Introduction
Materials and Methods
Isolation of Pathogenic Fungi
Genomic DNA Extraction
Identification of Pathogenic Fungi
Antifungal Susceptibility Test
Microdilution Assay
Spray Treatment
Application of PAA to Pears
Results
Discussion
Introduction
Black stain, also known as skin sooty dapple disease (Park et al., 2008b), is a postharvest disease occurring on fruit skin of Niitaka pears (Pyrus pyrifolia Nakai) during cold storage (Chun et al., 2010). This skin disorder, which is manifested as amorphous dark brown spots, does not affect the pears’ taste (Chun et al., 2010), however it causes considerable economic losses to pear production (Mari et al., 2003; Park et al., 2008b). During the growth period of Niitaka pears, black stain frequently occurs on the fruits’ surface when fruit-wrapping bags with low air permeability and high hygroscopicity are used (Hong et al., 2003), and its incidence is most severe in years with excessive precipitation (Kim et al., 2006). High humidity in cold-storage chambers have also been shown to severely increase black stain occurrence (Yun et al., 2000; Hong et al., 2003; Moon et al., 2014). Fungal pathogens are the predominant causal factor of postharvest diseases (Mari et al., 2003), and Gloeodes pomigena (Hong et al., 2003), Cladosporium sp. (Park et al., 2008b, 2008c), and Mycosphaerella graminicola (Nam et al., 2014) were identified as pathogens causing black stain in Niitaka pears.
Chemical fungicides are the primary means of controlling postharvest diseases and have shown satisfactory results over the past half century (Tripathi and Dubey, 2004; Mari et al., 2014). Until recently, to reduce postharvest losses during cold storage, chemical fungicide treatments were applied pre- and postharvest in many pear orchards. In previous studies, active components such as benzimidazoles (thiabendazole), anilinopyrimidines (pyrimethanil), and phenylpyrroles (fludioxonil) have been shown to be effective in controlling postharvest decay of pears (Park et al., 2008a; Sugar and Basile, 2008; Lutz et al., 2017; Ali et al., 2018). However, the use of chemical fungicides has been gradually restricted due to growing concerns among consumers and regulatory agencies regarding high levels of fungicide residues on fruits as well as increasing fungicide resistance in fungal pathogens (Mari et al., 2014; Wisniewski et al., 2016). Furthermore, intensive use of fungicides may pollute the environment and elicit potential adverse effects on human health, including potential carcinogenicity, teratogenicity, and acute and chronic toxicity (Komárek et al., 2010; Oruc, 2010; Rouabhi, 2010). As discussed above, growing demand for chemical residue-free produce, along with decreasing options for managing postharvest disease due to de-registration of fungicides, motivated a search for safe management methods that may effectively replace conventional fungicides.
Peracetic acid (PAA) is a strong oxidizing agent and is commonly used as an effective disinfectant in various industrial fields such as food, beverage, medical, pharmaceutical, textile, and pulp processing as well as sewage treatment due to its bactericidal, viricidal, fungicidal, and sporicidal effects (Malchesky, 1993; McDonnell and Russell, 1999; Kitis, 2004; Kim and Huang, 2021). PAA is converted to innocuous acetic acid and oxygen, remains active in the presence of organic matters, and is effective over a wide range of temperatures (0 to 40°C) and pH values (3.0 to 7.5) (Kitis, 2004; Vandekinderen et al., 2009). Moreover, PAA shows little ecotoxicity and produces few disinfection by-products, e.g., trihalomethanes and haloacetic acids, unlike chlorine-based disinfectants such as hypochlorite and chlorine dioxide, which are commonly used in wastewater treatment (Chhetri et al., 2020). Therefore, to reduce environmental pollution and risks to health and safety of workers, application of PAA, which has been shown to be effective against various pathogenic fungi, may be an attractive alternative to lower the incidence of black stain in Niitaka pears.
The aim of this study was to assess antifungal effects of PAA on black stain–causing fungi using various susceptibility tests. In addition, we examined preventive effects of PAA on the occurrence of black stain through application to pears. To this end, antifungal effects of PAA on isolated fungi were compared with NaOCl, which is frequently used as a disinfecting agent, using various susceptibility tests including disc diffusion, mycelial growth tests, and a microdilution assay. Furthermore, the incidence of black stain in pears treated with or without PAA was examined after cold storage for 90 days. Our results provide useful insights into the control of postharvest disease of Niitaka pears during cold storage.
