Introduction
Materials and Methods
Plant Materials
Determination of ROS (O2•− and H2O2) and Lignin Levels
Cell wall-related Enzyme Activity
RNA Extraction and Reverse Transcription-Quantitative PCR
Statistical Analysis
Results
Fruit Fresh Weight During Fruit Development and Ripening
Reactive Oxygen Species in Pear Flesh During Fruit Development and Ripening
Lignin Content in Pear Flesh During Fruit Development and Ripening
Activities of Cell Wall-related Enzymes in Pear Flesh During Fruit Development and Ripening
Expression of Cell Wall Lignification-related Genes
Expression of Cell Wall Expansion-related Genes
Discussion
Conclusion
Introduction
Reactive oxygen species (ROS), such as superoxide radical (O2•–), hydrogen peroxide (H2O2), and hydroxyl radical (•OH), have long been documented as toxic byproducts produced during abiotic or biotic stress. Accumulation of ROS causes protein oxidation, RNA damage, and lipid peroxidation, consequently reducing growth upon short-term stress and causing plant cell death upon long-term stress (Hernández et al., 2001; Lee et al., 2007). Most studies have focused on the toxic effects of ROS. However, the focus has shifted recently to elucidate the function of ROS as cell signaling molecules for normal biological processes (Schmidt et al., 2016). For example, during germination and pollen elongation, ROS induces proficient germination by releasing dormancy (Singh et al., 2016; Basbouss-Serhal et al., 2017) and promoting pollen growth toward the female gametophyte (Potocký et al., 2012). Altered ROS homeostasis has also been reported to affect lateral root emergence and root hair growth by interacting with auxin signaling (Mangano et al., 2017). Huan et al. (2016) reported that the production of ROS during the early expansion stages was closely related to the quick development of fruit, suggesting that ROS might be involved in regulation of fruit cell expansion.
Among the major ROS, O2•– in apoplast is produced by electron transferred from plasma membrane-bound NADPH oxidase and rapidly converted to H2O2 by superoxide dismutase (SOD) (Waszczak et al., 2015). H2O2 supplies electrons to phenolic substrates (coumaric, coniferyl, and sinapyl alcohols), which get polymerized by peroxidases (POXs) in the cell wall, resulting in the cross-linking of cell wall components such as lignin and suberin (Lee et al., 2007; Schmidt et al., 2016). It has been reported that five of 41 POX genes in Chinese pear (Pyrusbretschneideri) are significantly correlated to lignin content during fruit development (Cao et al., 2016). In addition, a POX gene FaPOD27, which is a key gene for lignin biosynthesis, is primarily expressed in green and immature strawberry fruit (Yeh et al., 2014).
On the other hand, H2O2 gets converted into •OH by the Fenton reaction or Haber–Weiss reaction, which breaks covalent bonds in cell wall polymers, xyloglucan and pectin, leading to cell loosening and cell elongation, consequently cell expansion (Müller et al., 2009; Francoz et al., 2015; Richards et al., 2015). Cell expansion is mainly regulated by the cell wall-degrading enzymes such as xyloglucan endotransglucosylases (XETs), xyloglucan endotransglucosylases/ hydrolases (XTHs), and expansins (EXPs). Catalá et al. (2000) reported that expression levels of LeEXPs are the highest at the fruit expansion stage and then decrease at the ripening stage in tomato. Positive correlations between XTH accumulation and ripening were observed in apple (Atkinson et al., 2009) and tomato (Miedes and Lorences, 2009). Therefore, cell wall expansion, which is regulated by the balance between cell wall stiffening and loosening, is closely related to ROS homeostasis (Wolf et al., 2012; Francoz et al., 2015).
