Introduction
Materials and Methods
Experimental Material
Hydropriming and Light Quality Pretreatments
Cabbage Seed Germination and Sprout Cultivation
Extraction and Concentration of Bioactive Compounds
Total Flavonoid and Total Polyphenol Content
Free Radical Scavenging Activity
Statistical Analysis
Results and Discussion
Germination and Growth of Cabbage Seeds Pretreated with Hydropriming and Light Quality
Effect of the Extraction Solvent on Antioxidant Content and Free Radical Scavenging Activity of Cabbage Sprouts
Antioxidant Content and Free Radical Scavenging Activity of Pretreated Cabbage Sprouts
Conclusions
Introduction
Cabbage (Brassica oleracea var. capitate) is mainly consumed as a leafy vegetable and is rich in health-promoting bioactive compounds such as fiber, vitamins, glucosinolates, polyphenols, and flavonoids (Nawaz et al., 2018; Novotny et al., 2018; Haghighi et al., 2020). Flavonoids have anti-tumor, anti-aging, and anti-inflammatory properties as well as oxidative prevention against diseases induced by oxygen free radicals through free radical-mediated reactions of biomolecules such as nucleic acids, polyunsaturated lipids, and sugars (Xiao et al., 2016). Antioxidants protect against protein damage at low concentrations; vitamin C and polyphenols, the major antioxidants, protect cells from oxidative damage by directly scavenging free radicals (Podsędek, 2007).
Sprouts are the first stage of seed germination. Seeds store all the vitamins, minerals, proteins, fats, and carbohydrates needed for plant growth. Sprouts contain a large amount of bioactive compounds, such as minerals, amino acids, vitamins, and flavonoids, compared to mature plants (Kim et al., 2004; Xu et al., 2020). Sprouts are good sources of dietary antioxidants (Bendary et al., 2013). The abundance of bioactive compounds in sprouts is greatly influenced by seed quality and developmental stage (Nam et al., 2018).
Seed priming involves hydration and drying of the seed to improve germination and induces biochemical and physiological changes in the seed that influence the antioxidant and metabolic activity via synthesis of proteins and nucleic acids (RNA and DNA) (Farooq et al., 2010; Di Girolamo and Barbanti, 2012). Seed priming methods include hydropriming, halopriming, osmopriming, solid matrix priming, biopriming, nutripriming, and priming with hormones and plant growth regulators (Sher et al., 2019). Hydropriming is a simple, low-cost technique that activates metabolic processes by soaking seeds in water in the dark for a certain period of time and maintains an appropriate amount of moisture after drying (Waqas et al., 2019).
Light is not only the source of energy for photosynthesis but also a signal for multiple physiological responses (Zhang et al., 2020). In particular, ultraviolet (UV), infrared, and visible light have a significant impact on flavonoid production during germination (Tsurunaga et al., 2013). The beneficial effects of various light quality treatments, such as an increase in plant growth, the production of bioactive compounds (carotenoids, vitamin C, and tocopherol), and antioxidant activity, have been reported (Wu et al., 2007; Ciska et al., 2008; Pérez-Balibrea et al., 2008; Peng et al., 2015; Qian et al., 2016; Samuolienė et al., 2017; Choi et al., 2022).
This study was performed to compare the growth, bioactive compounds, and antioxidant activities of sprouts from cabbage seeds pretreated with hydropriming and different light quality conditions.
Materials and Methods
Experimental Material
The experiments were carried out on cabbage seeds (Brassica oleracea var. capitate cv. Tarano) harvested during 2020 from JOEUN Seeds Co., Ltd. (Goesan, Korea). The seeds were stored at 4°C until sprout cultivation.
Hydropriming and Light Quality Pretreatments
To determine the effect of hydropriming under different light quality treatments, the seeds were placed in a conical tube (SPL Life Sciences Co., Ltd., Pocheon, Korea), soaked in distilled water, and primed for 24 h in a growth chamber (20 ± 1°C) (LBI-250E, Daihan Labtech Co., Ltd., Namyangju, Korea) under dark conditions or various light qualities (Fig. 1) [100 µmol·m-2·s-1 PPFD, white LED (HT100-5700), red LED (660 nm, HT080-Red), green LED (520 nm, HT080-Green), or blue LED (450 nm, HT080-Blue) manufactured by BISSOL LED, Seoul, Korea]. After that, the seeds were dried for 24 h in a dark room controlled at 25 ± 2°C and used for sprout cultivation. Control seeds were soaked in distilled water for 24 h and cultivated without drying.