Materials and Methods
Isolation of Pathogenic Fungi
Unless otherwise stated, all reagents were purchased from Sigma-Aldrich, Thermo Fisher Scientific, or Merck Millipore. Pears showing symptoms of black stain were collected from commercial orchards (Naju, Korea) and were disinfected using 3% sodium hypochlorite and 70% ethanol, followed by rinsing twice with distilled water. Fungi were isolated from skin lesions of infected pears and were cultivated on potato dextrose agar (PDA; Difco, Lawrence, KS, USA) at 24°C for 2 weeks. A piece of fungal mycelium was removed from solid cultures using a sterile scalpel and was placed in potato dextrose broth (PDB; Difco) followed by incubation at 24°C under agitation (120 rpm) for 7 days. After incubation, mycelia were harvested by filtration through cheesecloth, washed twice with ice-cold PBS, ground into a fine powder in liquid nitrogen using a mortar and pestle, and were stored at –60°C until further use.
Genomic DNA Extraction
Isolation of genomic DNA was performed according to the method described by Yuan (2019). To 100 mg powdered mycelium, 750 µL CTAB DNA extraction buffer (100 mM Tris-HCl [pH 8.0], 1.4 M NaCl, 20 mM EDTA, 2% [w/v] cetyltrimethylammonium bromide [CTAB], 2% [w/v] polyvinylpyrrolidone 40 [PVP-40], and 5 mM ascorbic acid) was added, after which the mixture was vortexed and incubated at 60°C for 15 min under inversion every 5 min. After incubation, 500 µL chloroform:isoamyl alcohol (24:1; v/v) was added, and the mixture was thoroughly mixed by inverting. The mixture was then centrifuged at 13,000 × g for 10 min at room temperature (22.5 ± 1°C), and the aqueous upper layer (approximately 500 µL) was transferred to a new tube. DNA was precipitated by adding 10% (v/v) 7.5 M ammonium acetate and 1 volume of chilled ethanol and was incubated at –20°C for 2 h. DNA was pelleted by centrifugation at 13,000 × g for 15 min at 4°C and was washed twice using 70% ethanol. The recovered DNA pellet was resuspended in 40 µL nuclease-free water and was stored at –20°C until further use.
Identification of Pathogenic Fungi
DNA purity and concentration were measured using an Eppendorf BioSpectrometer Basic (Eppendorf AG, Hamburg, Germany). Internal transcribed spacer (ITS) regions and large subunit (LSU) of nuclear ribosomal RNA gene were amplified using the primer pairs ITS1/ITS4 (White et al., 1990) and LROR/LR7 (Vilgalys and Hester, 1990), respectively. PCR was performed in a total reaction volume of 20 µL comprising 10 ng genomic DNA, 0.5 µM of each primer, 0.2 mM dNTPs, 1× PCR buffer, and 0.5 U Taq DNA polymerase (Takara, Tokyo, Japan) using a Tprofessional Thermocycler (Biometra, Göttingen, Germany). Thermocycling was performed at 98°C for 2 min, followed by 35 cycles of 98°C for 30 sec, 52°C for 50 sec, and 72°C for 1 min 30 sec and a final step of 72°C for 5 min. PCR products were visualized by electrophoresis on a 1% (w/v) agarose gel in 0.5% Tris-acetate EDTA buffer followed by staining using ethidium bromide and UV exposure. All positive amplicons were sequenced using a Bigdye Terminator cycle sequencing kit v3.1 on the ABI 3730XL DNA Analyzer by SolGent Co., Ltd. (Daejeon, Korea).
Antifungal Susceptibility Test
Antifungal susceptibility of C. porophorum was tested using a disc diffusion assay. Spores were collected by scraping the surface of sporulated fungi grown on PDA for 7 days using a sterile toothpick and were suspended in PDB containing 0.1% (v/v) Tween 20. The suspension was vortexed vigorously to break up aggregates and was adjusted to a concentration of 1 × 108 spores/mL using a hemocytometer (Marienfeld, Lauda-Königshofen, Germany). An aliquot (200 µL) of spore suspension was spread uniformly over the surface of the PDA medium. PAA dilutions were prepared at concentrations ranging from 0.0025% to 0.16% in PBS. Sterile paper discs (8-mm diameter) were treated with 50 µL of the PAA dilutions and were placed on the surface of PDA medium. PBS was used as negative control, and NaOCl was used as positive control. The plates were then incubated at 24°C for 2 days, and the growth inhibition zone around the discs was measured. Clear areas larger than 10 mm were considered growth inhibition zones.