In Korea, the Asian pear (Pyrus pyrifolia Nakai), popular for its sweetness and juiciness, is abundantly produced. The most common cultivar in Korea, ‘Niitaka’, is a middle-late-maturing cultivar and characterized by large fruit size and slow ripening (Pasquariello et al., 2013). Some areas cultivate the early-maturing cultivars, ‘Wonhwang’ and ‘Whangkeumbae’, which are characterized by a small fruit size and fast ripening (Yoo et al., 2002). Among the pear cultivars, fruit firmness decreases more rapidly in the early-maturing cultivars than in the late-maturing type, during the early stage of fruit development (Oanh et al., 2012). The decrease in fruit firmness is associated with a decrease in the polysaccharide content of the cell wall, resulting in rapid cell wall expansion due to cell loosening (Richards et al., 2015; Yoo et al., 2020). In our previous study, the absolute fruit growth rate was higher in the early-maturing cultivars ‘Wonhwang’ and ‘Whangkeumbae’ than in the late-maturing cultivar ‘Niitaka’ during the early stage of fruit development (Lee et al., 2021). This indicated that cell wall expansion occurs earlier in early-maturing cultivars than in late-maturing cultivars. Although cell wall expansion is known to occur differently depending on the fruit maturity period, the role of ROS-mediated signaling in regulating cell wall expansion has not been fully explored yet.
In the present study, we investigated how the modulation of ROS homeostasis by the action of POX affects cell wall expansion in two Asian pear (Pyrus pyrifolia Nakai) cultivars with different maturity periods. To address this question, we measured levels of ROS, lignin content, POX activity, and cell wall-related gene expression levels during pear fruit development and ripening.
Materials and Methods
Plant Materials
Two cultivars of Asian pear (Pyrus pyrifolia Nakai), ‘Wonhwang’ (early-maturing) and ‘Niitaka’ (late-maturing), were harvested from an orchard in the Naju province in South Korea. Pear fruits were harvested 57 to 177 days after full bloom (DAFB), at 15-day intervals. On each sampling date, three replicates (at least five fruits in each replicate from three trees) of fruit samples, were harvested and immediately measured for weight. After removing the core and peel, the fruits were cut into small pieces, frozen in liquid nitrogen, and stored in a deep freezer (–80°C) until further use. The fruit fresh weight was previously published by Lee et al. (2021).
Determination of ROS (O2•− and H2O2) and Lignin Levels
The levels of ROS [superoxide anion radical (O2•–) and hydrogen peroxide (H2O2)] and lignin were determined according to the spectroscopic methods described by Lee et al. (2009) and Lee et al. (2007), respectively.
Cell wall-related Enzyme Activity
Fresh samples (0.5 g) were mixed with 100 mM potassium phosphate buffer solution (pH 5.8) containing 2 mM phenylmethylsulphonyl fluoride, and centrifuged at 14, 000 × g at 4°C for 20 min. The obtained pellet was washed four times with 50 mM sodium phosphate (pH 5.8) and incubated in 0.1 M sodium acetate buffer (pH 5.0) containing 0.5% cellulase and 2.5% pectinase for 24 h. The resulting supernatant contained the cell wall fraction. SOD activity was detected using an EZ-SOD assay kit (Dogen, DG-SOD400). The activities of guaiacol peroxidase (GPOX) and coniferyl alcohol peroxidase (CPOX) were determined by following the method described by Lee et al. (2007).
RNA Extraction and Reverse Transcription-Quantitative PCR
Total RNA was isolated from 100 mg fresh flesh of fruit using cetyltrimethylammonium bromide (CTAB) buffer containing 20 mM dithiothreitol. The cDNA was synthesized using the GoScript Reverse Transcription System (Takara). Quantitative PCR (qPCR) was conducted Quantitative PCR (qPCR) was conducted on a Bio-Rad CFX96 qPCR System using the TB Green Premix Ex Tag (Takara). Gene-specific primers used for qPCR are presented in Table 1. All reactions were run in duplicates for each of the three independent samples. All the quantifications were normalized to ubiquitin.
Table 1.
Specific primers used for RT-qPCR
Statistical Analysis
The data were subjected to Duncan’s multiple range test using SAS 9.4 (SAS Institute Inc., Cary, NC, USA), and differences at p < 0.05 were considered statistically significant.
Results
Fruit Fresh Weight During Fruit Development and Ripening
The fresh weight started to increase after 72 and 87 DAFB in ‘Wonhwang’ and ‘Niitaka’, respectively, and then increased steadily until the end of fruit harvest (Fig. 1). Fresh weight was higher in ‘Wonhwang’ than in ‘Niitaka’ before 147 DAFB; this trend reversed post 147 DAFB. A rapid fruit growth rate was observed between 102 and 117 DAFB in ‘Wonhwang’ and between 132 and 147 DAFB in ‘Niitaka’.