Cabbage Seed Germination and Sprout Cultivation
Seeds treated with hydropriming in each light quality were sown in three repetitions of 100 seeds each, with filter paper (Whatman, Piscataway, NJ, USA) on the sprout trays (32 × 22 × 14 cm). After culturing the seeds for 7 d in a culture room under controlled light conditions (white LED, 16/8 h light/dark cycle) and temperature of 25 ± 2°C, the final germination (%) was measured, and germinated seedlings were classified into normal and abnormal seedlings according to ISTA (2018) rules. The seedlings were considered normal if two green cotyledons developed after radicle protrusion, and the hypocotyl was straight. Seedlings were considered abnormal when the primary root did not protrude from the seed coat, cotyledons developed prior to the root, or if necrosis occurred due to deformed, broken, damaged, or discolored cotyledons. Then, 20 uniform normal seedlings were selected and their fresh weights and shoot lengths were measured. All treatments had three replicates. Cabbage sprouts from each treatment were harvested and used to compare the content of bioactive compounds and antioxidant effects.
Extraction and Concentration of Bioactive Compounds
To determine the best bioactive compound extraction solvent for cabbage sprouts, distilled water, 80% ethanol and 100% ethanol were used. A sprout sample that was hydroprimed under dark conditions was harvested, freeze-dried, and ground was used for the extraction solvent experiment. The sprout sample (1 g) was soaked in 50 mL of solvent and ultrasonically extracted for 30 min using an ultrasonic cleaner (Power Sonic 510, Emerson Electric Co., Ferguson, USA). Each extract was filtered using a vacuum filtration pump (N810, KNF Neuberger, Inc., Freiburg, Germany) and filter paper. It was then concentrated using a rotary evaporator concentrator (Eyela N-1000, Tokyo Rikakikai Co., Tokyo, Japan). After concentration, moisture was removed in a desiccator, the dry weight was obtained, and the yield of the extract was calculated using the dry weight. The yield from each extraction solvent was measured, i.e., in distilled water (386.2 ± 1.86 mg·g-1 dry weight), 80% ethanol (318.5 ± 3.09 mg·g-1 dry weight), and 100% ethanol (240.3 ± 7.78 mg·g-1 dry weight). The appropriate extraction solvent was selected by comparing the bioactive compounds and antioxidant activity of each extract. As a result, 80% ethanol was used as the extraction solvent for the sprout samples harvested from seeds pretreated with hydropriming under different light quality conditions.
Total Flavonoid and Total Polyphenol Content
Total flavonoid content (TFC) was measured following the diethylene glycol colorimetric method (Gragaham, 1992). Diethylene glycol (2 mL) and sodium hydroxide solution (200 µL of 1N NaOH) were mixed with 200 µL of each extract adjusted to a concentration of 0.5 mg·mL-1 and reacted in a constant temperature water bath (BW3-10G, JEIO Tech. Co., Ltd., Daejeon, Korea) at 37 ± 2°C for 1 h. The absorbance of the reactant was measured at 420 nm using a UV/visible spectrophotometer (Libra S22, Biochrom Ltd., Cambridge, UK). The TFC was calculated from a standard curve prepared using naringin and expressed as mg of Naringin Equivalents (NE) per g.
Total polyphenol content (TPC) was measured using the modified Folin-Ciocalteu method (Velioglu et al., 1998). After diluting each sprout sample extract to 0.5 mg·mL-1, 100 µL of each extract was added to 2 mL of 2% sodium carbonate solution. After 3 min, 100 µL of 50% Folin-Ciocalteu’s reagent was added and allowed to react for 30 min, and the absorbance of the reactant was measured at 750 nm using a UV/visible spectrophotometer. The TPC of the extract was calculated from a standard curve prepared using tannic acid and expressed as mg of Tannic Acid Equivalents (TAE) per g.
