Introduction
Materials and Methods
Stock Plant Conditions
Plant Culture Treatments and Propagation Environment
Rooting Assessment
Photosynthetic Characteristics Measurements
Statistical Analysis
Results and Discussion
Adventitious Root Development
Photosynthetic Characteristics of Hemp Cuttings with Different Leaf Numbers
Photosynthetic Characteristics of Hemp Under Various CO2 Concentrations
Conclusion
Introduction
In North America, cannabis (Cannabis sativa L.) production for legal markets, including the recreational and medicinal industries, is becoming profitable. North American spending on legal cannabis is estimated to reach $22.6 billion in 2021 (ArcView Market Research, 2017). The medicinal value of cannabis is mainly attributed to a group of secondary metabolites called cannabinoids, which are mostly concentrated in unfertilized female flowers (Potter, 2014). Nowadays, some countries including the United States, Canada, and the Netherlands have mitigated their legislations and established programs that permit the use of cannabis for medicinal purposes. In these programs, stringent safety criteria are enforced to manage the quality of cannabis administered to patients; however, growers are allowed a few manipulations for horticultural management (Caplan et al., 2017; Caplan et al., 2018). Currently, cannabis is mainly produced under controlled environments using a hydroponic system with artificial light (Caplan et al., 2017; Caplan et al., 2018). Hemp can be propagated by seeds (David, 2009), in vitro methods (Lata et al., 2009), and vegetative stem cuttings (Caplan et al., 2018). Hemp growers often prefer propagation by vegetative stem cuttings because they provide uniform plants with lower cost compared to propagation by seeds or in vitro methods (Caplan et al., 2018). The most important objective of propagation by vegetative stem cuttings is to promote adventitious roots (ARs) (Lebedev, 2019). Leaf number, cutting position on the plant, lighting, rooting hormones, water status, mineral nutrition, and rooting medium affect adventitious rooting in vegetative stem cuttings (Park et al., 2011; De Almeida et al., 2017; Park et al., 2017; Caplan et al., 2018). In this study, we focused on leaf numbers of hemp cuttings.
In some plant species, leaf retention on the stem cuttings is essential to ensure successful propagation (Hartmann and Kester, 1975; Park et al., 2011). Leaves are the major organ of photosynthesis for cuttings and are key to successful rooting. Increasing leaf number may enhance AR formation and rooting success rate in cuttings (Ofori et al., 1996; Caplan et al., 2018). Moreover, leaves can enhance rooting by serving as the source of endogenous auxin and rooting co-factors (Tombesi et al., 2015). In contrast, a larger leaf area and higher leaf number provide a greater surface area for evapotranspiration, which may negatively influence the rooting rate (Davis and Potter, 1989). The optimal leaf number on stem cuttings in lychee was four, although it varies among species (Alves et al., 2016).
Light intensity is the key regulator of photosynthesis, and it is involved at the photoreaction stage of photosynthesis by supplying sufficient electrons for dark responses (Zhou et al., 2012; Zhang et al., 2020). Plant responses to high CO2 concentrations are mediated by leaf photosynthesis, which is associated with changes in leaf structure, carbon balance, and chemical composition, depending on the plant species (Lee et al., 2001; Ainsworth et al., 2002; Zheng et al., 2019b). Photosynthetic characteristics at the rooting stage of cuttings have been examined in some plant species, such as poinsettia (Svenson et al., 1995), Acer rubrum (Smalley et al., 1991), and petunia (Klopotek et al., 2012), but not in hemp. Therefore, the objective of the present study was to explore the effects of leaf number on hemp cuttings, grown for medicinal purposes on AR generation and to understand the photosynthetic characteristics of this species.
Materials and Methods
Stock Plant Conditions
Hemp (Cannabis sativa L.) mother plants were cultivated in a greenhouse controlled at 25/18°C ± 2°C (day/night) with a pad and fan cooling system at North Carolina State University. The mother plants were grown in pots (approximately 30 L) containing a blended organic growing substrate and watered 3–5 times a day using an automated drip irrigation system with Hoagland nutrient solution (1.2 dS·m-1 electrical conductivity and 5.8 pH) for 8 months (Lam et al., 2020).
