Introduction
Materials and Methods
Plant Materials and Growth Conditions
Microbubble Generation
Measurements
Statistical Analysis
Results and Discussion
Microbubble Analysis
Plant Growth
Conclusion
Introduction
Water scarcity is currently one of the limiting factors for food production, affecting the growing population’s need for water in the 21st century (Dubois, 2011). Irrigation, which is essential for plant cultivation, is expected to increase in developing countries with increasing population growth, and owing to climate change, the need to replace water resources utilized for crop production also is increasing (Atzori et al., 2019). As a solution, seawater, which accounts for 97% of all Earth's water, can be considered as an alternative for agricultural purposes (Yermiyahu et al., 2007). Seawater contains ions necessary for plant growth (Boyko, 1966) and is being used for plant cultivation. Research on the use of seawater as irrigation water in various plants is ongoing in many countries. Using diluted seawater increased the growth rate and sodium content of spinach leaves (Atzori et al., 2016) and did not affect growth of chard and chicory (Caparrotta et al., 2019). It has also been suggested that seawater treatment can improve tomato cultivation (Mizrahi et al., 1988). However, in another case, seawater decreased tomato yield and quality and increased total sugar content (Kim et al., 2000). Therefore, the use of seawater in agriculture can be a sustainable and resource-saving solution.
However, seawater use in agriculture may cause moisture stress, oxidative stress, membrane breakdown, and nutrient damage, leading to stunted plant growth (Munns, 2002). The continuous use of seawater can cause salt accumulation and increase free radicals in plants due to environmental stress (Lee and Oh, 1994; Ashworth, 2007). In addition, salt stress during the cultivation period causes ionic imbalance, leading to oxidative stress and nutrient deprivation (Cheong and Yun, 2007). Furthermore, the increase in salinity affects photosynthesis through the closure of pores and changes in biochemical processes, leading to the aging of leaves and slow growth (Munns and Tester, 2008; Praxedes et al., 2010); this creates an imbalance in the nutritional status and ion concentrations in plants (Läuchli and Epstein, 1990). The ionic imbalance occurs due to an increase in the concentration of Na+ in the plant, causing a disorder in metabolic activities and nutritional deficiency (Ajeet and Ramasare, 2009). NaCl inhibited water absorption in tomatoes, resulting in poor fruit development and decreased yields in the upper parts of the plants (Rhee et al., 2001). Salinity also rapidly decreased the photosynthetic rate and pore conductivity in musk melons during harvest (Kwak et al., 2003).
Microbubbles are tiny gas bubbles in water, with a size less than 50 µm in diameter, and their surfaces are negatively charged. Owing to these charges, the bubbles attract positively charged ions or materials (Takahashi, 2005; Ohnari et al., 2006). In addition, microbubbles have the advantage of being able to dissolve oxygen gas, owing to their rapid decomposition speed and long residual time in a liquid (Zhang et al., 2007). These features enable the use of microbubbles in various fields, such as in the removal of residual pesticides (Takahashi, 2009), purification of contaminated water (Khuntia et al., 2012), and culture of oysters (Onari et al., 2002). However, there are only a few reports on the use of microbubbles in agriculture. These include sterilization of cultures using ozone microbubbles (Kobayashi et al., 2012), increasing lettuce growth using air microbubbles in hydroponic cultivation (Park and Kurata, 2009), and increasing the growth of fruits and vegetables using ozone microbubbles (Ohashi-Kaneko et al., 2009). Therefore, further investigation is required on the introduction of microbubbles in the field of agriculture.
Hydroponic cultivation is a method of cultivating plants using mineral nutrient solutions in water, without soil, and includes the nutrient film technique (NFT) and the deep flow technique (DFT) (Savvas et al., 2002; Schwarz, 2012). Hydroponic systems are widely used in the production of flowers and vegetables and offer many advantages, including high productivity, fast growth, easy management, and environmental safety (dos Santos et al., 2013). Compared to conventional soil culture, hydroponic systems can be used to produce crops continuously throughout the year regardless of season and ambient temperature (Brechner et al., 1996) and are therefore becoming an attractive alternative for an increasing number of farmers. In 1996, 12,000 ha of hydroponics system area was estimated worldwide (Donnan, 1998). In 2000, this area increased to about 30,000 ha, and in 2011, it was estimated to be over 35,000 ha (Hickman, 2011; Schwarz et al., 2001). The domestic hydroponic cultivation area was 3,355 ha in 2016, which accounted for 6.4% of the total vegetable cultivation area in the facility (MAFRA, 2017), and the domestic hydroponic cultivation area in 2019 increased to 3,696 ha (MAFRA, 2019).