Free Radical Scavenging Activity
The ABTS+ radical scavenging ability was measured by modifying the method described by Re et al. (1999). ABTS (2,2'-azino-bis, 7.4 mM) was mixed with 2.6 mM potassium peroxydisulfate and allowed to react in the dark for 24 h. The ABTS solution was diluted so that the absorbance value at 732 nm was 0.70 ± 0.03. After mixing 50 µL of sprout sample extract diluted 100, 200, 400, and 800 times with 950 µL of ABTS solution, it was kept in the dark for 10 min to react, and the absorbance was measured at 732 nm using the UV/Vis-spectrophotometer. The ABTS radical scavenging activity was calculated using the following formula:
Scavenging activity (%) = [(Control OD – Sample OD) / Control OD] × 100.
The DPPH radical scavenging activity was determined by modifying the method described by Blois (1958). Using the reducing property of DPPH, 800 µL of DPPH (1,1-diphenyl-2-picrylhydrazyl) was added to 200 µL of the sprout sample extract diluted 100, 200, 400, and 800 times. After keeping the mixed solution in the dark for 30 min, the absorbance was measured with the UV/Vis spectrophotometer at 517 nm. DPPH radical scavenging activity was calculated in the same way as the ABTS radical scavenging activity.
Statistical Analysis
The mean and standard errors were calculated for all measured values investigated in the experiments, and the analysis of variance was performed using the SAS software (version 9.4, SAS Institute Inc., Cary, NC, USA), and the significance was tested at the level of 0.05, using the Duncan’s multiple test method.
Results and Discussion
Germination and Growth of Cabbage Seeds Pretreated with Hydropriming and Light Quality
We investigated the effects of hydropriming and light quality pretreatment on cabbage seed germination 7 d after sowing. Hydropriming pretreatment under the individual light wavelengths resulted in a final germination of 82.3–95.7%, and there was no significant difference in the effect of light quality on the germination rate when compared to the control except for the blue light, compared to the control (Table 1). The number of normal seedlings ranged from 54.3–73.5 out of 100 in all treatments. In this experiment, the harvest time of sprouts was on day 7; therefore, the low proportion of normal seedlings was due to an insufficient growth period as the 10th day of growth is the recommended evaluation time for the final germination rate of cabbage seeds as specified in the ISTA (2018) rules.
Table 1.
Growth of Brassica oleracea var. capitate sprouts influenced by the hydropriming and light quality treatments
Treatmentz |
Germination (%) | Normal seedlingsy (ea) | Abnormal seedlingsx (ea) |
Fresh weight (g) |
Shoot length (cm) |
Control | 92.5 ± 1.77wav | 73.5 ± 3.18 a | 19.0 ± 1.14 a | 0.30 ± 0.01 c | 1.44 ± 0.01 d |
HD | 95.7 ± 4.82 a | 66.3 ± 4.82 ab | 29.3 ± 5.09 a | 0.58 ± 0.03 a | 3.37 ± 0.01 a |
HW | 91.0 ± 2.05 a | 66.3 ± 1.52 ab | 24.7 ± 0.54 a | 0.53 ± 0.03 ab | 3.28 ± 0.04 a |
HR | 95.3 ± 1.52 a | 58.3 ± 6.08 ab | 37.0 ± 7.59 a | 0.46 ± 0.03 b | 2.79 ± 0.08 c |
HG | 91.0 ± 0.47 a | 54.3 ± 3.84 b | 36.7 ± 3.93 a | 0.51 ± 0.01 ab | 2.97 ± 0.03 b |
HB | 82.3 ± 3.57 b | 56.0 ± 1.41 b | 28.0 ± 2.94 a | 0.56 ± 0.02 ab | 3.31 ± 0.03 a |
zControl, soaked in water for 24 h without drying; HB, hydro-primed with blue light; HD, hydro-primed under dark; HG, hydro-primed with green light; HR, hydro-primed with red light; HW, hydro-primed with white light.
y,xRefer to ISTA (2018).
The fresh weight and shoot length of hydro-primed sprouts increased by 1.5–1.9 and 1.9–2.3 times, respectively, compared to the control. Similarly, hydropriming in Momordica charantia (Adhikari et al., 2021) increased shoot length by more than 1.6 times compared to the control, and shoot length in Cuminum cyminum (Neamatollahi et al., 2009) increased 1.2 times. Hydropriming stimulates a series of biochemical changes in the seed that are essential for germination like dormancy breaking, hydrolysis, catabolism of growth inhibitors, imbibition, and enzyme activation (Ajouri et al., 2004; Pukacka and Rajajczak, 2005; Waqas et al., 2019). Therefore, hydropriming is thought to enhance germination and growth by stimulating pre-germinative processes.
