Introduction
Materials and Methods
Plant Materials and Virus-free Shoot Induction
In vitro Rooting of Shoots and Analysis of Growth Characteristics
Qualitative and Quantitative Analysis of Phenolics by HPLC
Statistical Analysis
Results
Rooting of in vitro Shoots
Phenolic Content in the Shoots
Discussion
Conclusions
Introduction
Woody plant species are generally considered recalcitrant for in vitro micropropagation (Thorpe, 2006). Micropropagation of woody plant species is largely through the proliferation of axillary shoots from axillary buds or multiple shoots from shoot tips, followed by rooting of elongated shoots generally in a low salt medium with auxin. Success in micropropagation is thus dependent on the growth of good quality adventitious roots from regenerated shoots (Hou et al., 2010). Adventitious root regeneration from shoots involves three distinct phases: induction, initiation, and development (De Klerk et al., 1999). Therefore, numerous studies have focused on the control mechanism of adventitious root regeneration from shoots developed in vitro using various root promoting substances (De Klerk et al., 2011; Jeong et al., 2021).
Plant growth regulators, such as auxins, play a crucial role in the regeneration and development of adventitious roots from shoots of different genotypes of apple (Malus domestica Borkh.) (Lei et al., 2018; Texeira et al., 2019; Tahir et al., 2022). Several studies have used auxins such as indole-3-acetic acid (IAA), indole-3-butyric acid (IBA), and naphthalene acetic acid (NAA) (Texeira et al., 2019). In some cases, the combination of IBA and NAA was most effective (Modgil et al., 2017; Modgil and Thakur, 2017). Modgil et al. (2010) achieved a higher rooting percentage using IBA (–82%) than using NAA (–71%) in apple rootstock EMLA111. The success of rooting in apple shoots also depends on the genotype, for example, in ‘Jarka’ and ‘Mividbe’, IBA was not an effective auxin to induce roots (8% and 1%, respectively). However, a combination of NAA (1.0 mg·L-1) and IAA (1.0 mg·L-1) induced 44% and 22% rooting in ‘Jarka’ and ‘Mividbe, respectively, as reported by Paprstein and Sedlak (2015). Regeneration of adventitious roots from shoots remains to be problematic for in vitro apple plantlet production (De Klerk et al., 2011; Kim et al., 2020a). Consequently, several attempts have been directed toward the choice and combination of growth regulators, root induction procedure, one- or multiple-step culturing, and treatment duration (De Klerk et al., 2011; Kim et al., 2020a; Souza et al., 2022). Previously, the effects of exogenous auxin on in vitro rooting of apples, which were difficult to root due to physiological or genetic reasons, were examined. Excessive auxin improved the rooting rate; however, it led to the formation of a callus and did not result in further rooting (Data not shown).
Phenolic compounds are wounding-related substances that promote adventitious root induction (De Klerk et al., 1999). Several studies show that phenolics protect auxins from decarboxylation; after the application of phenolics, more auxin is available to induce roots (Wilson and Van Staden, 1990). A positive correlation between phenolic content and root formation has been reported in many woody species including cherry, chestnut, and olive (Trobec et al., 2005; Osterc et al., 2008). However, certain phenolics were reported to inhibit the process of rooting by stimulating IAA oxidation or promoting IAA decarboxylation (Bandurski et al., 1995). It has been shown that the phenolics pool varies in composition and amount between species and even cultivars and is also influenced by developmental stages and environmental factors (De Klerk et al.,1999; Osterc et al., 2007; Denxa et al., 2012). De Klerk et al. (1999) studied the effects of exogenously applied phenolics on adventitious root formation and oxidative decarboxylation of indole acetic acid in M. domestica ‘Jork 9’. However, studies on endogenous levels of phenolics and their role in induction of in vitro adventitious roots from apple rootstocks and scions have not been performed.
Therefore, this study aimed to analyze and quantify the endogenous phenolic compounds present in shoots of easy-to-root rootstocks (M.9 and M.26) and difficult-to-root cultivars (‘Hongro’ and ‘Fuji’; scions) and determine their role in the rooting of in vitro-cultured apples to produce virus-free apple plants.