Romaine lettuce (Lactuca sativa L.) is one of the most widely used salad vegetables and contains many phytochemicals such as antioxidants and carotenoids (Humphries and Khachik, 2003; Nicolle et al., 2004). Since lettuce is usually eaten uncooked, it is more nutritious than other cooked vegetables, and the consumption of its first leaves and seedlings is increasing, along with the consumption of mature lettuce (Xiao et al., 2012). Lettuce is produced in many countries, such as the United States, Italy, India, and Japan, and is especially important as a commercial crop in Europe, Asia, and North and Central America (Lebeda et al., 2007; Mou, 2008). In the United States, lettuce is also widely grown in plant factories with an annual crop value of $2 billion and is cultivated over 121,000 ha (Lucier and Jerardo, 2006).
In this study, we investigated the feasibility of using high-salinity water for lettuce cultivation by applying the microbubble technique in a hydroponic system with the aim to determine how microbubbles affected growth of hydroponically grown lettuce under conditions of high salinity.
Materials and Methods
Plant Materials and Growth Conditions
The lettuce seeds used in the experiment are of the variety ‘Cos lettuce’ (Takii Seed Co., Tokyo, Japan). Seeds were sown in peat and vermiculite mix (1:1 ratio) and raised in a temperature-controlled plant growing room (4.0 × 5.7 m). Light with a PPFD (photosynthetic photon flux density) of 160 ± 15 µmol·m-2·s-1 was applied to the plants through a fluorescent lamp (CREA1-FPL55EX-L; Iwasaki Electric Co., Tokyo, Japan), and the day and night temperatures were maintained at 21 ± 1/18 ± 1°C. The photoperiod was 16 hours during the day and 8 hours at night. Fourteen days after sowing, the seedlings were separated from the mixed soil and washed, and their roots were wrapped inside urethane cubes. Thirty seedlings were placed in half-strength Yamazaki nutrient solution containing 45.4 mg·L-1 nitrogen (41.9 mg·L-1 nitrate and 3.5 mg·L-1 ammonium), 7.7 mg·L-1 phosphorus, 78.1 mg·L-1 potassium, 20.1 mg·L-1 calcium, 6.1 mg·L-1 magnesium, 8.0 mg·L-1 sulfur, and other micronutrients (Yamazaki, 1982). One week later, 26 uniform seedlings were selected and divided into two groups of 13 seedlings for transplantation into two separate DFT systems supplied with 3% NaCl solution. The planting interval was 13.0 cm, and the day and night temperatures were set to 21 ± 1 and 18 ± 1°C, respectively, and the light intensity of 173 ± 15 µmol·m-2·s-1 was provided by a fluorescent lamp (CREA1-FPL55EX-L, Iwasaki Electric Co., Tokyo, Japan). The lighting cycle was an 18-h photoperiod (day)/6-h dark period (night). A shading net was installed between the fluorescent lamp and the crops to equalize the intensity of the light by the location. The DFT system used in the experiment was 46.0 × 66.0 × 33.0 cm (L × W × H) with a capacity of 70.0 L of water. A cooler (Coolpipe 150LF; Taitec Co., Saitama, Japan) and heater (CTR-420; AGC Techno Glass Co., Chiba, Japan) were installed in the nutrient solution tank to maintain the water temperature at 22°C during the cultivation period, and microbubbles were generated throughout the experiment.
Microbubble Generation
Microbubbles were generated using a rotary type microbubble aerator (3.5-cm diameter × 7.0-cm long; M2-MS/SUS; Nanoplanet Research Institute Co., Yamaguchi, Japan) with a pump (PMD-1521B6E; Sanso Electric Co., Hyogo, Japan). Fig. 1 shows an overall schematic diagram of the microbubbles used in the experiment. Air is automatically introduced by the centrifugal force generated through the circulation flow meter, and a vortex of air is formed along the central axis. Air used for microbubbles was injected at a rate of 1.0 L·min-1 during the growing period.
Measurements
Electrical conductivity (EC), dissolved oxygen (DO), and pH were measured to determine the effect of microbubbles on the nutrient solution. The measurement interval was 2 days during the cultivation period using a DO electrode (9520-10D with a D25 reading unit; Horiba, Kyoto, Japan), an EC meter (B-173, Horiba, Kyoto, Japan), and a pH electrode (6377-10D with a D21 reading unit, Horiba, Kyoto, Japan). Fourteen days after transplanting, leaf length, leaf width, longest root length, and fresh weight of leaves and roots were measured. The leaves and roots were then left to dry in an air dryer (DX400; Yamato Scientific Co., Tokyo, Japan) for 4 days at 66°C, and the dry weight of the samples was measured.