Reactive Oxygen Species in Pear Flesh During Fruit Development and Ripening
Superoxide (O2•–) levels in the two pear cultivars rapidly decreased to 102 DAFB and its decrease rate was higher in ‘Niitaka’ than in ‘Wonhwang’. No significant difference between the two pear cultivars was observed between 102 and 147 DAB (Fig. 2A). On the other hand, hydrogen peroxide (H2O2) content in ‘Wonhwang’ sharply decreased from 57 DAFB (at the beginning of sampling) to 72 DAFB; the decrease became gradual during fruit development and ripening. In ‘Niitaka’, H2O2 content was maintained during the early stage of fruit development and ripening but it was significantly reduced from 87 to 117 DAFB (Fig. 2B).
Lignin Content in Pear Flesh During Fruit Development and Ripening
Lignin content was the highest at the beginning of sampling, regardless of the cultivar. Lignin content decreased substantially in ‘Wonhwang’ until 102 DAFB, whereas it was maintained at a level comparable to that at the beginning of sampling until 87 DAFB and then continuously decreased until 132 DAFB in ‘Niitaka’ (Fig. 3).
Activities of Cell Wall-related Enzymes in Pear Flesh During Fruit Development and Ripening
SOD activity in both pear cultivars was the highest at the beginning of sampling (57 DAFB), with higher levels in ‘Niitaka’ than in ‘Wonhwang’. SOD activity in ‘Wonhwang’ gradually decreased from 57 DAFB, whereas its activity in Niitaka started to decrease after 87 DAFB (Fig. 4A). GPOX activity in ‘Wonhwang’ continuously decreased from 57 to 177 DAFB. In ‘Nittaka’, however, GPOX activity was maintained until 87 DAFB and