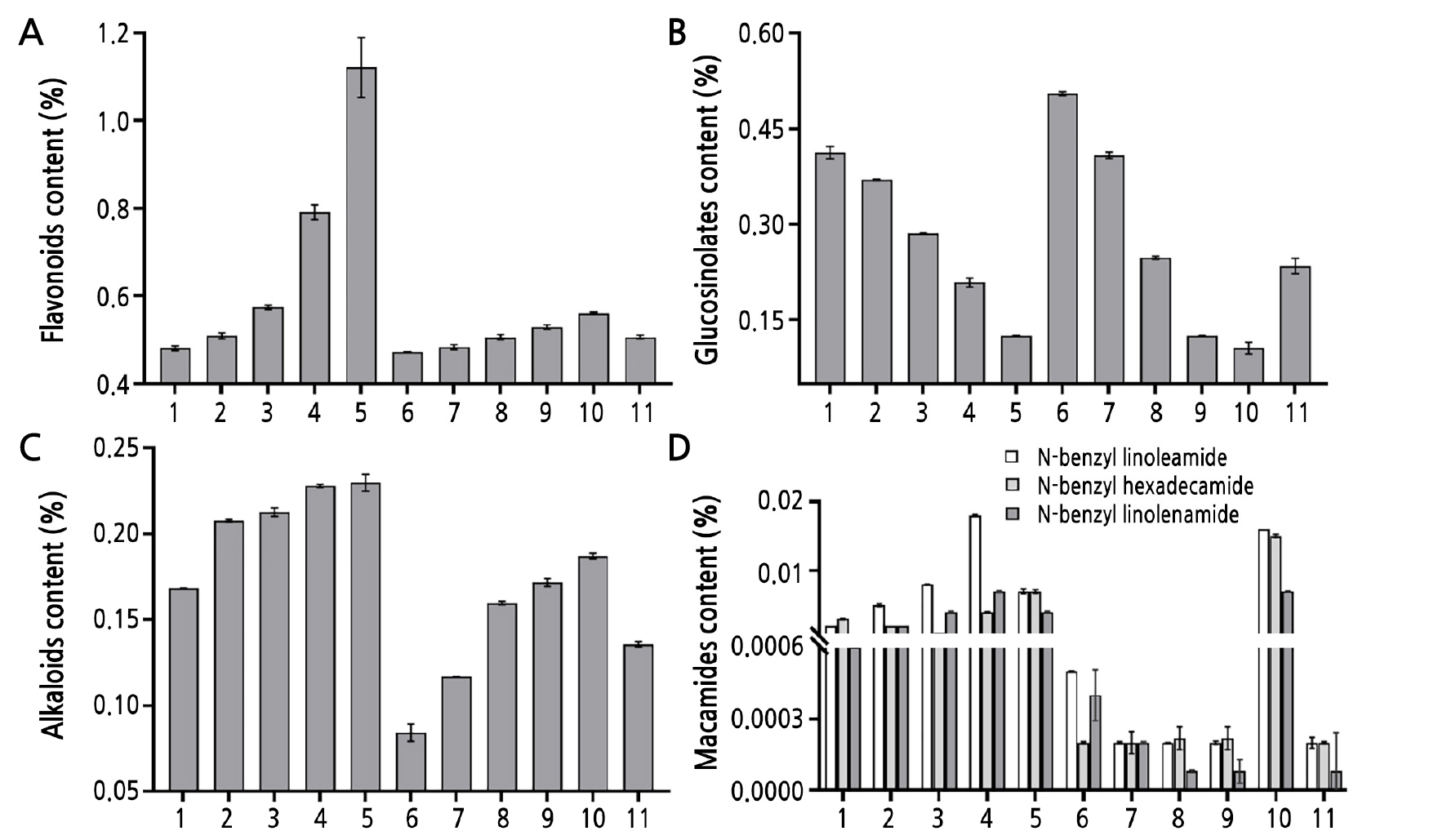
Fig. 3.
Effects of different processing methods on the contents of flavonoids (A), glucosinolates (B), alkaloids (C) and macamides (in this case N-benzyl linoleamide, N-benzyl hexadecanamide and N-benzyl linolenamide) (D) in maca. 1–11 in the diagram denote the different processing methods. Vertical bars indicate the standard error (n = 3).