Plant Culture Treatments and Propagation Environment
Approximately 150 hemp cuttings with two (two fully expanded top leaves), three (three fully expanded top leaves), and four leaves (four fully expanded top leaves) were harvested from the apical regions of 5 mother plants, and 18 uniform cuttings per replication were selected (Fig. 1). We defined the leaf with a leaf length of three cm or more. We conducted two replications per treatment. The cuttings were dipped into 1% diluted soap water and then into a bactericide (ZeroTol 2.0, Biosafe System, LLC., East Hartford, CT, USA) diluted to 37% (0.5 ozone/1 gallon) for one minute for disinfection and transplanted in a plug tray (72 holes) filled with a horticultural substrate (Fafard 4P Mix, Sun Gro Horticulture Co. Ltd, Agawam, MA, USA). The trays were randomly placed in an indoor cultivation room controlled at 25°C ± 2°C average temperature with 95%, 90%, and 85% relative humidity in the first, second, and third weeks, respectively. Red and blue LEDs were installed for light irradiation at approximately 80 µmol·m-2·s-1 with a photoperiod of 16 h for 22 days after transplantation (DAT). Cuttings were watered with an ebb and flow system every 5 min for 22 DAT.
Rooting Assessment
The tray bottom was checked daily from 12 DAT onward for ARs, and cuttings were harvested at 22 DAT. The substrate was removed from the rooted cuttings by washing with water. The average length of ARs was determined with a measuring tape (STHT-36127, Stanley Electric Co., Ltd., Tianjin, China), and the average fresh weight of ARs was measured using an electronic scale (Si-234, Denver Instrument, Bohemia, NY, USA). The average AR number was also counted. The AR generation rate was calculated as the percentage of rooted cuttings in each treatment. All AR characteristics were determined at 22 DAT on eighteen cuttings per replication (n = 18).
Photosynthetic Characteristics Measurements
A portable photosynthesis system (LI-6800XT, LI-COR Biosciences Inc., Lincoln, NE, USA) was used to measure the net photosynthetic rate, stomatal conductance, and transpiration rate of cuttings. Five plants (n = 5) in each replication were used and the measurement was performed with two replications per treatment. The net photosynthetic rate of cuttings with two, three, and four leaves was measured at 22 DAT and that of well-rooted hemp plants was measured at 60 DAT. For measuring the net photosynthetic rate of cuttings with two, three, and four leaves at 22 DAT, the light intensity (photosynthetic photon flux density [PPFD]) was set at 0, 50, 100, 175, 250, 500, 750, 1,000, and 1,500 µmol·m-2·s-1; CO2 concentration was set at 400 µmol·mol-1, and chamber temperature was set at 25°C. In measurement 1 at 60 DAT, photosynthetic characteristics were measured at PPFD of 0, 50, 100, 175, 250, 500, 750, 1,000, and 1,500 µmol·m-2·s-1; chamber temperature of 25°C; and CO2 concentration of 400 µmol·mol-1. In measurement 2 at 60 DAT, photosynthetic characteristics were measured at CO2 concentrations of 50, 100, 200, 300, 400, 600, 800, 1,000, and 1,500 µmol·mol-1; PPFD of 1,500 µmol·m-2·s-1; and chamber temperature of 25°C.
Statistical Analysis
In this experiment, we conducted two replications by using completely randomized designs. The SPSS 20.0 program (SPSS 20, SPSS Inc., Chicago, IL, USA) was used for a one-way analysis of variance. Significant differences among the means of treatment groups at the 5% level were compared using Tukey’s multiple range test.
Results and Discussion
Adventitious Root Development
Adventitious root (AR) development in hemp cuttings was affected by the number of leaves. At 22 DAT, there was no significant difference among leaf number treatments; however, the two-leaf treatment showed higher values of average AR length, average AR fresh weight, AR generation rate, and average number of ARs compared with other treatments (Figs. 1 and 2). Two leaves per cutting likely reduced evapotranspiration; therefore, fewer leaves would enhance root growth because of reduced evapotranspiration. Previous studies have shown the effects of cutting size and leaf number as well as of cutting length × leaf area interaction on rooting (Tchoundjeu and Leakey, 1996; OuYang et al., 2015). The number of mature leaves on cuttings at the time of transplantation is important for the production of new leaves (Yamdagni and Sen, 1973). Root weight, root length, and roots number were affected by leaflets left on each cutting (Park et al., 2011). Leaves may directly affect shoot growth since the initial growth of shoots in seedlings depends on the carbon assimilation by mature leaves (Costa and Challa, 2002). Leaves on cuttings support CO2 assimilation throughout the rooting period (Smalley et al., 1991; Klopotek et al., 2012). At the initial stages of cutting growth, water uptake occurs partially at the base of the cut surface in the absence of ARs. The higher transpiration rate of cuttings with four leaves than that of cuttings with two leaves may lead to physiological disabilities (Newton et al., 1992). Typically, at the early stages of rooting, high humidity is maintained to inhibit transpiration and induce AR formation (De Almeida et al., 2017). Our results indicate that cuttings with two leaves are the most suitable for the adventitious rooting of hemp.