The cotyledons developed normally in all treatments, and the hypocotyl that grew after hydropriming in blue light was purple compared to the other treatments (Fig. 2). In Fragaria × ananassa (Kadomura-Ishikawa et al., 2013) and Myrica rubra (Shi et al., 2014) blue light promoted anthocyanin accumulation. Blue light was found as the most efficient wavelength for enhancing anthocyanin production, which may be due to a difference in the degree of anthocyanin biosynthesis in plant cells (Thwe et al., 2014; Lee and Oh, 2021). Interestingly, pretreatment with hydropriming and light quality, not the growth process, affects growth. In addition, seed pretreatment does not negatively affect the external quality indicators such as shape and size during sprout cultivation.
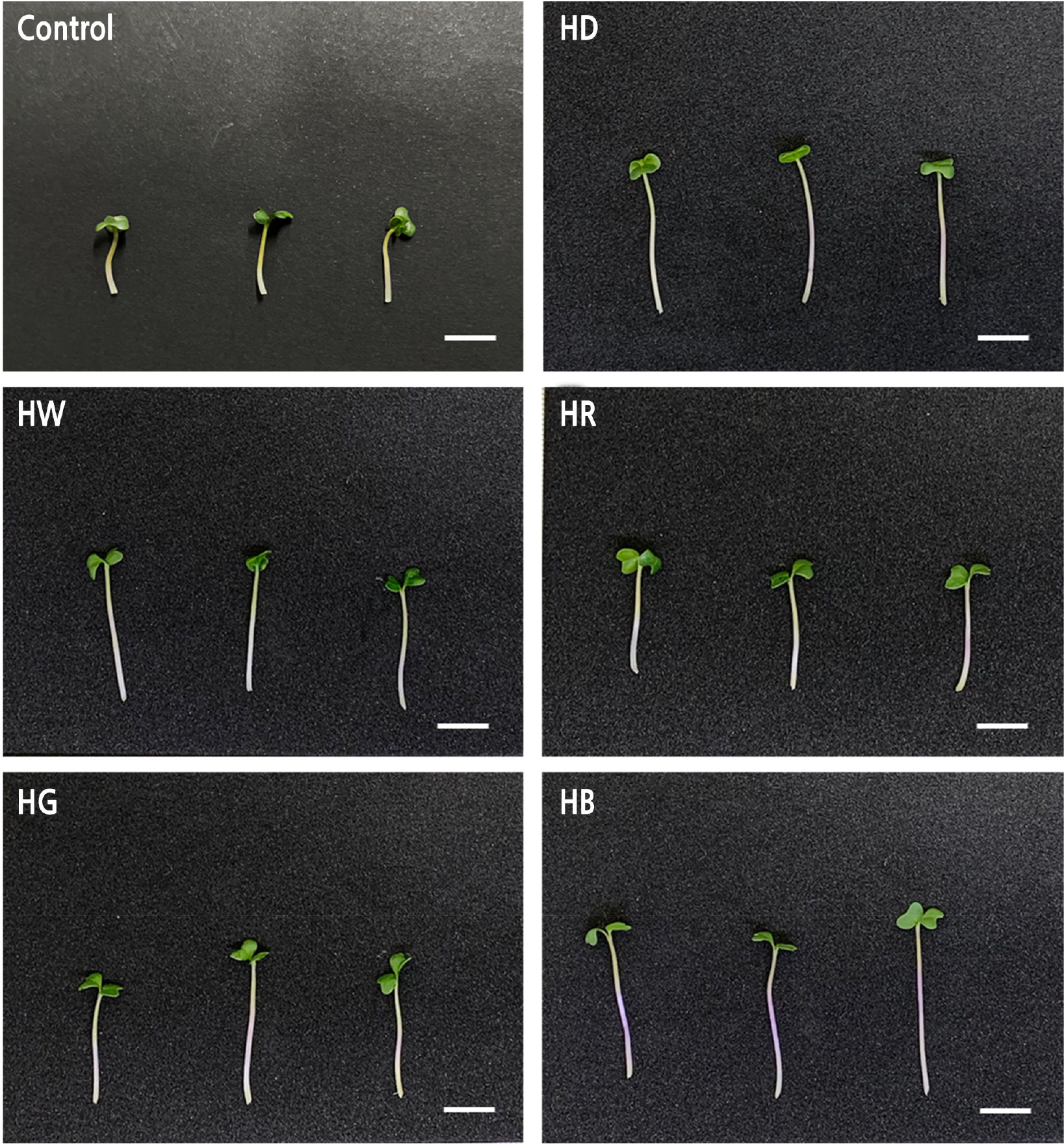
Fig. 2.