In one set of experiments, paper discs treated with different PAA dilutions were applied to fungal mycelium grown on PDA for 5 days. Briefly, spore suspension was applied to the center of fresh PDA, and after 5 days of inoculation, sterile paper discs were placed at a distance of 1 cm from the edge of the growing mycelium. The sterile discs were impregnated with 50 µL of different PAA dilutions at concentrations ranging from 0.0025% to 0.16%. Inhibition of mycelial growth around the discs was examined for 3 days.
Moreover, antifungal effects on C. porophorum were assessed by comparing mycelial growth of fungi treated with PAA or NaOCl to that of untreated fungal colonies. Spore suspensions were prepared in PDB containing 0.1% (v/v) Tween 20. Spore suspension (50 µL at 5 × 104 spores/mL) containing various concentrations of either PAA or NaOCl was applied to the center of the PDA plates, and after 5 days of incubation, mycelial growth of fungi treated with PAA or NaOCl was compared to that of the control. Inhibition of mycelial growth was evaluated through visual observation.
Microdilution Assay
Fungal metabolic activity was monitored using a colorimetric WST-1 assay (05015944001; Roche Diagnostics GmbH, Mannheim, Germany) to measure mitochondrial dehydrogenases activity, according to the manufacturer's instructions. Spores were collected by scraping the surface of sporulated fungi grown on PDA for 7 days using a sterile toothpick and were suspended in PDB containing 0.1% (v/v) Tween 20. Aliquots (100 µL) of spore suspension (5 × 104 spores/mL) containing different concentrations of PAA at final concentrations of 0.000625% to 0.08% were transferred to individual wells of a 96-well microplate. The plate was then incubated at 24°C for 24 h. NaOCl was used as positive control. To confirm the presence of viable fungal spores, aliquots (10 µL) of WST-1 were added to the wells, and the reactions were incubated at 24°C for 18 h. The minimum inhibitory concentration (MIC) of PAA was defined as the lowest concentration that reduced fungal metabolic activity by more than 80%. To quantify the produced yellow-colored formazan, absorbance at 450 nm was measured using a µquant microplate reader (BioTek Instruments, Winooski, VT, USA), and the measured absorbance was adjusted by subtracting background absorbance at 570 nm. All assays were performed in triplicates.
Aliquots collected from the wells corresponding to the MIC and higher concentrations were subcultured onto PDA plates and incubated at 24°C for 48 h. The minimum fungicidal concentration (MFC) of PAA was defined as the lowest concentration inhibiting visible fungal growth on PDA medium. The MFC/MIC ratio was calculated to determine whether the compound exerted fungicidal (MFC/MIC ≤ 4) or fungistatic (MFC/MIC > 4) effects (Hazen, 1998).
Spray Treatment
Antifungal effects of PAA were additionally tested by spraying it directly on the surface of C. porophorum grown on PDA. After placing an agar block (10-mm diameter) of a 7-day-old culture of C. porophorum on the center of fresh PDA, the plates were incubated at 24°C for 2 days. PAA dilutions at different concentrations (0.025%–0.5%) were sprayed (0.5 mL per dish) five times directly on cultures grown on PDA, and sterile distilled water was used as a negative control. The plates were then incubated at 24°C for 4 days to assess inhibition of fungal growth.
Application of PAA to Pears
Twenty boxes of commercially packed Niitaka pears (uniform size; approximately 10–13 pears per 5-kg box) were procured from an agricultural cooperative located in Sinan-gun, Korea, on October 12, 2020. To evaluate the efficacy of PAA in reducing the occurrence of black stain, pears from 10 of the boxes were treated with 0.1% PAA, and the others were left untreated. All pears were then stored at 0.0 ± 0.5°C for 90 days after which the incidence of black stain was recorded.
Results
Several fungal isolates isolated from skin lesions of pears showing symptoms of black stain were examined. Fungal colonies were dense, velvety textured, and of olivaceous-green color with grey, narrow, and undulated margins. Most strains resembled the previously reported genus Cladosporium in morphological observation of fungal colonies grown on PDA. For species confirmation, the ITS region was sequenced. The obtained sequence was compared to those in GenBank using BLAST (https://blast.ncbi.nlm.nih.gov/Blast.cgi). No interspecific discrimination was observed in ITS sequence obtained from fungal isolates. The ITS region of fungal isolates displayed 99–100% nucleotide sequence identity with those of Cladosporium sp. (KC662230, KT150671), C. cladosporioides (MF072625, MG890303), C. xanthochromaticum (MG836599), and C. aciculare (MG836594). After isolates were confirmed as belonging to the genus Cladosporium, the gene region encoding the LSU was additionally sequenced. For LSU, BLAST analysis of a 465-bp fragment from isolates showed 92% identity to C. porophorum (syn Alternariamalorum var. polymorpha, AY354912). These results, together with the preliminary identification based on morphological observations of fungal colonies, indicated that these strain belongs to C. porophorum. Among the fungal genera isolated from skin lesions of pears, Cladosporium was the most predominant, followed by Alternaria and Penicillium (data not shown).