Materials and Methods
Plant Materials and Virus-free Shoot Induction
Nodal explants with axillary buds were collected in spring 2020 (from March to May) from 5-year-old mature trees of Malus domestica Borkh. (rootstocks: M.9 and M.26; cultivars: ‘Hongro’ (HR) and ‘Fuji’ (FJ), scions) established in an experimental field station in Chungcheongbuk-do, Republic of Korea. The excised nodal explants (–1 cm) were washed under running tap water and then surface sterilized in 70% alcohol for several seconds. Then, explants were disinfected with different concentrations of sodium hypochlorite (10%) for 10 min. The sterilized single nodal explants were inoculated into test tubes (200 × 24 mm2) with 15 mL of nutritive solution. The medium contained the mineral salts of Murashige and Skoog (1962) medium (MS, 1962) supplemented with 1.1 µM 6-benzyl amino purine (BAP) for inducing, sprouting, and shoot differentiation, enriched with 30 g·L-1 sucrose and gelled with 8.0 g·L-1 agar, 0.4 mg·L-1 thiamine hydrochloride, and 100 mg·L-1 of inositol. The pH was adjusted to 5.8 ± 0.1. Cultures were maintained at 24 ± 1°C under a 16-h light/8-h dark photoperiod using white light-emitting diodes (LEDs) at an intensity of 88.0 µmol·m-2·s-1 PPFD. Shoot tips (3 cm in height) obtained from in vitro differentiated shoots were transferred to MS medium supplemented with 2.2 µM BAP for induction of multiple shoots according to the methods of Kim et al. (2020b). Shoots were grown and sub-cultured at 4-week intervals. The 4 clones of apple shoots were cultured at 32°C and sub-cultured every four weeks for three months to eliminate any virus present. After checking whether the viruses were removed from the shoots at the Korea Seed & Variety Service, only the individuals confirmed to be virus-free were propagated and used in this experiment.
In vitro Rooting of Shoots and Analysis of Growth Characteristics
The shoots were cultured in MS medium supplemented with 2.45 µM IBA, 30 g·L-1 sucrose, and 9.0 g·L-1 agar for induction of roots. Apple shoots (3 cm in height) were cultured in 400-mL glass bottles containing 80 mL of medium. Five shoots were cultured in each culture vessel. Shoot cultures were maintained at 24 ± 1°C under a 16-h light/8-h dark photoperiod using white LEDs at an intensity of 88.0 µmol·m-2·s-1 PPFD for 40 days. The percentage of shoots involved in rooting and callusing, number of roots, fresh weight (FW), dry weight (DW), plant height, number of leaves and number of nodes were measured every 10 days of culture. The FW of individual shoots was measured after washing the explants with sterile distilled water, and the DW of shoots was recorded after drying to a constant weight by freeze-drying (Alph1-4 dryer, Christ, Osterode, Germany) for 3 days.
Qualitative and Quantitative Analysis of Phenolics by HPLC
The plant material (shoots) was harvested every 10 days, and the basal parts (1-cm-long stem portion) and rest of the shoots were separated and used for analysis. The shoot parts were lyophilized in a freeze dryer, ground into powder, and stored in a freezer (–60°C) until analysis. The plant samples (100 mg) were triple extracted in 80% methanol. The residue was dissolved in methanol and filtered through Whatman filter paper (0.2 µm). The samples were analyzed on a high-performance liquid chromatography system (HPLC; 2690 Separation Module, Waters, Milford, USA) using a C18 column (5 µL, 150 × 4.6 mm). Acetonitrile (A) and 0.1% acetic acid (B) (v/v) were used as mobile phases with linear gradients of 8% A at zero min, 10% A at 2 min, 30% A at 27 min, 90% A at 50 min, 100% A at 51–60 min, and 8% A at 70 min. The sample aliquots were 20 µL each and the flow rate was 1.0 mL·min-1. Calibration and quantification of phenolics were carried out using standard phenolic compounds (ChromaDex, Irvine, USA).
Statistical Analysis
All experiments were replicated at least three times using a completely randomized design. For rooting experiments, 30 shoots (5 shoots × 5 vessels) were used in each treatment. The final data are the means of the two replicated experiments. The data were subjected to a two-factor analysis of variance (ANOVA). Significant differences between means were determined by Duncan’s tests at p = 0.05.