Growth of Brassica oleracea var. capitate sprouts as influenced by the hydropriming and light treatments. Scale bar = 1 cm. Control, soaked for 24 h without drying; HB, hydro-primed with blue light; HD, hydro-primed under dark; HG, hydro-primed with green light; HR, hydro-primed with red light; HW, hydro-primed with white light.
Effect of the Extraction Solvent on Antioxidant Content and Free Radical Scavenging Activity of Cabbage Sprouts
The TPC of the cabbage sprouts was 118.5 and 125.6 mg TAE·g-1 in 80% ethanol and distilled water extracts, respectively, which was approximately 1.3 times higher than that of 100% ethanol (Fig. 3). The TFC of the cabbage sprouts was 69.9–141.8 mg NE·g-1, which was significantly higher in both treatments, except for the distilled water extract. In the case of Artemisia annua extract, the TPC and TFC were higher in the mixed solvent than in water and ethanol alone (Ryu et al., 2011; Kim and Kim, 2020). Extraction of phenolic compounds with a mixed solvent is known to be more suitable than extraction with a single solvent (Goli et al., 2005; Turkmen et al., 2006). According to Razali et al. (2012), the aqueous solvent has a better extraction power than the pure solvent because the mixing of non-polar solvent and water may increase the polarity index of solvents and hence may further enhance its extraction efficiency. In our study, among the extraction solvents, 80% ethanol showed significantly high values of polyphenols, which can be postulated from the results that the difference was affected by the polarity.
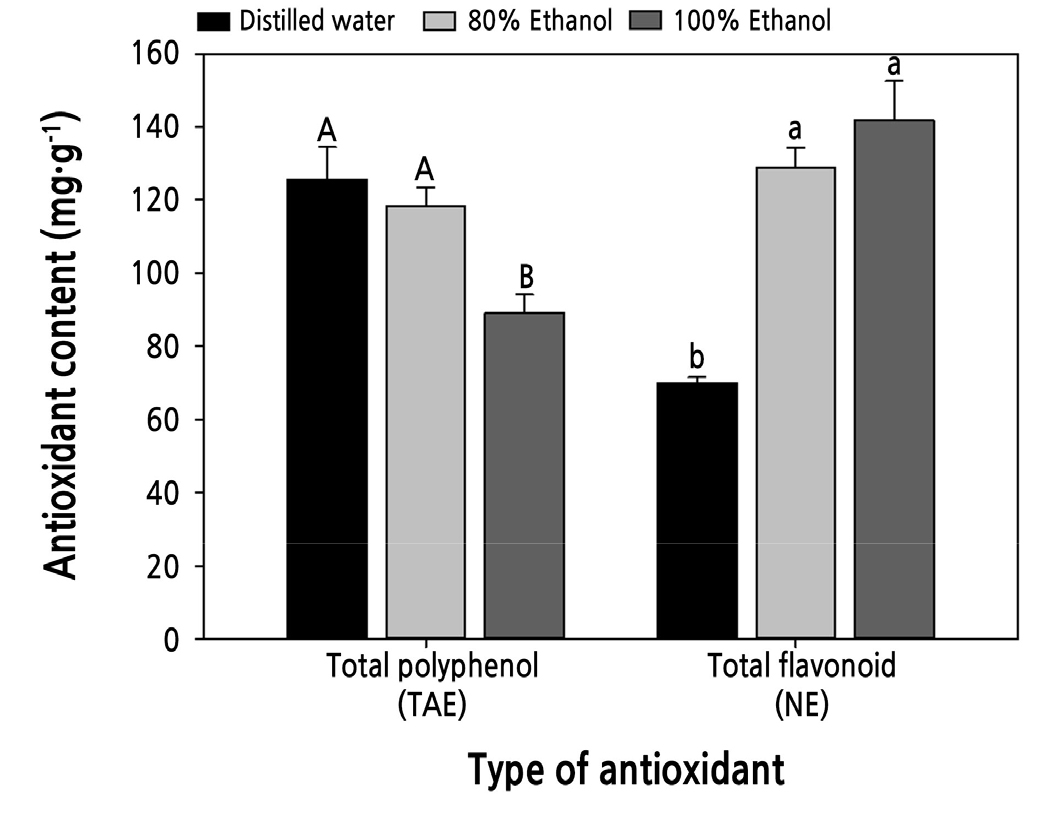
Fig. 3.