Disc diffusion assays are frequently used to evaluate antifungal effects of drug for fungal pathogens. Considerable inhibition of fungal growth was observed on discs treated with 0.16% or 0.08% NaOCl, whereas no inhibition was observed at concentrations below 0.08% (Fig. 1A). Growth inhibition on discs treated with 0.16% or 0.08% PAA was stronger than that after treatment with the same concentrations of NaOCl 2 days after incubation. The inhibition zones around the disc treated with 0.16% or 0.08% PAA remained constant until the fifth day after incubation, whereas at this time point, zones around discs treated with the same concentrations of NaOCl were significantly reduced in diameter compared to those produced on the second day of incubation. The growth inhibitory effect of PAA applied to the growing mycelium occurred at concentration ranges of 0.04%–0.16% until 2 days after incubation (Fig. 1B). However, no inhibition was observed at corresponding concentrations of PAA after 3 days of incubation. Antifungal effects were also evaluated by measuring the inhibition of mycelial growth due to various concentrations of either PAA or NaOCl. Complete inhibition of mycelial growth was observed at PAA concentrations exceeding 0.02% after 5 days of incubation (Fig. 1C). By contrast, concentrations of NaOCl that completely inhibited mycelial growth were above 0.04% (Fig. 1D).
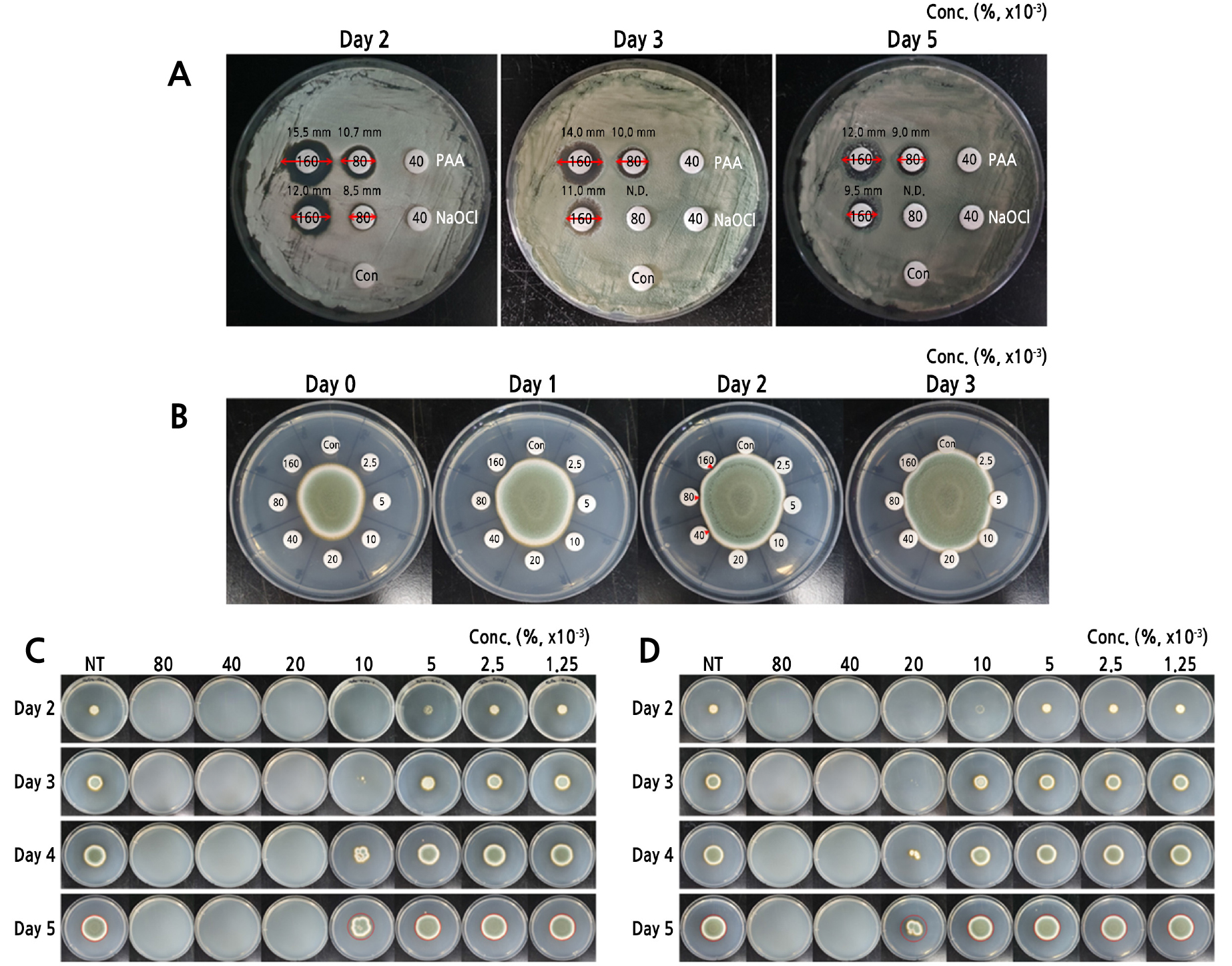
Fig. 1.