Results
Rooting of in vitro Shoots
The M.9 and M.26 shoots grew and attained a FW of 129.93 mg/plantlet and 219.37 mg/plantlet, respectively (Table 1). The DW of M.9 and M.26 shoots increased gradually and reached 21.66 mg/plantlet and 39.88 mg/plantlet, respectively. The height of the explants, number of leaves, and number of nodes also increased during the 40-day culture (Table 1). The in vitro shoots of HR and FJ also showed growth of shoots, with the FW reaching 292.12 mg/plantlet and 105.02 mg/plantlet, respectively. There was also an increase in the DW, height of plantlets, number of leaves, and number of nodes for HR and FJ over the culture period (Table 1). The percentage of shoots involved in rooting were 6.67% and 16.67% in M.9 and M.26, respectively, after 10 days of culture (Fig. 1); and the percentage increased to 64.44% and 80.00%, respectively, after 40 days of culture (Fig. 1). The number of roots formed were 4.39 and 6.45 per shoot in M.9 and M.26, respectively (Figs. 1 and 2). The percentages of rooting were 12.50% and 8.33% in HR and FJ, respectively (Figs. 1 and 3), whereas the number of roots were 1.00 in HR and 1.28 in FJ (Fig. 1).
Table 1.
Growth characteristics of easy-to-root rootstocks (ER; M.9, M.26) and difficult-to-root cultivars (DR; Hongro, Fuji) of Malus domestica during 40 days of in vitro culture
Cultivar / Rootstock | Day |
Fresh weight (mg) |
Dry weight (mg) |
Height (mm) |
No. of leaves (per plantlet) |
No. of nodes (per plantlet) | |
ER | M.9 | 0 | 50.23 ± 0.32 iz | 8.37 ± 0.05 j | 24.90 ± 0.17 fgh | 6.38 ± 0.14 ij | 5.72 ± 0.20 gh |
10 | 50.38 ± 1.53 i | 8.40 ± 0.25 j | 25.13 ± 0.04 fg | 6.25 ± 0.08 j | 5.65 ± 0.24 gh | ||
20 | 69.87 ± 6.39 hi | 11.64 ± 1.06 hij | 25.86 ± 0.12 ef | 6.87 ± 0.13 fghi | 5.58 ± 0.22 h | ||