Antioxidant contents in the extracts of different solvents. Uppercase letters indicate the statistical difference of total polyphenol contents (p < 0.05), and lowercase letters indicate the statistical difference of total flavonoid contents (p < 0.05). Data presented are means with standard errors (n = 3).
The RC50 of ABTS radical scavenging activity of distilled water, 80% ethanol, and 100% ethanol were 1.77, 1.40, and 2.43 mg·mL-1,respectively, and the RC50 of DPPH radical scavenging activity were 2.09, 1.42, and 2.20 mg·mL-1, respectively (Fig. 4). For the different solvents, the ABTS and DPPH radical scavenging activity of their extracts showed similar tendencies, and the 80% ethanol extract showed the highest scavenging activity. This result is probably due to the mixing of water and ethanol, which facilitated the elution of phenolic compounds (Shin and Lee, 2011). Therefore, we selected 80% ethanol as the extraction solvent for subsequent experiments.
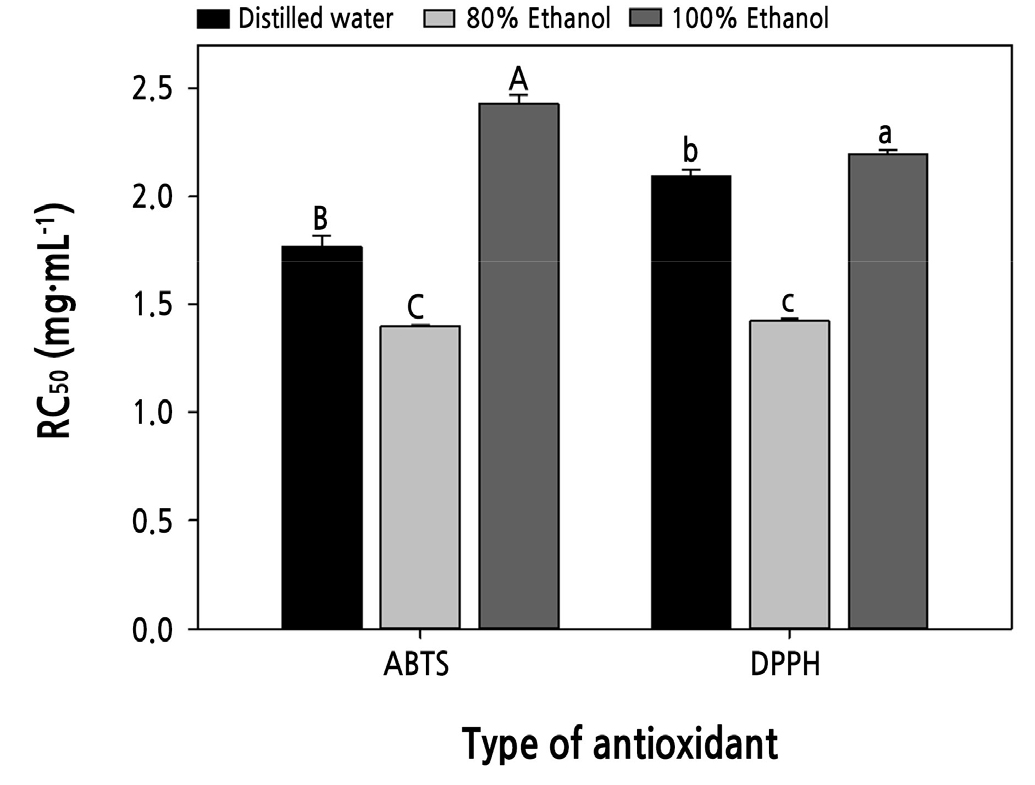
Fig. 4.
The free radical scavenging activity depending on the solvent used for extraction. Uppercase letters indicate the statistical difference of ABTS radical scavenging activity (p < 0.05), and lowercase letters indicate the statistical difference of DPPH radical scavenging activity (p < 0.05). Data presented are means with standard errors (n = 3).