then gradually decreased to 177 DAFB but was still higher than that in ‘Wonhwang’ (Fig. 4B). Like GPOX, CPOX activity in ‘Niitaka’ was maintained at a high level until 87 DAFB and then rapidly decreased until 132 DAFB. On the other hand, in ‘Wonhwang’, CPOX activity decreased continuously after 72 DAFB (Fig. 4C).
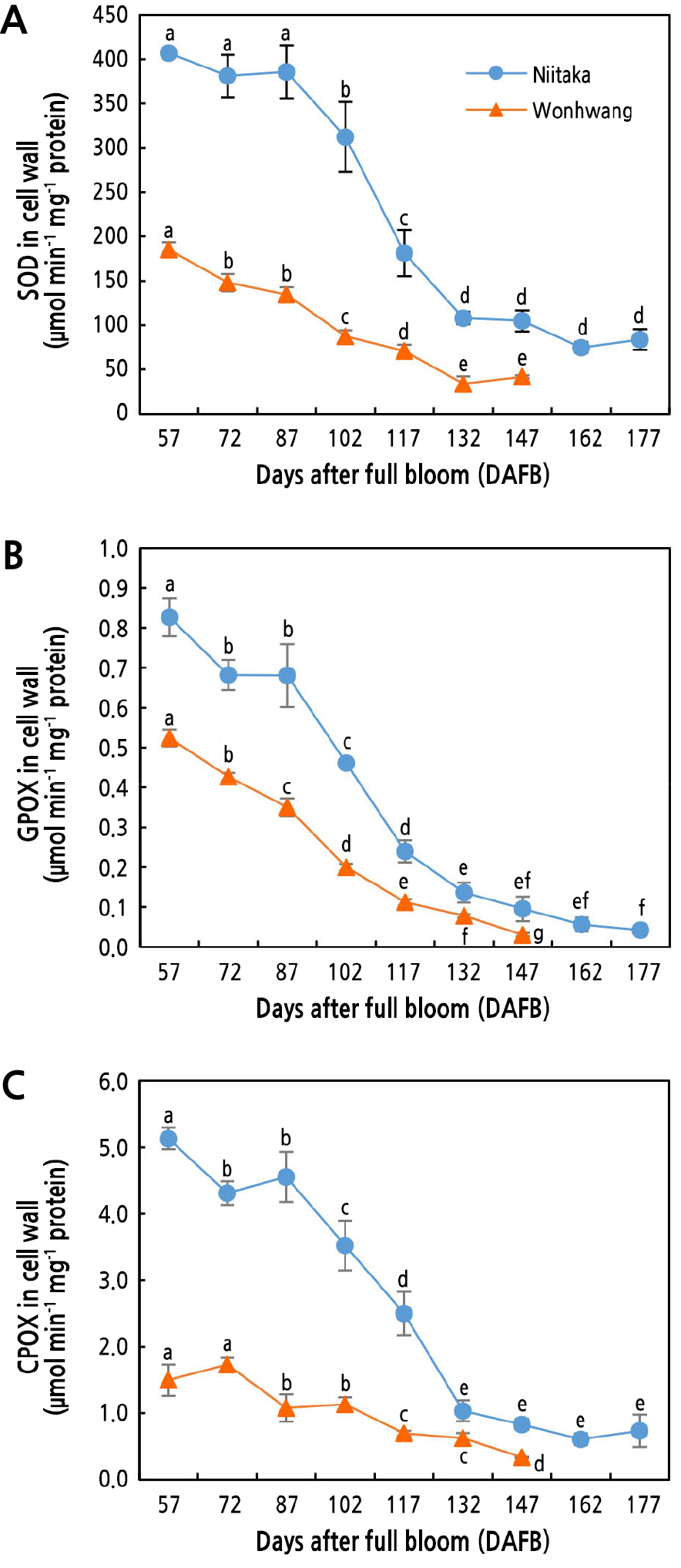
Fig. 4.
Changes in activities of SOD (A), GPOX (B), and CPOX (C) enzymes in Asian pear ‘Wonhwang’ and ‘Niitaka’ during fruit development and ripening. SOD, superoxide dismutase; GPOX, guaiacol peroxidase; CPOX, coniferyl alcohol peroxidase. Data are expressed as mean ± SD (n = 3). Letters indicate statistically significant differences between stages of fruit development in each cultivar (p < 0.05).
Expression of Cell Wall Lignification-related Genes
The expression levels of four cell wall-related genes—PpPOX1, PpPOX2, PpPOX3, and PpPOX4—were observed in the two pear cultivars during fruit development and ripening (Fig. 5). The expression level of PpPOX1 decreased continuously from 57 to 102 DAFB in ‘Wonhwang’, whereas it significantly increased from 57 to 102 DAFB and then rapidly decreased to 117 DAFB in ‘Niitaka’ (Fig. 5A and 5E). The expression level of PpPOX2 significantly decreased after 57 and 72 DAFB in ‘Niitaka’ and ‘Wonhwang’, respectively (Fig. 5B and 5F). PpPOX3 expression decreased in both cultivars after 57 DAFB (Fig. 5C and 5G). The expression of PpPOX4 was high until 72 DAFB in ‘Wonhwang’ and increased between 57 and 87 DAFB in ‘Niitaka’, but then decreased until the end of sampling in both cultivars (Fig. 5D and 5H).