Comparison of antifungal effect of PAA and NaOCl against Cladosporium porophorum. (A) Inhibition zones were observed on discs treated with either 0.16% or 0.08% of PAA and 0.16% of NaOCl after 5 days of incubation. Red arrows indicate the diameter of the inhibition zone. (B) Growth inhibition was observed around discs treated with PAA at concentrations >0.04% until 2 days of incubation, as indicated by red arrowheads. (C, D) The columns in each panel indicate the final concentration (in percent; × 10-3) of PAA (C) or NaOCl (D) applied to the spore suspension. The red circle indicates the size of mycelial growth of non-treated cultures after 5 days of incubation. Note that mycelial growth of fungi treated with PAA or NaOCl was partially inhibited at concentrations of 0.01% and 0.02%, respectively. One of three replicate experiments is shown.
Antifungal susceptibility of conidial suspension was examined at concentrations of PAA or NaOCl ranging from 0.000625% to 0.08% using a WST-1-based microdilution assay measuring fungal metabolic activity. When spore suspension was treated with >0.0025% PAA or >0.005% NaOCl, no yellow-colored formazan formation was observed (Fig. 2A). WST-1 is converted to yellow-colored formazan by mitochondrial dehydrogenase activity, which exclusively occurs in viable cells; the amount of produced yellow formazan is thus proportional to the number of viable cells in conidial suspension. Based on the results of the microdilution assay, spore viability was up to 90% at PAA concentration of 0.00125%, while at concentrations of 0.005%–0.08%, more than 95% of this viability was lost (Fig. 2B). The MIC and MFC values of PAA against C. porophorum were found to be 0.0025% and 0.005%, respectively (Fig. 2C); NaOCl showed MIC and MFC values of 0.005% and 0.01%, respectively. The MFC/MIC ratio indicated that PAA and NaOCl had fungicidal effects on C. porophorum.
To confirm susceptibility of hyphal fragments, not of conidia, to the antifungal agents, the spore suspension was pre-incubated for 24 h before treatment with various concentrations of PAA or NaOCl. After pre-incubation, fungal spores had formed hyphal fragments. Hyphal fragments exhibited no metabolic activity when exposed to >0.0025% PAA or >0.005% NaOCl (Fig. 2D). The MIC value of PAA regarding hyphal fragments was equal to that in conidial suspensions.
The required compound concentrations to inhibit fungal growth may vary depending on conidia density, type of fungal inoculum, or application method of the antifungal drug, as shown in the disc diffusion, mycelial growth, and microdilution assays. Conidial susceptibility to PAA decreased with increasing spore density, and metabolic activity in conidial suspensions with 5 × 104 to 5 × 106 spores was completely inhibited at PAA concentrations >0.005% (Fig. 2E). However, at 5 × 107 spores, metabolic activity was retained even at 0.04% PAA.