30 | 94.60 ± 6.48 fg | 15.77 ± 1.08 fg | 26.25 ± 0.18 ef | 7.62 ± 0.14 cde | 5.71 ± 0.12 gh | ||
40 | 129.93 ± 4.61 e | 21.66 ± 0.77 e | 27.41 ± 0.77 ed | 7.33 ± 0.12 def | 5.64 ± 0.27 gh | ||
M.26 | 0 | 52.79 ± 2.73 i | 9.60 ± 0.50 ij | 28.21 ± 0.43 cd | 7.85 ± 0.04 abcd | 5.71 ± 0.08 gh | |
10 | 74.50 ± 2.37 ghi | 13.55 ± 0.43 hi | 28.40 ± 0.19 cd | 7.75 ± 0.13 bcd | 5.85 ± 0.08 fgh | ||
20 | 86.60 ± 4.18 fgh | 15.75 ± 0.76 gh | 28.67 ± 0.29 bcd | 7.88 ± 0.04 abcd | 5.95 ± 0.10 fgh | ||
30 | 155.45 ± 10.52 d | 28.26 ± 1.91 d | 39.06 ± 0.54 bcd | 8.27 ± 0.16 ab | 6.57 ± 0.03 de | ||
40 | 219.37 ± 15.24 b | 39.88 ± 2.77 b | 30.33 ± 0.50 ab | 8.30 ± 0.10 ab | 6.42 ± 0.04 def | ||
DR | Hongro | 0 | 193.58 ± 6.32 c | 33.38 ± 1.09 c | 28.56 ± 1.42 bcd | 6.50 ± 0.44 hij | 6.21 ± 0.22 efg |
10 | 195.33 ± 15.94 c | 33.68 ± 2.75 c | 28.85 ± 0.55 bcd | 7.08 ± 0.33 efgh | 6.28 ± 0.19 efg | ||
20 | 234.79 ± 8.21 b | 40.48 ± 1.41 b | 28.80 ± 0.95 bcd | 6.94 ± 0.22 fghi | 6.88 ± 0.26 cd | ||
30 | 272.79 ± 6.94 a | 47.03 ± 1.20 a | 29.91 ± 0.68 bc | 8.15 ± 0.34 abc | 7.00 ± 0.51 cd | ||
40 | 291.17 ± 12.94 a | 50.20 ± 2.23 a | 31.58 ± 0.53 a | 8.41 ± 0.20 a | 7.29 ± 0.15 bc | ||
Fuji | 0 | 54.86 ± 3.18 i | 10.16 ± 0.59 ij | 21.13 ± 0.33 j | 6.37 ± 0.01 ij | 6.95 ± 0.07 cd | |
10 | 78.89 ± 2.91 gh | 14.61 ± 0.54 h | 22.47 ± 0.16 ij | 6.59 ± 0.04 ghij | 7.40 ± 0.15 abc | ||
20 | 87.85 ± 9.85 fgh | 15.79 ± 0.82 gh | 23.57 ± 0.33 ij | 7.11 ± 0.04 efg | 7.79 ± 0.06 ab | ||
30 | 98.83 ± 0.77 fg | 18.30 ± 0.14 fg | 22.57 ± 0.63 ghi | 7.12 ± 0.02 efg | 7.77 ± 0.06 ab | ||
40 | 105.02 ± 1.39 f | 19.45 ± 0.26 de | 23.41 ± 0.73 hi | 7.03 ± 0.19 efgh | 7.95 ± 0.06 a |
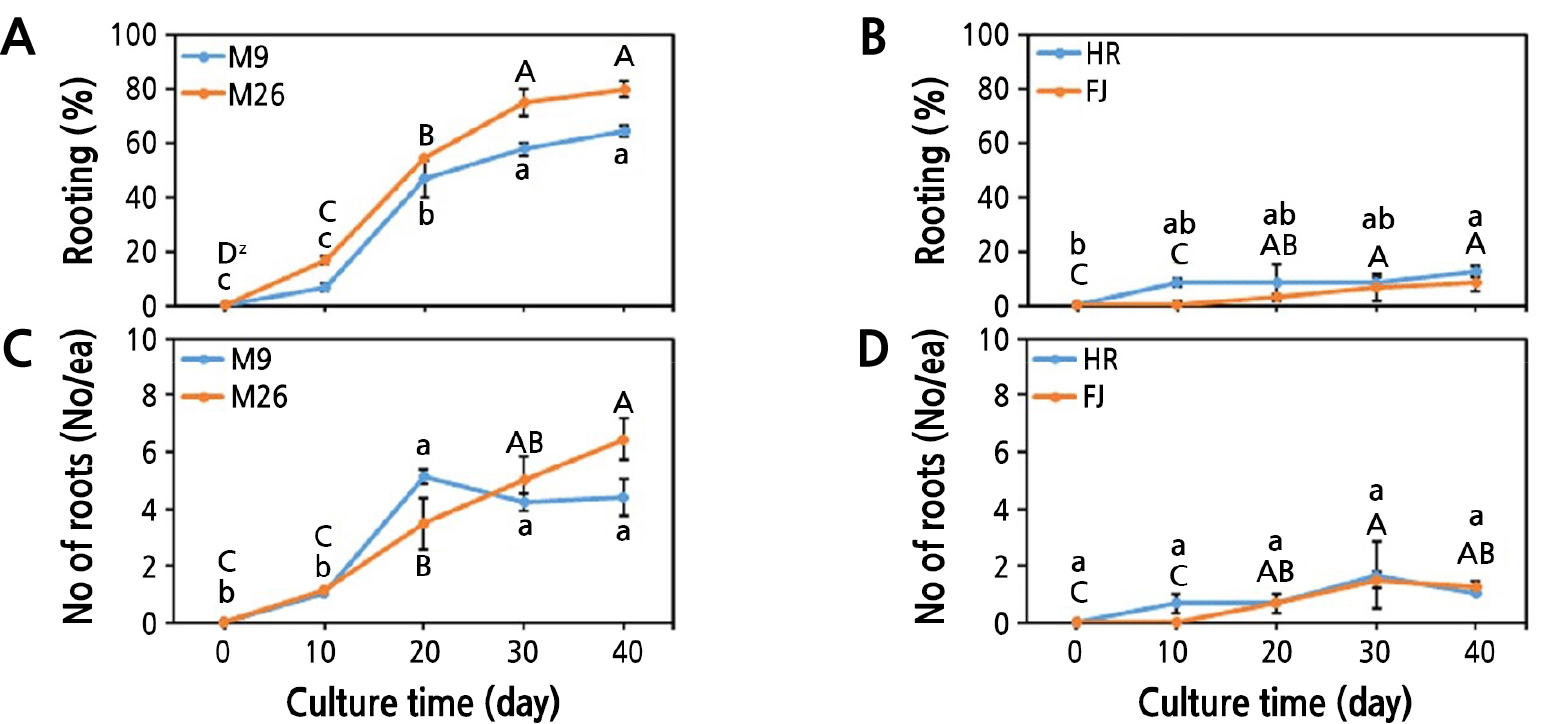
Fig. 1.