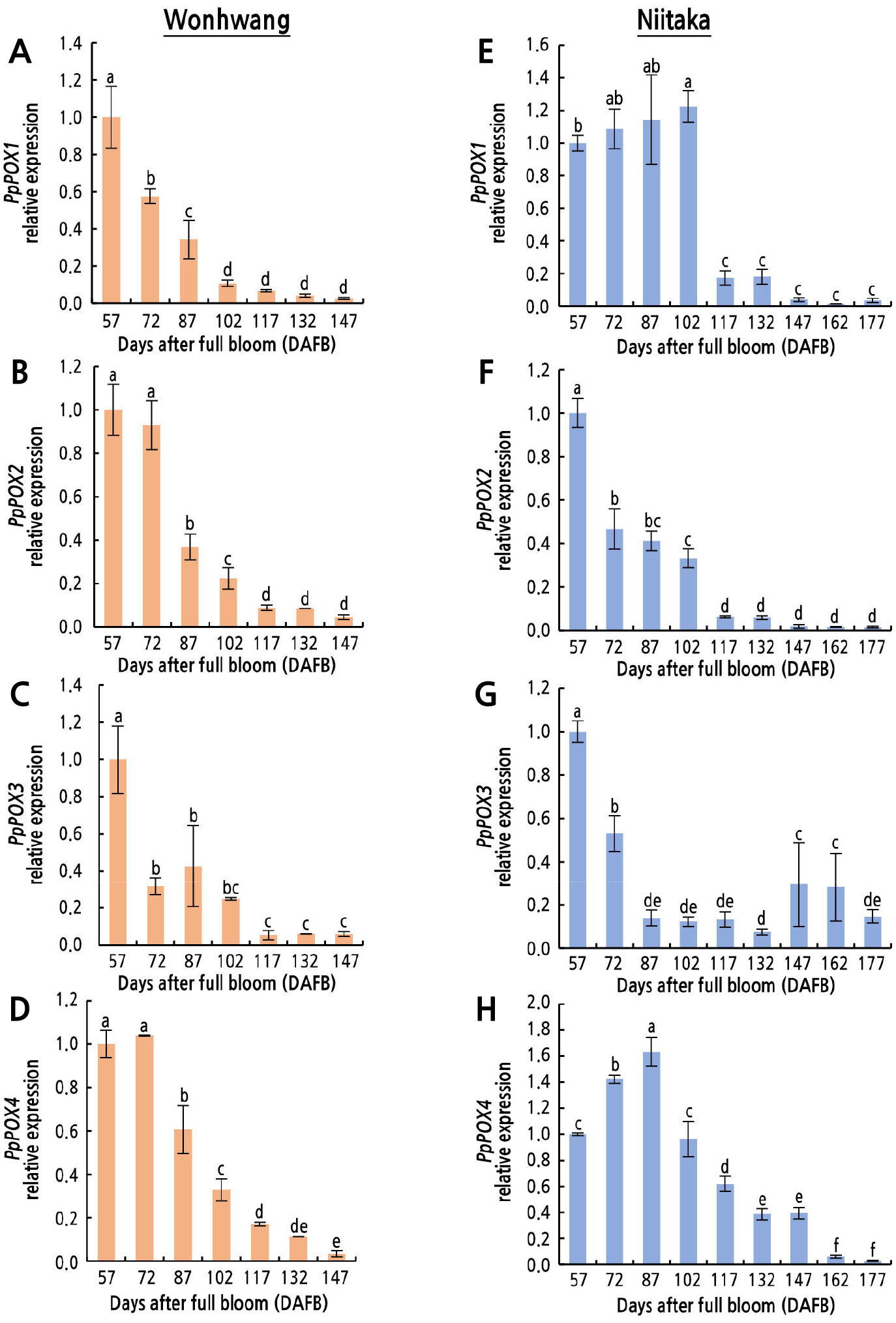
Fig. 5.
Relative expression levels of peroxidase genes in Asian pear ‘Wonhwang’ and ‘Niitaka’ during fruit development and ripening. PpPOX1 (A, E), PpPOX2 (B, F), PpPOX3 (C, G), and PpPOX4 (D, H). Data are expressed as mean ± SD (n = 3). The levels at 57 DAFB in each cultivar are set to 1. Letters indicate statistically significant differences between stages of fruit development in each cultivar (p < 0.05).
Expression of Cell Wall Expansion-related Genes
The expression patterns of the cell wall expansion-related genes PpEXP1 and PpXET1 differed during fruit development and ripening (Fig. 6). The expression of PpEXP1 was the highest between 102 and 117 DAFB in ‘Wonhwang’ and between 102 and 132 DAFB in ‘Niitaka’ (Fig. 6A and 6B). The expression of PpXET1 in ‘Wonhwang’ gradually increased from 57 to 132 DAFB, when it was 2.4-fold higher than that at 57 DAFB (Fig. 6C). In ‘Niitaka’, the expression of PpXET1 was 1.7-fold higher at 102 DAFB than at 57 DAFB, and it was 5.0-fold higher at 177 DAFB than at 57 DAFB (Fig. 6D).