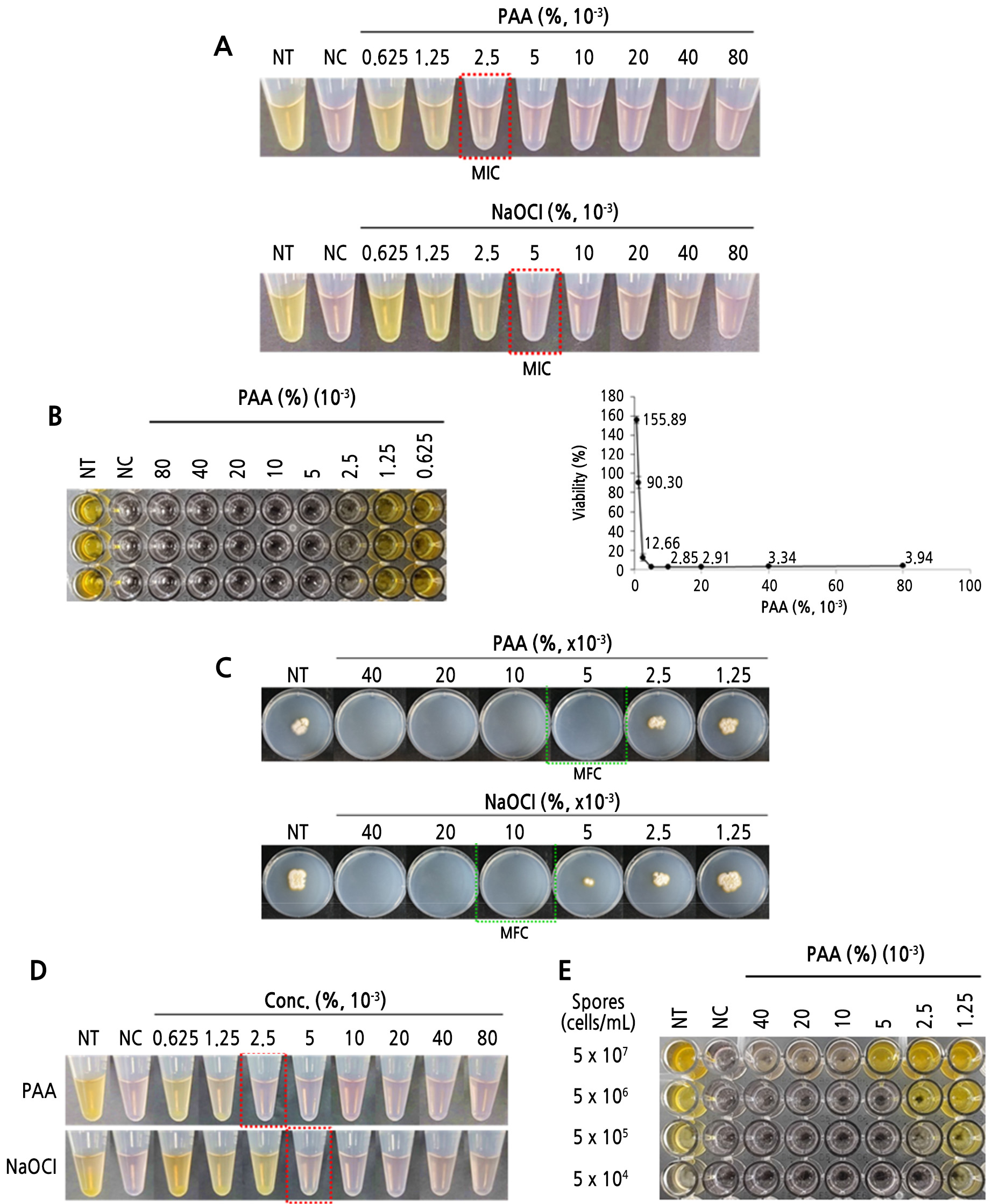
Fig. 2.
MIC and MFC of PAA and NaOCl for Cladosporium porophorum. (A) In the presence of >0.0025% PAA or >0.005% NaOCl, fungal spores lost their metabolic activity and did not form yellow-colored formazan. The MICs of PAA and NaOCl were 0.0025% and 0.005%, respectively, as indicated by the red dotted box. NT, non-treated (no drug added); NC, negative control (media instead of spore suspension). (B) Fungal viability was determined by monitoring the amount of yellow formazan formed by cleavage of WST-1. Spore viability was up to 90% at PAA concentration of 0.00125%, while at concentrations of 0.005%–0.08%, more than 95% of this viability was lost. (C) The MFCs of PAA and NaOCl were 0.005% and 0.01%, respectively, as indicated by the green dotted box. (D) When hyphal fragments were exposed to >0.0025% PAA or >0.005% NaOCl, no metabolic activity was observed, as indicated by the red dotted box. (E) Photograph showing that susceptibility to PAA decreased with increasing conidia abundance.