Statistical Analysis
The experiment was repeated twice following a completely randomized design. For the growth survey, 10 samples were analyzed for each treatment, and analysis of variance (ANOVA) was performed using the SPSS 20 (SPSS 20, SPSS Inc., Chicago, IL, USA) program for statistical analysis of measurement data. Statistical analysis was performed using ANOVA followed by the t-test method (p < 0.05) to verify the significance between the means of each treatment.
Results and Discussion
Microbubble Analysis
pH, EC, and DO did not show significant differences in the initial and final values during the 2-week period. As a result of comparing the control group with the microbubble treatment, the pH values were 6.0 and 6.1 after 14 days (Fig. 2A). EC in the microbubble treatment was 0.4 dS·m-1 higher in the first 2 days and 0.3 dS·m-1 higher in the last 2 days compared to the control (Fig. 2B). DO levels were higher in microbubble conditions during the first 2 days and did not show significant differences over the last 12 days (Fig. 2C).
Changes in water characteristics when microbubbles are used in hydroponic cultivation have been evaluated differently in many reports. During hydroponic cultivation of lettuce, when microbubbles were intermittently generated in water (15, 30, 60 min/h), the pH decreased to 4.5 (Park et al., 2010), and in another experiment, microbubble treatment increased DO from 6.15 to 8.6 mg/L (Abu-Shahba et al., 2021). However, when microbubbles were generated during the cultivation of lettuce using the DFT system, the changes in pH, EC, and DO were not significant (Park and Kurata, 2009), showing the same trend as in this experiment. In watercress cultivation using microbubbles, EC and pH did not significantly differ during the cultivation period (Bok et al., 2019). During this experiment, it was expected that the microbubbles would have changed the pH, EC, and DO. However, contrary to expectations, there was no statistically significant change, and the reason was thought to be related to water quality. Generating microbubbles in high-salinity water or seawater reduce duration by suppressing bubble adhesion and reducing surface