Effects of Different Harvesting Times on the Quality of Black Maca
Effects of different harvesting times on the nutritional components of black maca
Among the three harvesting times, the nutritional component contents of leachate, starch, polysaccharides, crude fiber, moisture, ash, soluble protein and crude fat gradually decrease. As the harvesting time is delayed, the contents of polysaccharides, starch, crude fat, crude fiber, leachate, soluble protein, moisture and ash in maca gradually increase. The maca harvested on December 27, 2016 had the highest of the aforementioned nutritional component contents at 12.84%, 23.82%, 2.8%, 10.06%, 38.86%, 2.83%, 9.69% and 7.97%, respectively (Table 5).
Table 5.
Effects of different harvesting times on the nutrient contents of maca (%) (n = 6)
Effects of different harvesting times on the contents of bioactive components in black maca
Among the three harvesting times, the flavonoid compounds showed the highest levels among all bioactive components, followed by alkaloids and glucosinolates, whereas the amounts of macamides were found to be lowest. The contents of N-benzyl linoleamide, N-benzyl linolenamide and N-benzyl hexadecanamide in the black maca collected On December 25, 2018 were higher than those in the black maca collected on the other days, at 0.012%, 0.011% and 0.004%, respectively. There were no significant differences in the contents of N-benzyl linoleamide and N-benzyl hexadecanamide in the maca collected on November 29, 2018 and December 13, 2018. With an extension of the harvesting time, the contents of flavonoids, glucosinolates and alkaloids tended to increase. The contents of flavonoids, glucosinolates and alkaloids in the maca collected on December 25, 2018 were highest at 0.556%, 0.149% and 0.229%, respectively (Fig. 4).
Effects of Different Storage Periods on the Contents of Bioactive Components of Black Maca
Effects of different storage periods on bioactive components of black maca
From 2016 to 2019, a certain amount of fresh maca was collected during each harvest season, cut into slices about 8 millimeters thick, and dried with hot air at 55°C. After drying, all of the maca from the storage periods was ground into powder for testing. The storage times of the maca collected in 2016, 2017, 2018, and 2019 were set to three years, two years, one year, and zero year, respectively.
When the storage period of black maca is zero year, the contents of N-benzyl linoleamide, N-benzyl linolenamide and N-benzyl hexadecanamide were significantly higher than those from the other storage periods, at 0.132%, 0.052% and 0.058% respectively. After two years of storage, the contents of the three macamides ranked second, with no significant difference between three years and one year of storage. The contents of flavonoid compounds in black maca gradually increase when the storage period is three years, two years, one year and zero year, but the difference is not significant in any case. When the storage period is zero year, the content of flavonoids in black maca is highest at 0.556%. As the storage period decreases from three years to zero year, the contents of glucosinolates and alkaloids in black maca gradually increase. These contents were highest when the storage period was zero year, at 0.489% and 0.237%, respectively (Fig. 5).
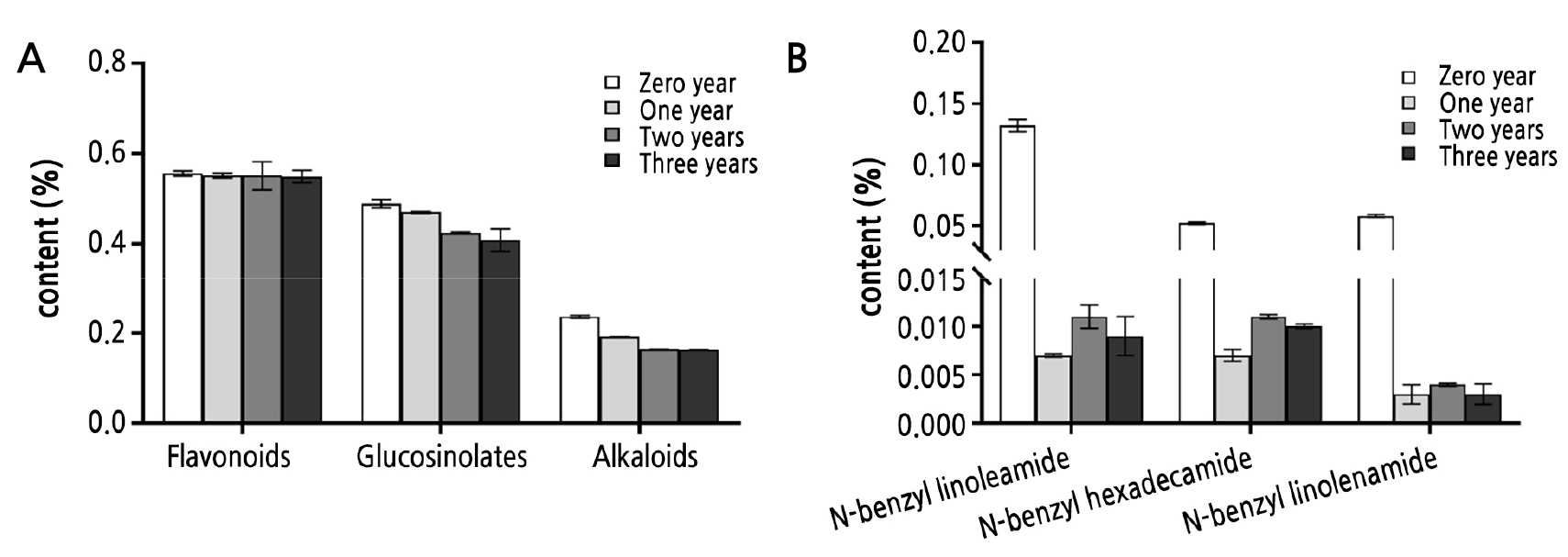
Fig. 5.