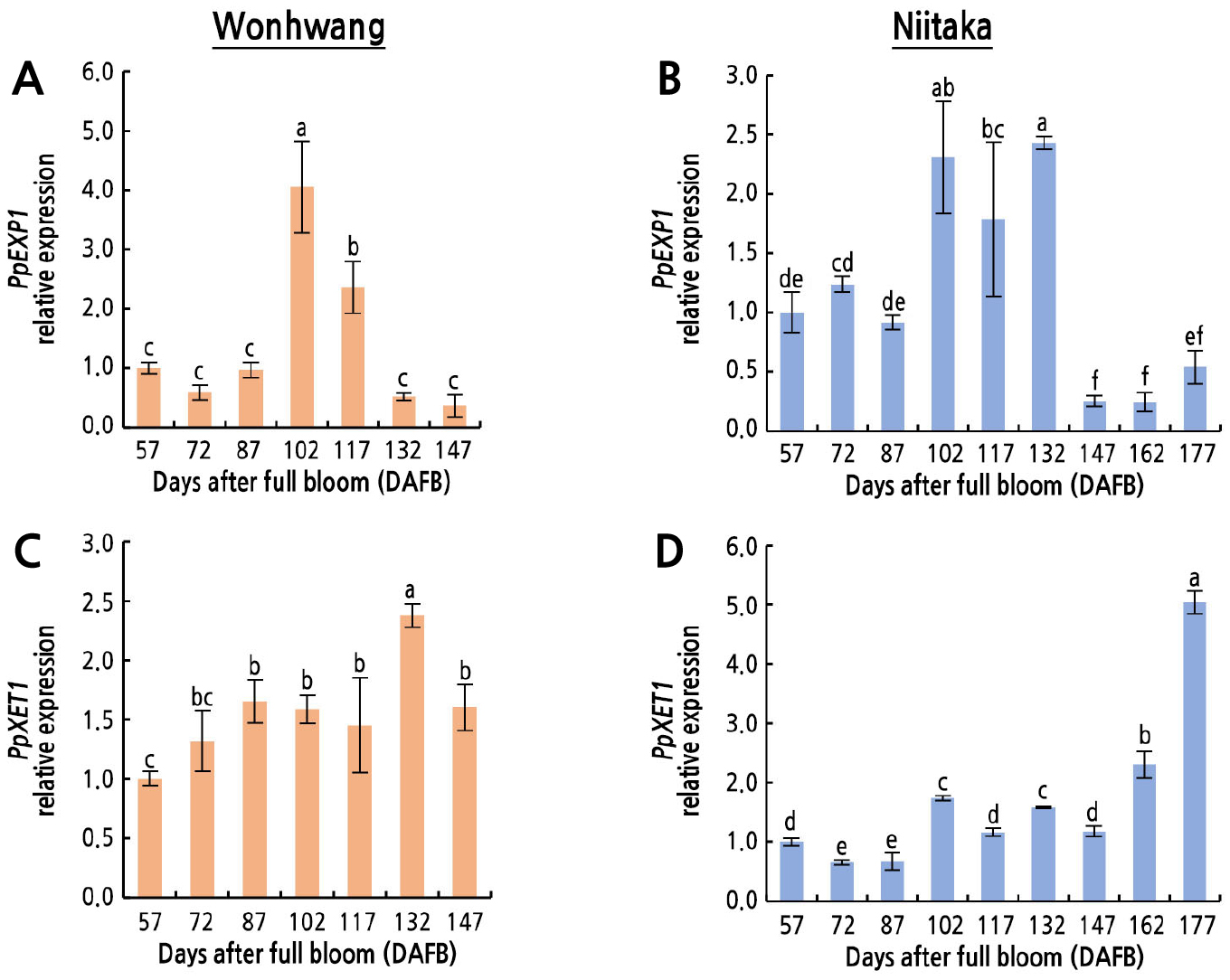
Fig. 6.
Relative expression levels of cell wall expansion-related genes in Asian pear ‘Wonhwang’ and ‘Niitaka’ during fruit development and ripening. Expansin 1 (PpEXP1) (A, B), and xyloglucan endotransglucosylases 1 (PpXET1) (C, D). Data are expressed as mean ± SD (n = 3). The levels at 57 DAFB in each cultivar are set to 1. Letters indicate statistically significant differences between stages of fruit development in each cultivar (p < 0.05).