tension (Kawahara et al., 2009). Thus, it was determined that there is no difference in the DO measurement results because the holding time is shortened due to the decreased tension of the bubbles. Therefore, although the microbubbles were generated in artificial seawater conditions, it was judged that the water quality did not change significantly.
Plant Growth
We investigated the impact of microbubbles on lettuce grown in 3% NaCl seawater and found that microbubble-treated lettuce had better growth than controls. Leaf length and leaf width were 5% higher and showed no significant difference (Fig. 3A and 3B). Leaf number of the microbubble-treated samples was 19.8, i.e., 1.4 more leaves than that in the control (Fig. 3C). The longest root length was 6.9 cm longer in microbubble-treated samples than that in the control (Fig. 3D). The leaf fresh weight was 3.02 g heavier than that of the control (Fig. 4A). Microbubble-treated samples had 56% and 42% higher root fresh weight and leaf dry weight, respectively (Fig. 4B and 4C). Under microbubble conditions, the most significant difference was observed for the root dry weight, which was 226% higher (Fig. 4D).
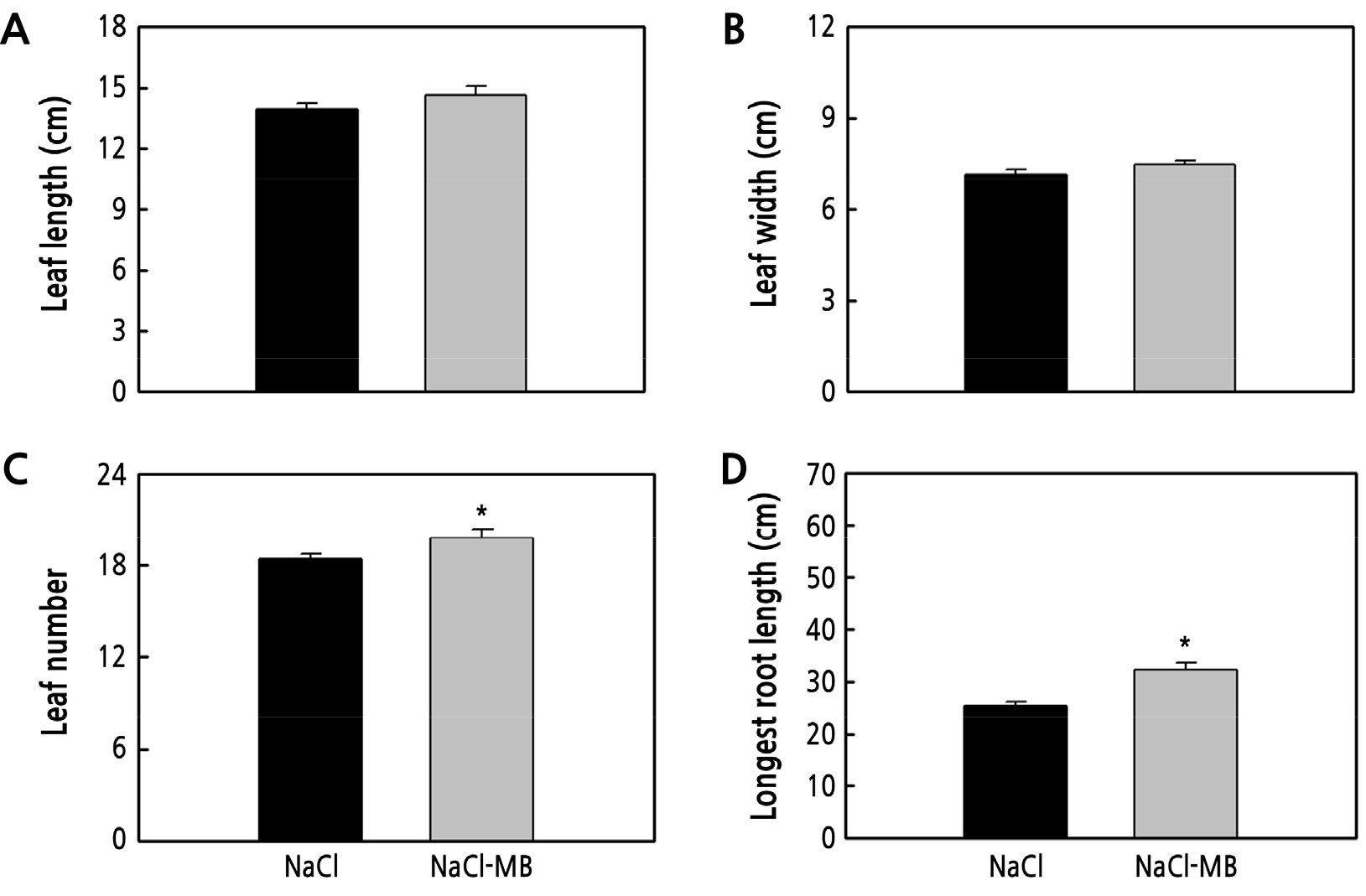
Fig. 3.
Effect of microbubbles on lettuce grown via hydroponic cultivation under high-saline conditions. Leaf length (A), leaf width (B), leaf number (C), and longest root length (D) of lettuce grown in the hydroponic system for 2 weeks. The data represent the means, and the vertical bars indicate standard errors (n = 10). Means with an asterisk are significantly different by t-test (p < 0.05).
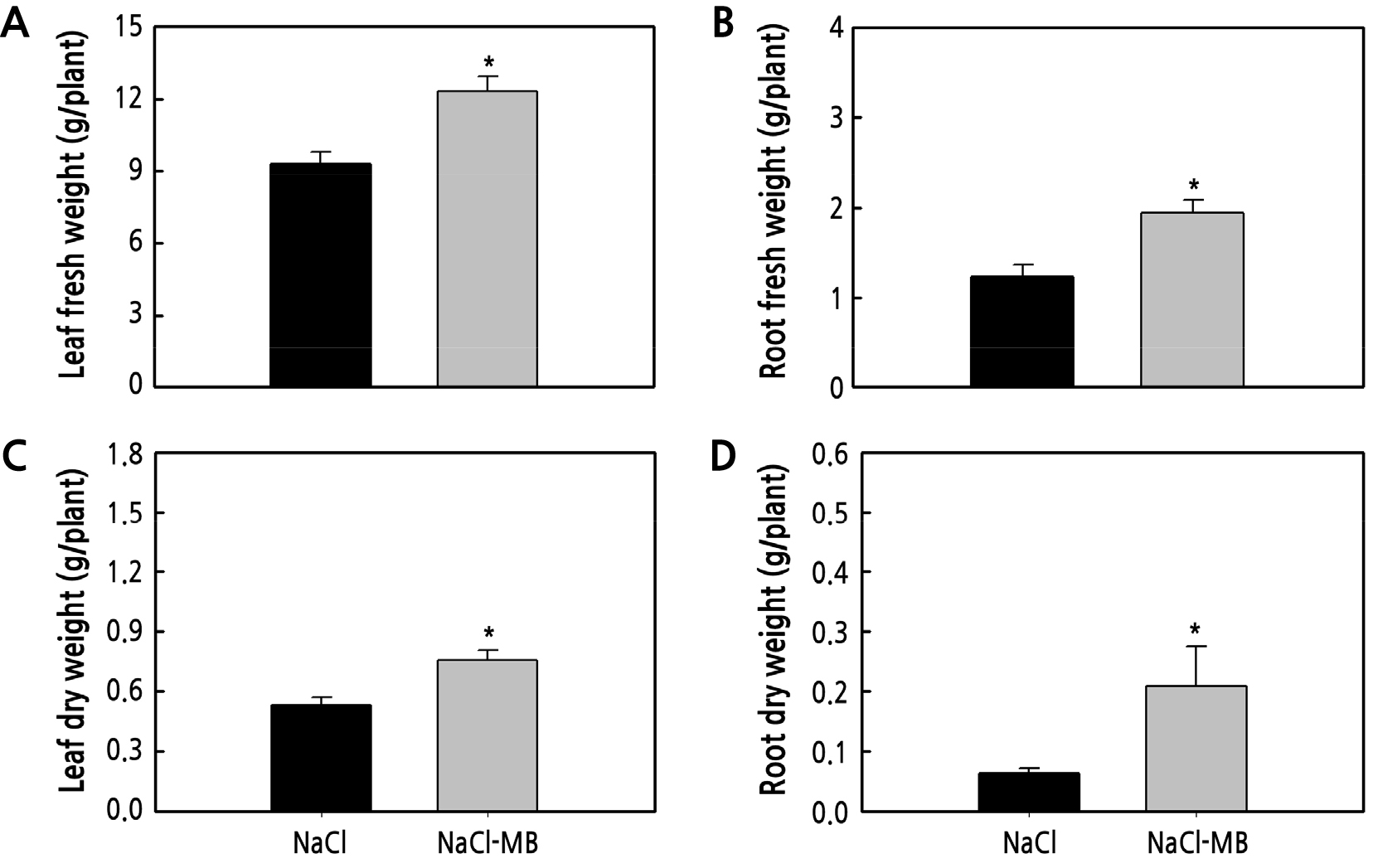
Fig. 4.
Effect of microbubbles on lettuce grown via hydroponic cultivation under high-saline conditions. Leaf fresh weight (A), root fresh weight (B), leaf dry weight (C), and root dry weight (D) of lettuce grown in the hydroponic system for 2 weeks. The data represent the means, and the vertical bars indicate standard errors (n = 10). Means with an asterisk are significantly different by t-test (p < 0.05).