Antioxidant Content and Free Radical Scavenging Activity of Pretreated Cabbage Sprouts
The treatments showed significantly higher bioactive compounds with 95.4–120.4 mg TAE·g-1 and 112–157 mg NE·g-1 respectively in TPC and TFC compared to the control (Fig. 5). Blue light can increase the TPC and TFC when applied during germination of sprouts such as Brassica napus (Park et al., 2019), B. oleracea (Acharya et al., 2016), and Pisum sativum (Liu et al., 2016). In particular, flavonoid synthesis mostly increases when exposed to the blue spectrum (Taulavuori et al., 2016; Nam et al., 2018), and treatment with different light qualities is commonly performed during the greening stage of sprout cultivation. Our study confirms that exposure to various light qualities during hydropriming at the seed stage can result in different TFC and TPC of sprouts.
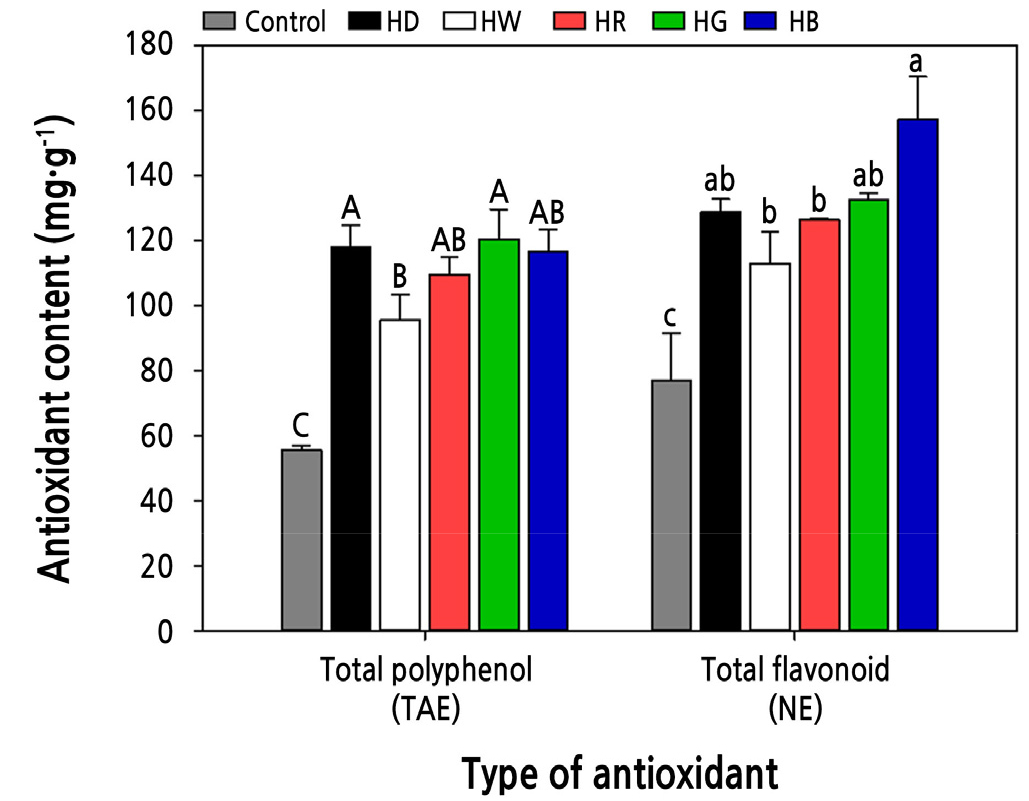
Fig. 5.
Antioxidant contents as influenced by the hydropriming and light quality treatments. Uppercase letters indicate the statistical difference of total polyphenol contents (p < 0.05), and lowercase letters indicate the statistical difference of total flavonoid contents (p < 0.05). Data presented are means with standard errors (n = 3). Control, soaked for 24 h without drying; HB, hydro-primed with blue light; HD, hydro-primed under dark; HG, hydro-primed with green light; HR, hydro-primed with red light; HW, hydro-primed with white light.