Discussion
As expected, fruit fresh weight was higher in the late-maturing cultivar ‘Niitaka’ than in the early-maturing cultivar ‘Wonhwang’. A large increase in fruit weight was observed from 72 to 117 DAFB for ‘Wonhwang’ and from 102 to 147 DAFB for ‘Niitaka’ (Fig. 1), indicating that rapid cell wall expansion occurred during these periods. Cell wall expansion is regulated by a balance between cell wall stiffening and loosening, which is associated with ROS homeostasis (Wolf et al., 2012; Francoz et al., 2015). In the present study, a higher level of ROS (O2•– and H2O2) was observed during the early stage of pear fruit development than during the later stage (Fig. 2A and 2B). This was accompanied by a high SOD activity (Fig. 4A), which accelerates the dismutation of O2•– into H2O2 and oxygen. During the early stage, a lower level of O2•– was observed in ‘Wonhwang’ compared to in ‘Niitaka’ (Fig. 2A). The low O2•– content may be due to the production of •OH, which catalyzes the oxidative cleavage of xyloglucan and pectin leading to cell wall loosening, consequently promoting cell elongation (Müller et al., 2009). These results are consistent with the rapid fruit growth rate in ‘Wonhwang’ during the early stage of fruit growth and development (Fig. 1). During this stage, a high-SOD-induced H2O2 accumulation was observed in ‘Niitaka’ (Fig. 2B). H2O2 is a signaling molecule, and its accumulation can cause cell wall loosening by producing •OH through the Haber–Weiss reaction (Müller et al., 2009) or cell wall stiffening by cross-linking of cell wall polymers (Cona et al., 2006; Dunand et al., 2007; Richards et al., 2015). A high level of H2O2 content in ‘Niitaka’ was maintained until 87 DAFB; concomitantly, fruit growth rate was maintained at the same level during the initial harvest (57 DAFB) (Figs. 1 and 2B). These results indicate that, in the late-maturing cultivar ‘Niitaka’, H2O2 accumulation during the early stage of fruit growth and development results in cell wall stiffening, thereby reducing the elongation capacity of cell walls.
H2O2-mediated cell wall cross-linking is catalyzed by POXs (Cona et al., 2006), which oxidize monolignols in the cell wall to form radicals, consequently resulting in the production of polymerized lignin (Lee et al., 2007; Francoz et al., 2015). Indeed, a significantly higher level of GPOX and CPOX activities was observed at the early stage of fruit growth and development than at the later stage; the high POX activity was accompanied by a high lignin content (Figs. 3, 4B and 4C). Lee et al. (2007) reported that an increase in GPOX, CPOX, and synaphyl alcohol peroxidase (SPOX) activity can cause lignification and rigidification of cell walls. Lignin content was maintained for longer and at a higher level in ‘Niitaka’ than in ‘Wonhwang’ during the early stage of fruit growth and development (Fig. 3), resulting in a slower fruit growth rate in ‘Niitaka’ compared to in ‘Wonhwang’ (Fig. 1). Therefore, lignin accumulation-induced cell wall stiffening is closely related to restriction of cell expansion (Dunand et al., 2007; Richards et al., 2015). However, lignin content continuously decreased with fruit maturation (Fig. 3), as reported by Mamat et al. (2019) and Zhang et al. (2021), indicating that lignin biosynthesis mainly occurs during the early stages of fruit development. Recently, it has been reported that the lignin content in pear fruit flesh is closely related to the accumulation of H2O2 and stone cells, which gradually decrease as fruit develop (Wang et al., 2021). Lignin content is also closely correlated with fruit firmness, which decreases as fruit matured (Cai et al., 2006). For example, in strawberry, an increase in lignin biosynthesis due to POX accumulation leads to improved firmness (Yeh et al., 2014), delaying fruit ripening. There is an inverse correlation between cell growth and POX activity (Lee et al., 2007). Previous studies have reported that the activities of several POX isoforms (44, 48, and 53 kDa) were detected in the exocarp of tomato fruits from the mature-green to red-ripe stage and were associated with cessation of fruit growth by stiffening of the walls rather than fruit ripening or softening (Andrews et al., 2000). In addition, different POX genes from tomato were identified in immature fruits (LePrx35 and LePrxA) and in both immature and mature green fruits (LePrx17 and LePrx09) (Wang et al., 2015). In the present study, the expression levels of PpPOX1, PpPOX2, PpPOX3, and PpPOX4 decreased as fruit matured, but their expression patterns differed depending on the fruit cultivar (Fig. 5). PpPOX1 and PpPOX4 expression levels decreased after 57 and 72 DAFB, respectively, in ‘Wonhwang’, whereas they decreased after 102 and 87 DAFB, respectively, in ‘Niitaka’ (Fig. 5). The expression trends of these two genes were consistent with the changes in lignin content during fruit development (Fig. 3). Similarly, Cao et al. (2016) found that the expression of five PbPRXs genes (PbPRX2, PbPRX22, PbPRX34, PbPRX64, and PbPRX75) related to the lignin synthesis pathway in Chinese pear (Prus bretschneideri) increased during the early stage of fruit development (until 63 DAFB) and then decreased during pear fruit ripening (until 145 DAFB). These results reveal that H2O2-dependent POX is responsible for cell wall lignification.