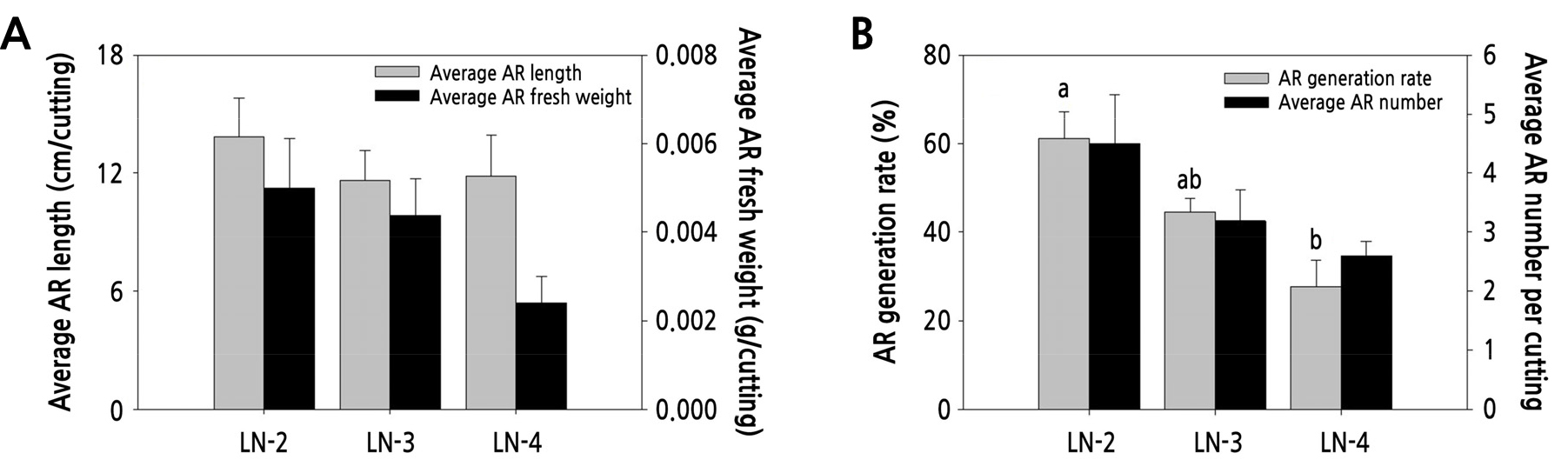
Fig. 2.
Average adventitious root (AR) length (A left), average AR fresh weight (A right), AR generation rate (B left), and average AR numbers (B right) in hemp cuttings with two (LN-2), three (LN-3), and four (LN-4) leaves. The cuttings were obtained from the apical regions of the mother plant. Values are presented as mean ± SE (n = 18).
Photosynthetic Characteristics of Hemp Cuttings with Different Leaf Numbers
The net photosynthesis rate of hemp cuttings with different leaf numbers was measured under various light intensities and a constant CO2 concentration and temperature (400 µmol·mol-1 and 25°C, respectively) (Fig. 3). There were significant differences in the net photosynthetic rate of cuttings with different leaf numbers. Cuttings with two leaves showed the highest net photosynthetic rate probably because of reduced water loss from fewer leaves. Although cuttings with two and three leaves showed similar responses to different light intensities, the two-leaf cuttings achieved a higher net photosynthetic rate than the three-leaf cuttings. These results indicate that cuttings with two leaves were more efficient in initial AR formation and carbohydrate supply to these ARs than cuttings with three leaves. At 22 DAT, the net photosynthetic rate was the highest in cuttings with two leaves probably due to earlier AR development.