Effects of different storage periods on the contents of flavonoids, glucosinolates, alkaloids (A) and macamides (B) in black maca. The maca samples were harvested from 2019, 2018, 2017 and 2016 with storage periods of zero, one, two and three years, respectively. Vertical bars denote the standard error (n = 3).
Principal Component Analysis of Quality Indicators of Black Maca according to the Processing Method
The nutrient contents of eleven processing methods of maca were analyzed to check for correlations using SPSS 19.0. Three principal components were extracted from the nutrient content based on the principle of eigenvalues (λ)≥1. The eigenvalues were 4.106, 1.77, and 1.201, with corresponding contribution rates of 51.326%, 22.126%, and 15.01%. The cumulative contribution rate reached 88.462%, which can explain the change trends of eight nutrient components. Three principal components were also extracted from the bioactive components, with eigenvalues of 3.989, 0.869, and 0.567 and corresponding contribution rates of 66.481%, 14.475%, and 9.448%. The cumulative contribution rate reached 90.404%, which can explain the change trends of six bioactive components (Tables 6 and 7).
Table 6.
Characteristic value, contribution rate, and accumulative contribution rate of every principal component in the nutritional component of maca
Table 7.
Characteristic value, contribution rate, and accumulative contribution rate of every principal component in the bioactive component of maca
The principal component comprehensive score model was calculated by multiplying the eigenvalue and corresponding contribution rate of each principal component. The formulas for calculating the nutrient and bioactive component scores are shown below.
Nutritional composition:
F = 0.580 × F1 + 0.250 × F2 + 0.169 × F3
Bioactive ingredients:
F = 0.736 × F1 + 0.160 × F2 + 0.104 × F3
By summing up the comprehensive scores of the nutrient and bioactive components, the comprehensive total score of black maca was obtained, after which the ranking was calculated based on the total score (Table 8). The results showed that processing method 10 had the highest total score and that the processing method 5 ranked second. Both of these processing methods involve drying whole black maca, suggesting that the quality of whole black maca processing was better and should be given priority when processing at origin.
Table 8.
Eigenvalues and comprehensive evaluation of different processing methods of black maca
Discussion
This research finds that the contents of polysaccharides and starch in dried maca sliced at 8 mm at a temperature 55°C were highest, while the contents of flavonoids, alkaloids and other nutrients were relatively high. It may be that the thick slice has a smaller specific surface area such that fewer losses of active ingredients occurred, with these components being better retained, resulting in the content being higher than in a thin slice. The content of glucosinolates in 2 mm slices dried at 35°C is highest, which may be due to the rapid inactivation of myrosinase under the drying conditions at 35°C and the low degree of decomposition. Maca with a large slice thickness has a slow rate of water loss, and glucosinolates are easily blackened. One study reported that increasing the boiling temperature can increase the decomposition rate of broccoli glucosinolates. The hydrolysis of glucosinolates occurs by myrosinase to sulforaphane [(–)-1-isothiocyanato-(4R)-(methylsulfinyl)butane] (Jin et al., 1999). Glucosinolates have been shown to be thermally degraded (Macleod et al., 1981; Kraljic et al., 2018). In intact cells, glucosinolates are cleaved by the endogenous enzyme myrosinase. When cells are disrupted, myrosinase by which hydrolyses glucosinolates will be released and glucosinolates are readily degraded to isothiocyanates (Marcinkowska and Jelen, 2020; Oloyede et al., 2021). Our results show that the content of glucosinolates was higher at 35°C than at 55°C. It is speculated that the cell tissue at 35°C is not destroyed as quickly as it is at 55°C, and glucosinolates are decomposed slowly by black mustard enzyme; hence, the glucosinolate content measured at 35°C is higher than that at 55°C, suggesting that our conclusion is more significant. Under the action of mustard enzymes, benzyl glucosinolates are hydrolyzed to form benzylamine, which reacts with different free fatty acids to form different macamides (Esparza et al., 2015). In this study, the highest content of N-benzyl linoleamide was obtained by drying 8 mm slices at 55°C, and the highest contents of N-benzyl hexadecanamide and N-benzyl linoleamide were obtained by drying whole maca at 35°C. One possible reason is that the glucosinolate enzyme is activated by drying 8 mm sliced maca at 55°C, and the reaction between the glucosinolate enzyme and glucosinolates is sufficient, with the content of benzylamine being high as well. Whole dried black maca also has a slow water loss rate at 35°C; the reaction time between the glucosinolate enzyme and glucosinolates is long, and the content of benzylamine is high. Finally the content of macamides is high when benzylamine reacts with the corresponding free fatty acid.