Given that POX prompts the formation of diferuloyl bridges between pectin residues and isodityrosine bridges between hydroxyprotein-rich extension molecules, a decrease in POX activity is associated with degradation of polysaccharide in the cell wall during fruit maturation, which indicates that cell wall expansion occurs due to cell loosening (Andrews et al., 2000). Additionally, •OH produced by H2O2 decomposition through the Fenton reaction or Haber–Weiss reaction accelerates cell elongation due to cell wall loosening by oxidative cleavage of xyloglucan and pectin (Müller et al., 2009). This cell wall loosening is regulated by two major proteins, EXP and XET, which cleave the bonds in xyloglucans, hemicellulose, and cellulose (Cosgrove, 2005; Park and Cosgrove, 2012), consequently allowing cells to expand. In several fruits, XTH-coding genes are highly expressed in young and mature fruits (Miedes et al., 2010; Opazo et al., 2017). Dramatic changes in PpEXP1 gene expression were observed between 87 and 117 DAFB in ‘Wonhwang’ and between 87 and 132 DAFB in ‘Niitaka’ (Fig. 6A and 6B), resulting in a longer period of cell expansion in ‘Niitaka’ (45 days) than in ‘Wonhwang’ (30 days). These results indicate that cell wall loosening due to an increase in EXP only presents during the middle stage of fruit development and ripening, which is consistent with the highest absolute fruit growth rate in ‘Wonhwang’ but not in ‘Niitaka’, as shown previously (Lee et al., 2021). Similarly, in pepper, expression levels of CaEXPA14 and CaEXPA19 genes during the early stage of fruit growth were positively related to the relative rate of cell expansion (Mayorga-Gómez and Nambeesan, 2020). Additionally, Lu et al. (2016) identified four expansin genes (SIEXPA8, SIEXPB8, SIEXLB1, and SIEXLB2) in tomato that regulated the cell division and enlargement stages. Here, PpXET1 gene expression level began to increase after 57 or 87 DAFB in ‘Wonhwang’ and ‘Niitaka’, respectively, and increased until the fruits ripened, indicating that PpXET1 is related to cell wall expansion throughout fruit development and ripening (Fig. 6C and 6D). These results indicate that cell wall expansion in ‘Wonhwang’ occurs faster than that in ‘Niitaka’, which is consistent with the lower lignin content and lignin synthesis-related enzyme (CPOX and GPOX) activity.
Conclusion
Cell wall expansion associated with an increase in fruit fresh weight occurred earlier in ‘Wonhwang’ than in ‘Niitaka’, which was consistent with the higher expression of the cell wall loosening-related genes, PpEXP1 and PpXET1, during fruit growth and development. Cell wall expansion was closely related to altered ROS (O2•– and H2O2) content. The higher ROS content in ‘Niitaka’ than in ‘Wonhwang’ enabled lignin biosynthesis by activating POX enzyme activity and gene expression during the early stage of fruit growth and development. This resulted in a delay in cell wall expansion, which is associated with low expression levels of PpEXP1 and PpXET1. Therefore, these results indicate that ROS-mediated lignin synthesis through POX activity is associated with cell wall expansion in Pyrus pyrifolia. These results will be useful for regulating of fruit size through modification of H2O2 content during the early stage of fruit development.