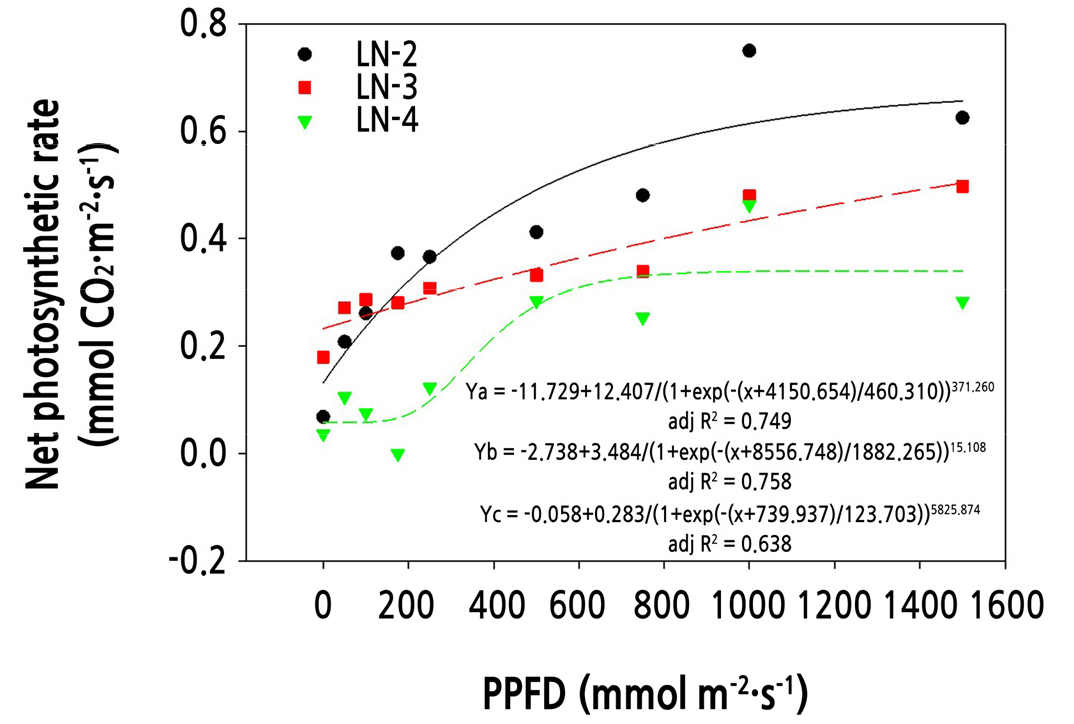
Fig. 3.
Regression analyses of mean photosynthesis rate on two (LN-2), three (LN-3), and four (LN-4) leaves for cuttings at 22 days after transplantation under different light intensities (photosynthetic photon flux density, PPFD) (0, 50, 100, 175, 250, 500, 750, 1,000, and 1,500 µmol·m-2·s-1). The solid, long dashed, and short dashed lines in the graph represent the quadratic regression for two (LN-2), three (LN-3), and four (LN-4) leaves and the relation equation is given as Ya, Yb, and Yc, respectively.
Photosynthetic characteristics of hemp were observed under various light intensities and influenced by changing the amount of light intensity. The received light is weak when the light intensity is low; therefore, the plant will create more photosynthetic pigments, especially chlorophyll (Chl) b, resulting in a decrease of the Chl a/b ratio as well as a decrease of the photosynthetic characteristics. At 60 DAT, the net photosynthetic rate of hemp markedly increased with increasing light intensity from 0 to 750 µmol·m-2·s-1. However, the net photosynthetic rate became saturated from 760 to 1,500 µmol·m-2·s-1 (Fig. 4A). The stomatal conductance of cuttings increased with increasing light intensity from 0 to 250 µmol·m-2·s-1 and then decreased with increasing light intensity from 300 to 1,500 µmol·m-2·s-1 (Fig. 4B). Likewise, the transpiration rate of hemp plants increased with increasing light intensity from 0 to 250 µmol·m-2·s-1 and then decreased with increasing light intensity from 300 to 1,500 µmol·m-2·s-1 (Fig. 4C). These results corroborate the findings reported by Zheng et al. (2019b); the authors found that the net photosynthetic rate markedly increased from 0 to 600 µmol·m-2·s-1 but remained stable from 800 and 1,800 µmol·m-2·s-1 (Zheng et al., 2019a). Thus, based on the net photosynthetic rate, it is economical to cultivate rooted hemp plants at a light intensity of ~600 µmol·m-2·s-1. Well-rooted hemp cuttings are adversely affected by high light intensity because of increased leaf transpiration and temperature (Zobolo, 2010). Leaf photosynthetic activity rapidly increases with increasing light intensity from 0 to 500 µmol·m-2·s-1, probably due to an increase in the levels of non-structural carbohydrates (Tombesi et al., 2015). Light intensity levels below the saturation peak can promote the photosynthetic activity of cuttings; however, extreme light regimes can enhance the air vapor pressure deficit, resulting in leaf desiccation (Tombesi et al., 2015; Zheng et al., 2019a). Until the saturation of the net photosynthetic rate, the stomatal conductance, and transpiration rate tend to increase. However, when the photosynthetic saturation point is reached, the plants gradually close the stomata to control transpiration while maintaining the photosynthetic saturation state and accelerating the CO2 exchange rate. The light saturation point of hemp is not as high as that of other horticultural crops (Xin et al., 2019); therefore, a light intensity over 400 µmol·m-2·s-1 should be set to promote hemp rooting in an indoor cultivation system.