The effect of NaCl on plant growth differs by plant species; however, in general, the effects are adverse. Although 50 mM NaCl treatment promoted the growth of lettuce, higher concentrations showed a growth inhibitory effect (Kim and Hyun, 2011); similar results were reported in Atriplex nummularia (de Araújo et al., 2006). In another study, long-term, low-concentration NaCl treatment reduced the growth and fruiting of crops such as tomatoes, peppers, and eggplants (Rhee et al., 2002). In addition, when the roots are exposed to salt stress, normal growth is difficult owing to the interference of NaCl on cell cycle activity, resulting in the inability of the roots to divide (West et al., 2004; Galvan-Ampudia et al., 2013). Also, NaCl stress decreased K and Ca content in roots and leaves as monovalent ions were released from the roots by Na absorption (Lynch and Lauchli, 1984; Cramer et al., 1985; Park et al., 2006). When Ca absorption in the root decreased, the growth point or Ca content in the leaves decreases, leading to deficiency symptoms (Bennett, 1993). Conversely, when Ca absorption increased, the fresh weight and dry weight increased (Choi and Park, 2008). In this study, microbubble treatment increased the growth and weight of the aboveground parts and roots because zeta potential was created on the surface of the microbubble, and the adsorption of cations on the bubble surface increased. This is thought to be because it facilitated the absorption of cations such as Ca and K (Takahashi, 2005; Park and Kurata, 2009). In fact, it was reported that microbubbles increased the absorption rate of K and Ca at pH 6.5 and that when the zeta potential was large, it helped the roots to absorb minerals (Minagawa et al., 2018). These data explain the reduced growth of lettuce in the control treatment.
Conclusion
This study showed that microbubbles attenuate salt stress in plants grown in high-salinity water without significant differences in pH, EC, and DO microbubbles in 3% NaCl solution. The fresh and dry weights of the roots and shoots significantly increased, suggesting the potential of microbubbles in enhancing plant growth in seawater-based coastal hydroponic cultivation. However, further microbubble experiments are required, especially using multiple NaCl concentrations.