Antifungal susceptibility of C. porophorum was examined by spraying the PAA directly on the surface of growing mycelium. PAA concentrations of 0.025%–0.5% were used, as inhibitory effects on growing mycelium were unclear at lower PAA concentrations (0.04%–0.16%). Inhibition of mycelial growth was evident in PDA plates treated with >0.25% PAA until 4 days after treatment (Fig. 3). However, the plate treated with 0.05% or 0.025% PAA showed no inhibition effect on mycelial growth.
To assess preventive effects of PAA on black stain, the incidence of black stain was examined after 90 days of cold storage in pears treated with or without 0.1% PAA. Differences in the occurrence of black stain between treatments are shown in Fig. 4. A considerable reduction in the occurrence of black stain was observed in pears treated with 0.1% PAA, and the incidence rate was approximately half (31.1% ± 1.9%) compared to that in untreated pears (58.8% ± 4.5%).
Discussion
Fruits are considerably susceptible to infection by fungal pathogens due to their low pH, high moisture content, and nutrient composition (Tripathi and Dubey, 2004). In addition, some fungal pathogens are known to be the main cause of postharvest diseases (Mari et al., 2003). Thus, to control postharvest diseases, powerful tools such as chemical fungicides have been introduced, and they were effective to some extent (Tripathi and Dubey, 2004; Mari et al., 2014). However, potential problems such as fungicide resistance in pathogens as well as chemical residues and associated toxicity (Mari et al., 2014; Wisniewski et al., 2016) have led to the demand for alternative methods of managing postharvest diseases. A number of approaches such as modified atmosphere packaging (Yoon et al., 2018), 1-methylcyclopropene treatment (Yoo et al., 2019; Win et al., 2021), and edible coatings (Shah and Hashmi, 2020) are being studied to reduce the occurrence of physiological disorders or post-harvest decay that may occur during cold storage of fruits.
The fungal isolates obtained in this study were identified as the type species of C. porophorum. Previously, Park et al. (2008b, 2008c) reported that Cladosporium sp. causes black stain in Niitaka pear. Cladosporium is among the most abundant fungi worldwide, is commonly isolated from soil and organic matter (Bensch et al., 2012), and is distributed in various environments such as orchards, packing houses, and markets (Watanabe et al., 2011). Other identified fungal genera, including Alternaria and Penicillium, which make up a minor portion of fungal isolates obtained from skin lesions of pears, are also known to cause black stain and postharvest decay in Niitaka pears (Park et al., 2008c; Lee et al., 2009; Nam et al., 2014; Min et al., 2015).
The susceptibility results comparing inhibitory effects of PAA and NaOCl on C. porophorum showed that PAA inhibited mycelial growth or metabolic activity at a concentration of two-fold lower than NaOCl (Figs. 1A, 1C, 1D and 2A). Antifungal efficacy of PAA is superior to that of NaOCl, as shown regarding food spoilage fungi (Lemos et al., 2020; Stefanello et al., 2020; Stefanello et al., 2021). Stefanello et al. (2021) showed that 1% PAA achieved complete inactivation (>5-log reduction) of Aspergillus brasiliensis at 20–40°C within 15 min, whereas 1% NaOCl did not achieve 3-log reduction. Similarly, in a different experiment by Stefanello et al. (2020), PAA exhibited the highest antifungal efficacy against heat-resistant molds, such as the Aspergillus and Paecilomyces, at concentrations of 0.6% and 1%, whereas NaOCl was not effective in eliminating these filamentous fungi. According to Lemos et al. (2020), PAA exerts superior antifungal effects (>5-log reduction) on Aspergillus species producing ochratoxin A at the tested concentrations (0.3%–1%), whereas NaOCl achieved 3-log reduction only in A. westerdijkiae, out of 15 examined strains. However, Bernardi et al. (2018) investigated antifungal effects of commercial sanitizers on several fungi that cause food spoilage, and NaOCl was more effective against C. cladosporioides than PAA, at the tested concentrations.
Antifungal susceptibility tests under in vitro conditions typically use a spore suspension as fungal inoculum. However, susceptibility of fungi to antifungal agents may differ depending on whether they occur as spores or hyphae (Van de Sande et al., 2010). Furthermore, fungal adhesion to the host cell surface is the first step in saprophytic interactions, and hydrophobicity of fungal hyphae has been known to play a crucial role in this adherence process (Lipke, 2018). Therefore, susceptibility results from fungal hyphae or hyphal clumps as a fungal inoculum can better reflect the fungal status in infected tissue than those of spore-based tests (Van de Sande et al., 2010), and antifungal agents can subsequently be applied at an effective concentration, even in the field.