A large number of studies show that the quality of Chinese herbal medicine is influenced by the variety. To date, there are three known varieties of maca that can be distinguished by their external color, with different properties attributed to each variety (Gonzales and Alarcón-Yaquetto, 2018). It has been reported that yellow maca produced in Yunnan has a better effect on alleviating physical fatigue than purple and black maca (Zhou et al., 2017). The contents of flavonoids, glucosinolates, macamides and alkaloids in yellow maca are higher than those in black maca, suggesting that yellow maca produced in Yunnan may have better anti-fatigue effects than black maca.
During the growth of Chinese herbal medicine, the storage content of nutrients is closely related to the harvest time. A study was conducted to analyze alfalfa at different harvest periods and storage periods to assess the dynamic changes in the VOCs and nutritional quality levels. The results indicated that the VOCs and nutrient quality of alfalfa varied significantly in different harvest periods; delayed harvesting significantly reduced a range of different nutrient levels (Yuan et al., 2022). The accumulation of five major saponins in three harvest seasons of Panax notoginseng was detected by HPLC, and it was found that September in the third year was the best harvesting time due to the highest content of these five major saponins in the roots at that time (Xia et al., 2017). The harvesting time also has a significant effect on the quality of maca (Wang et al., 2006). Research has shown that the best time to harvest maca is from mid-December to mid-January (Xiong and Liu, 2014). The accumulation of dry matter and bioactive components is insufficient during an early harvest, and the nutrients begin to transform after a late harvest. It has also been shown that the dry matter quality of maca root increases with an extension of harvesting time and that the dry matter quality of the aerial parts initially increase and then decrease (Chen et al., 2013). In our study, the contents of the main active and nutritional components of maca increased with an extension of the harvesting time. This is most likely due to sufficient light, less rain and a large temperature difference between day and night around the winter solstice. These climatic conditions are conducive to the accumulation of nutrients and bioactive components in maca.
The nutritional and pharmacodynamic components of Chinese herbal medicines also change significantly depending on the storage period. It was observed that the contents of catechins and amino acids showed a similar tendency to decrease with the storage period, while gallic acid increased with the storage period (Ning et al., 2016). Increased levels of nutritional compounds such as lipids, proteins and carbohydrates were found in seaweed stored for a longer period of time (126 days) as compared to seaweed under short-term storage (61 days) (Stévant et al., 2020). By studying the effects of olive storage times on the chemical and sensory properties of extra virgin olive oil, findings have shown that the duration of olive storage has an important effect on the quality of the final oil (Rotondi et al., 2021). It was also found that the contents of total flavonoids, polysaccharides, ursolic acid, oleanolic acid and rosmarinic acid in Prunella vulgaris gradually decreased as the number of storage years increased (Zhang et al., 2012). The present study found that the contents of bioactive components in maca, specifically macamides, flavonoids, glucosinolates, alkaloids and others, decrease gradually with longer storage periods.
Conclusions
In conclusion, different varieties, processing methods, harvesting times, and storage periods of maca have different effects on the nutritional and bioactive components, all of which influence the quality of maca. Therefore, it is recommended to dry whole maca at 35°C, harvest it around the winter solstice, and plant it at an altitude of around 3,000 meters. This is an important experimental basis for the cultivation and industrial development of maca. In addition, maca should not be stored for long periods and should be consumed as soon as possible.