Rooting characteristics of Malus domestica in rooting medium. Root formation rate (A, C) and average root number (B, D) of two cultivars and two rootstocks. Different letters indicate significant differences within cultivars/rootstocks at p < 0.05 according to Duncan’s multiple range test. M.26 and ‘Hongro’ (HR) are written in uppercase letters, and M.9 and ‘Fuji’ (FJ) are written in lowercase letters.
Phenolic Content in the Shoots
The results of the analysis of phenolics in M. domestica rootstocks and scions cultured in vitro showed the presence of large quantities of phenolic acids (Figs. 4 and 5). Phloretic acid, coumaric acid, salicylic acid, chlorogenic acid, catechin hydrate, hesperetin, rutin, quercetin, and biochanin were the various phenolics detected in the M. domestica shoots. During the rooting period, in the easy-to-root rootstocks (M.9 and M.26), there were fluctuations in phloretic acid and coumaric acid contents in the shoots (Fig. 4). The content of salicylic acid decreased significantly over the culture period in the M.9 shoots; the content of salicylic acid was 665.43 µg·g-1 DW after 10 days of culture and it decreased to 221.81 µg·g-1 DW after 40 days of culture (Fig. 4). A similar decreasing trend was evident in the content of salicylic acid in the basal part of M.26 shoots (Fig. 4). However, the content of salicylic acid increased and showed the highest content at the end of the rooting time (40th day) in the HR shoots. Similarly, the content of salicylic acid increased from 318.33 µg·g-1 DW (after 10 days of culture) to 407.92 µg·g-1 DW (after 30 days) in FJ shoots (Fig. 4); a similar trend was observed in the basal part of FJ (data not shown).
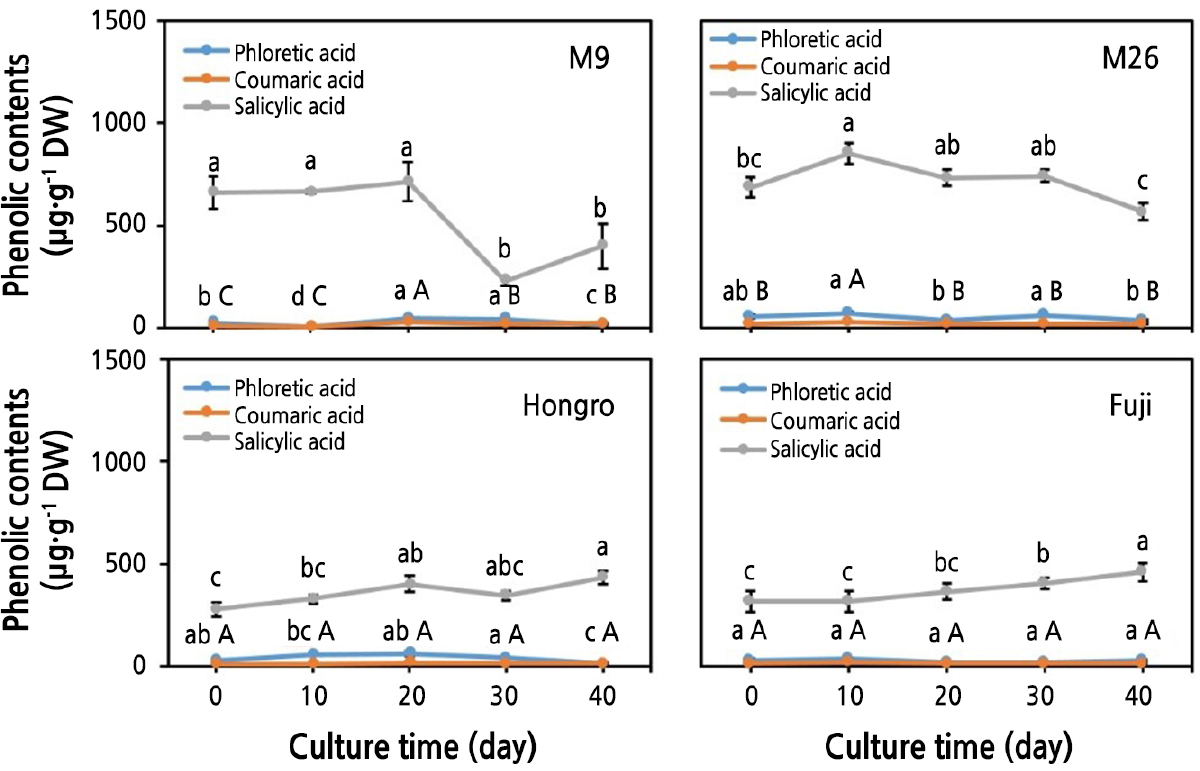
Fig. 4.
Changes in monophenol contents in shoots of Malus domestica during 40 days of in vitro culture. Easy-to-root rootstocks: M.9 and M.26. Difficult-to-root cultivars: ‘Hongro’ and ‘Fuji’. Different letters indicate significant differences among times at p < 0.05 according to Duncan’s multiple range test. Coumaric acid is written in uppercase letters, and phloretic acid and salicylic acid are written in lowercase letters.