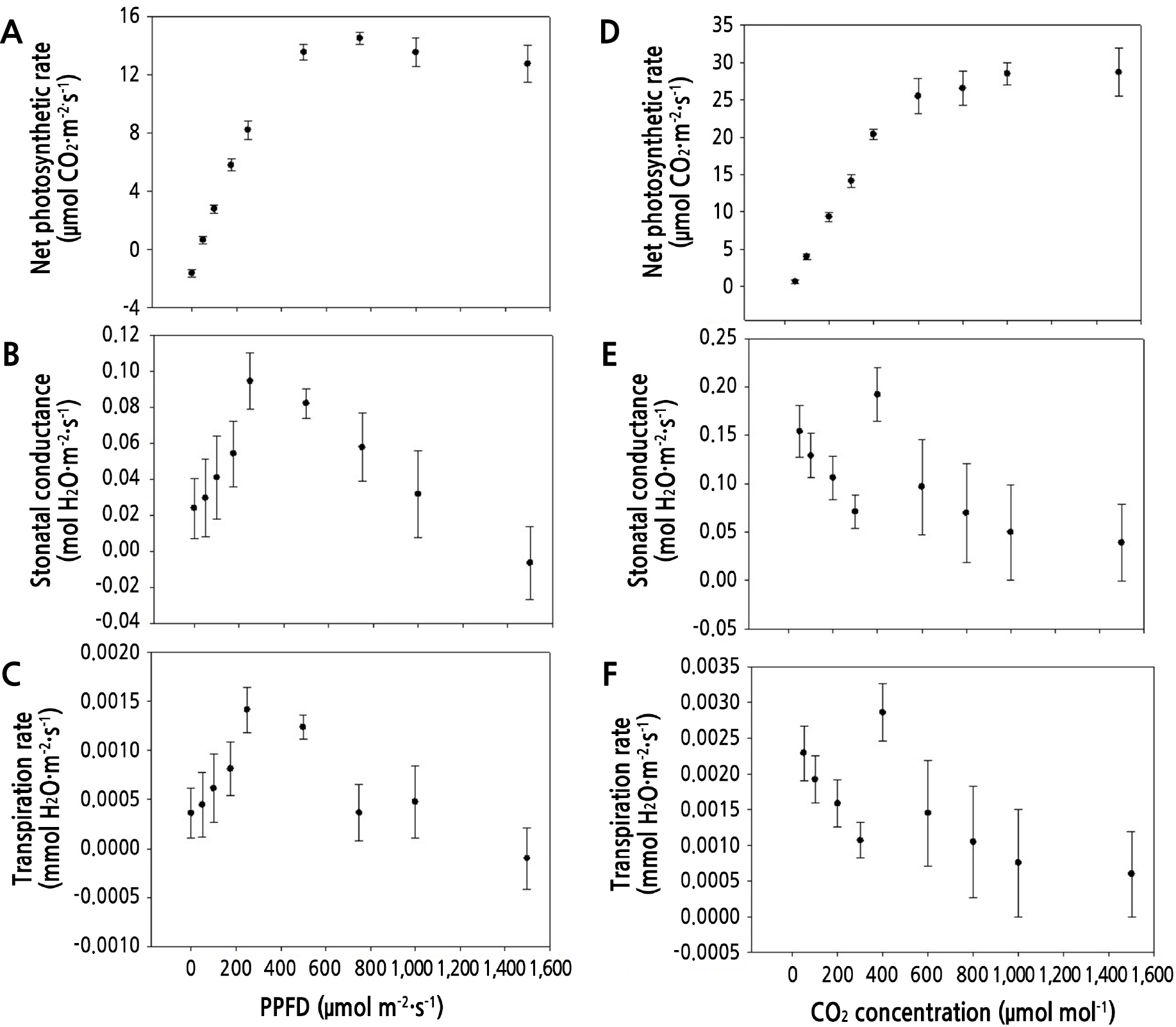
Fig. 4.