The RC50 of ABTS radical scavenging activity differed significantly depending on the treatment, and at the red, green, and blue wavelengths, the RC50 was 1.15, 1.12, and 1.12 mg·mL-1, respectively, which were effective at promoting ABTS radical scavenging activity (Fig. 6). The RC50 of DPPH radical scavenging activity levels also varied significantly depending on the light treatment, between 1.27–1.69 mg·mL-1. Among them, blue light resulted in an RC50 of 1.27 mg·mL-1, which was the most effective light quality for promoting DPPH radical scavenging activity. According to a previous study, the antioxidant activity of sprouts is positively correlated with flavonoid content and is greatly affected by light quality (Qian et al., 2016; Zhang et al., 2020). For example, irradiation with blue light with high photon energy significantly increased the TFC in kale and basil (Smith et al., 2017; Bian et al., 2018). In this study, when blue light was used during the hydropriming of cabbage seeds, the TFC increased, and the ABTS and DPPH radical scavenging abilities were similar. This suggests an association between the flavonoid content and antioxidant activity of the sprouts.
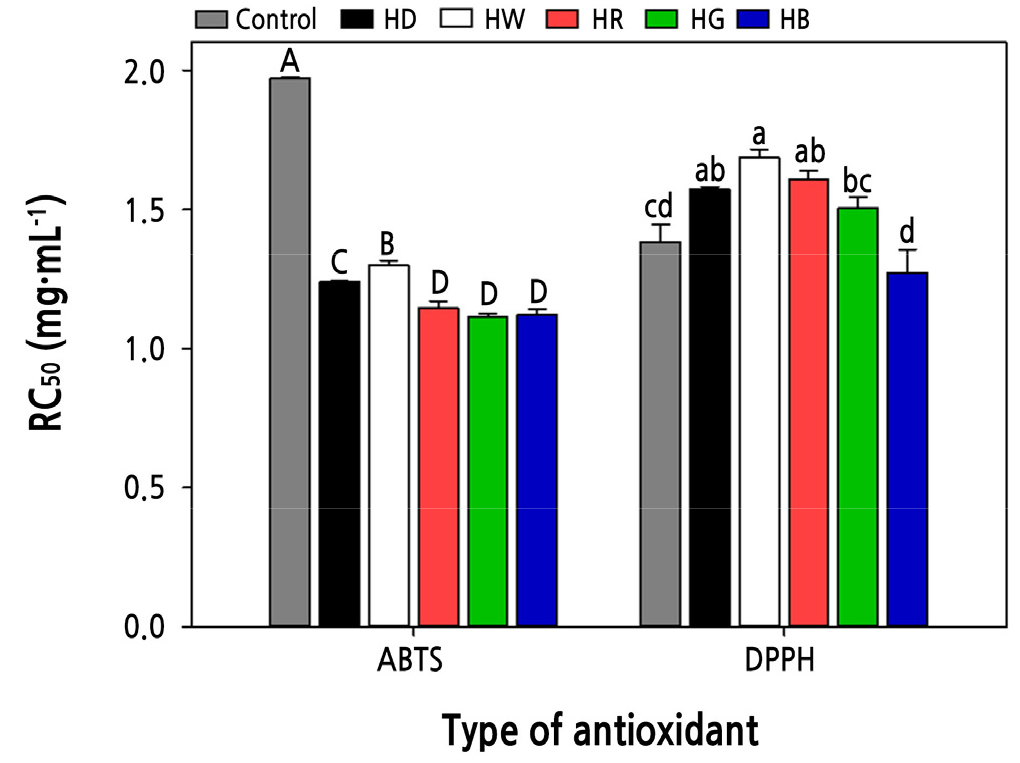
Fig. 6.
The free radical scavenging activity as influenced by the hydropriming and light quality treatments. Uppercase letters indicate the statistical difference of ABTS radical scavenging activity (p < 0.05), and lowercase letters indicate the statistical difference of DPPH radical scavenging activity (p < 0.05). Data presented are means with standard errors (n = 3). Control, soaked for 24 h without drying; HB, hydro-primed with blue light; HD, hydro-primed under dark; HG, hydro-primed with green light; HR, hydro-primed with red light; HW, hydro-primed with white light.
Conclusions
Hydropriming of B. oleracea seeds under various light qualities increased the biomass of sprouts and significantly increased the content of bioactive compounds and antioxidant activity. Therefore, this study suggests that hydropriming and light quality pretreatment of B. oleracea seeds can increase the bioactive compounds and antioxidant activity of the sprouts. Additional studies are needed to investigate the relationship between hydropriming and light quality to increase the bioactive compounds in sprouts and reduce the number of abnormal seedlings.