The content of chlorogenic acid increased rapidly from 61.09 µg·g-1 DW (after 10 days) to 224.54 µg·g-1 DW (after 30 days) in the M.9 shoots. A similar increasing trend in the contents of chlorogenic acid was evident in M.26 (Fig. 5). The catechin hydrate levels varied from 578.24 µg·g-1 DW (on the 10th day) to 788.15 µg·g-1 DW (30th day) in the shoots of M.9, whereas the levels increased from 588.89 µg·g-1 DW (10th day) to 679.15 µg·g-1 DW (30th day) in the basal parts of M.9 shoots (data not shown). The content of catechin hydrate was 631.30 µg·g-1 DW on the 10th day and was reduced to 414.81 µg·g-1 DW on the 30th day in the upper part of M.26 shoots (Fig. 5). The content of rutin was 72.09 µg·g-1 DW on day 10 and 271.28 on day 30 in M.9 shoots (Fig. 5). A similar trend was observed in M.26 (Fig. 5). The levels of quercetin, hesperetin and biochanin were very low in both M.9 and M.26 shoots (Fig. 5).
The content of chlorogenic acid was 119.67 µg·g-1 DW on day 10 and increased to 334.20 µg·g-1 DW on day 30 in HR shoots (Fig. 5). The content of chlorogenic acid in the FJ shoots was comparatively lower (i.e., 146.97 µg·g-1 DW on the 10th day) and remained steady until the 30th day (Fig. 5). A similar trend was observed in the basal parts of FJ shoots (data not shown).
The content of catechin hydrate was 201.51 µg·g-1 DW on day 10 and was reduced to 159.79 µg·g-1 DW on day 30 in HR shoots (Fig. 5). The levels of catechin hydrate showed a similar trend in the basal parts of HR shoots (Fig. 5). The FJ shoots had comparatively higher levels of catechin hydrate (i.e., 515.26 µg·g-1 DW on day 10), which reduced to 260.56 µg·g-1 DW on day 30 (Fig. 5). A similar trend was observed in the basal parts of FJ shoots (data not shown). Other phenolics, such as rutin, quercetin, hesperetin, and biochanin, were present in low contents in both HR and FJ shoots.