In the assessment of growth inhibitory effects of PAA on C. porophorum through various antifungal susceptibility tests, the required compound concentrations to inhibit fungal growth varied depending on conidia density, type of fungal inoculum, and application method of antifungal drug. Antifungal susceptibility results obtained using conidial suspension showed that PAA completely inhibited fungal growth and metabolic activity at concentrations of 0.02%–0.08% and 0.005%, respectively. However, the PAA concentrations exceeding 0.1% were required to inhibit fungal growth when applied to growing mycelium. Low susceptibility to PAA is probably caused by insufficient concentrations of PAA or by the fact that fungal mycelium is less accessible to PAA than conidia. Overall, our results indicated that high concentrations of PAA, at least 0.1% or higher, were required to inhibit growth of fungal mycelium. These results were consistent with those of Van de Sande et al. (2010), who found that conidia and hyphal fragments showed equal susceptibilities to amphotericin B, and the metabolic activity of fungal clumps was inhibited at relatively high concentrations of antifungal agents. Other studies also performed susceptibility tests with different types of fungal inoculums and found that higher concentrations of antifungal agents were required to inhibit metabolic activity in hyphal clumps compared to conidial suspensions (Mowat et al., 2007; Perkhofer et al., 2007). Although the susceptibility of conidia and hyphal fragments to PAA was equal (Fig. 2A and 2D), we used higher concentrations of PAA in our experiments due to the diverging results regarding antifungal susceptibility.
After applying PAA to pears, a considerable reduction in the occurrence of black stain was observed in fruits treated with 0.1% PAA (Fig. 4). The incidence rates of black stain in PAA-treated and untreated fruits were 31.1% ± 1.9% and 58.8% ± 4.5%, respectively. Strong oxidizing agents such as PAA are commonly applied as disinfectants (Malchesky, 1993; McDonnell and Russell, 1999; Kitis, 2004) and may be an ideal way to reduce viability of fungal pathogens that cause black stain. The antifungal activity of PAA has been thoroughly investigated, and some studies assessed the effects of PAA on fungal pathogens that cause postharvest decay (Mari et al., 1999; Chen et al., 2004; Korukluoglu et al., 2006; Smilanick et al., 2014).
Previous studies on the toxicity of PAAs do not raise concerns for mutagenicity (Monarca et al., 2002; Monarca et al., 2003), carcinogenicity (Müller et al., 1988), or reproductive toxicity, and the lethal doses on the species studied are generally well above the concentrations of PAA used for disinfection (Kitis, 2004). The DBPs identified in the PAA-treated water were mainly carboxylic acids and aldehydes, which are readily biodegradable (Kitis, 2004) and recognized as non-mutagenic (Monarca et al., 2002; Monarca et al., 2003), and no halogenated compounds were found (Monarca et al., 2002). On the other hand, chlorine-based disinfectants produced some DBPs suspected of being mutagenic and carcinogenic (Monarca et al., 2002; Kitis, 2004). The long-term exposure of 0.2% PAA showed no inflammation, no scar formation, and no risk of dysplasia associated with carcinogenesis in dorsal skin, and oral and vaginal mucosa of rabbits after 1 year (Müller et al., 1988).
In conclusion, PAA appears to provide better antifungal efficacy than NaOCl. In this study, we evaluated antifungal effects of PAA on C. porophorum under in vitro conditions and confirmed that PAA can reduce the occurrence of black stain after application to pears. In an antifungal susceptibility test comparing growth inhibitory effects of PAA and NaOCl on C. porophorum, PAA concentrations required to inhibit mycelial growth or metabolic activity were two-fold lower than those of NaOCl. The effectiveness of PAA in pear fruits is further highlighted by its inhibitory effect on fungal growth, which was better than that of NaOCl, stronger oxidation potential (Kim and Huang, 2021), and applicability at low temperatures unlike other disinfectants (Kitis, 2004; Vandekinderen et al., 2009). The oxidation potential of PAA (1.96 eV) is higher than that of hydrogen peroxide (1.78 eV), hypochloric acid (HOCl, 1.48 eV), and chlorine dioxide (ClO2, 1.28 eV) (Kim and Huang, 2021).
The inhibitory effect of PAA against fungi causing black stain may help counteract postharvest diseases that can be exacerbated during cold storage of Niitaka pears. The effectiveness and potential of PAA discussed above make PAA a potential alternative to control postharvest losses of Niitaka pears during cold storage with reduced risks to the environment and human health.