Net photosynthetic rate (A and D), stomatal conductance (B and E), and transpiration rate (C and F) of hemp at 60 days after transplantation under different light intensities (0, 50, 100, 175, 250, 500, 750, 1,000, and 1,500 µmol·m-2·s-1) and different CO2 concentrations (300, 200, 100, 50, 400, 600, 800, 1,000, and 1,500 µmol·mol-1). Values are presented as mean ± SE (n = 5).
Photosynthetic Characteristics of Hemp Under Various CO2 Concentrations
The net photosynthetic rate of cuttings increased markedly with increasing CO2 concentration from 50 to 600 µmol·mol-1 and became saturated above the concentration of 600 µmol·mol-1 (Fig. 4D). The stomatal conductance and transpiration rate increased in the order of initial CO2 concentrations of 300, 200, 100, 50, and 400 µmol·mol-1 and then gradually decreased from 600 to 1,500 µmol·mol-1 (Fig. 4E and 4F). When the CO2 concentration increases, the stomata close, and when the CO2 concentration decreases, the stomata are ordinarily open. To analyze the gas exchange characteristics according to the CO2 concentration, the order of the CO2 setpoints was 300, 200, 100, 50, 400, 600, 800, 1,000, and 1,500 µmol·mol-1. This is to confirm the pattern of increasing the stomatal conductance with decreasing the CO2 concentration (from 300 to 50), and to confirm the tendency of the stomatal conductance to decrease as the CO2 concentration increased under the condition of 400–1,500 µmol·mol-1. Moreover, the CO2 saturation point was determined to be 600 µmol·mol-1 in Fig. 4; however, the stomatal conductance reached the maximum value at 400 µmol·mol-1 CO2 concentration point before reaching the CO2 saturation point. These results indicate that elevated CO2 levels increased the net photosynthetic rate of plants, particularly C3 species (Singh and Reddy, 2016; Zheng et al., 2019b). The photosynthetic rate of the lettuce at 4, 7, 14, 21, and 28 days after transplanting was increased with increasing CO2 concentration from 100 to 600 µmol·mol-1 (Jung et al., 2016). This increase in photosynthesis under elevated CO2 concentrations may reduce dark respiration and photorespiration and enhance carboxylation efficiency (Duarte et al., 2013). Elevated CO2 levels may increase photosynthetic carbon assimilation and potentially improve plant growth and productivity. However, the net photosynthetic rate remained unchanged when the plants were grown under long-term high CO2 concentrations (Casteel et al., 2008; Robredo et al., 2010). In soybean, leaf photosynthesis was reduced with increasing CO2 concentrations from 400 to 1,000 µmol·mol-1 (Kanemoto et al., 2009). This reduction in the net photosynthetic rate under high CO2 concentrations may be attributed to a decline in Rubisco activity or carbohydrate accumulation in leaves (Jifon and Wolfe, 2002; Davey et al., 2006; Zheng et al., 2019b). Moreover, the suppression of photosynthesis at high CO2 concentrations may be caused by reduced stomatal conductance and respiration (Hamilton et al., 2001; Wang et al., 2013). Leaf biochemical characteristics and structure may play important roles in plant responses to elevated CO2 concentrations. Typically, high CO2 concentrations increase leaf thickness and mesophyll size because of elevated carbohydrate substrate availability, which is related to leaf photosynthetic rate (Tissue and Lewis, 2010; Zheng et al., 2019b). In addition, high CO2 concentrations might alter leaf biochemical composition, including the concentration of nitrogen and non-structural carbohydrates, which play essential roles in plant responses to elevated CO2 (Xu et al., 2012). Therefore, to achieve the maximum net photosynthetic rate of hemp, the CO2 concentration should be set to ~600 µmol·mol-1 with other suitable environmental conditions in an indoor cultivation system.
Conclusion
The present study demonstrated that to obtain the maximum adventitious rooting in hemp cuttings derived from the apical region of the mother plant, the cuttings should bear two expanded leaves. Moreover, to achieve the maximum net photosynthetic rate in hemp, a PPFD of 500 to 750 µmol·m-2·s-1 and a CO2 concentration of 600 µmol·mol-1 may be the most suitable settings in an indoor cultivation system.