Discussion
Micropropagation of virus-free apple (Malus domestica Borkh.) cultivars is achieved by in vitro cultures. However, rooting of in vitro shoots is still very problematic in some M. domestica cultivars. Several factors, such as light, temperature, auxins, phenolics, and their combination, have been tested to improve the induction and growth of adventitious roots from in vitro-raised apple shoots (Zimmerman, 1984). Phenolic compounds are known to enhance the rooting of shoots in many woody species, and researchers believe that phenolic compounds may act by controlling auxin oxidase-peroxidase activity (Gasper et al., 1992), thus promoting or inhibiting in vitro rooting. De Klerk et al. (2011) studied the effects of phenolic compounds on adventitious root formation and oxidative decarboxylation of exogenously supplied IAA in M. domestica ‘Jork 9’ using stem slices. They tested ortho-diphenols, paraphenols, and triphenols along with IAA on stem slices and reported the promotive effect of ferulic acid (a methylated ortho-diphenol); it increased the number of adventitious roots from 0.9 to 5.8. They also demonstrated that decarboxylation of IAA occurs in the presence of a monophenolic compound, namely salicylic acid. The above studies assessed the role of exogenous application of phenolic compounds. However, the role of endogenous phenolic compounds in the presence of exogenous auxin has not been studied so far.
In the current study, we used two rootstocks and two cultivars of M. domestica Borkh., namely M.9 and M.26 (apple rootstocks which are easy-to-root), and HR and FJ (apple cultivars which are difficult-to-root). We assessed various endogenous phenolic compounds from the shoots and verified their rooting efficiency in the presence of IBA. Analysis of endogenous phenolic compounds of in vitro-cultured apple shoots (M.9, M.26, HR, and FJ) revealed that phloretic acid, coumaric acid, salicylic acid, chlorogenic acid, catechin hydrate, hesperetin, rutin, quercetin, and biochanin were evident in all the cultivars and rootstocks. However, their concentrations varied depending on the cultivar/rootstock and the part of the shoot. Endogenous phenolic compounds are considered markers of a well-defined physiological state favorable to adventitious rooting (Curir et al., 1990; De Klerk et al., 1999). Furthermore, the phenolic pool can vary considerably among tissues and developmental stages, and in relation to the physiological status of plants (Pinhatti et al., 2010; Franklin and Dias, 2011).
The involvement of phenolic compounds in adventitious root formation has been well established for a long time (De Klerk et al., 1999; De Klerk et al., 2011; Denxa et al., 2012; Tahir et al., 2022). Several o-diphenols, p-diphenols, coumarins, and polyphenols are reported to inhibit auxin oxidation by scavenging the free radicals that catalyze the peroxidase reaction (Denxa et al., 2012). On the other hand, several other phenolic compounds, such as monophenols and m-diphenols, may inhibit the rooting process by stimulating IAA oxidation (Tafuri et al., 1972; Denxa et al., 2012), while others have no regulatory effect (Trobec et al., 2005). In the current study, there was an increase in accumulation of chlorogenic acid in M.9 and M.26 shoots throughout the culture period, while decreases in salicylic acid content were evident. These observations suggest that chlorogenic acid might be an essential phenolic compound which triggers the induction and proliferation of adventitious roots in apple. Similar to the current findings, Franklin and Dias (2011) showed that chlorogenic acid plays a role in root induction and root hair growth in medium supplemented with NAA. The promotive effect of chlorogenic acid on adventitious root induction has also been reported in olive stem cuttings (Denxa et al., 2012).
In this study, the HR and FJ shoots had significantly increased in salicylic acid concentrations during culture period. Accumulation of salicylic acid increases IAA decarboxylation in apple ‘Jork 9’, which in turn reduces adventitious root induction (De Klerk et al., 2011)
Furthermore, several phenolic compounds such as phloretic acid, catechol, and phloroglucinol have been reported to stimulate root formation, while rutin and tannic acid have been shown to inhibit root formation in woody species (Jones and Hatfiled, 1976; Zimmerman, 1984). The concentrations of phloretic acid, coumaric acid, catechin hydrate, hesperetin, rutin, quercetin, and biochanin were low and remained similar throughout the culture period in the different apple cultivars and rootstocks.
Conclusions
In the current study, we conducted a qualitative and quantitative analysis of phenolic acids in in vitro-cultured shoots of apple cultivars and rootstocks, namely M.9 and M.26 (easy-to-root rootstocks), and HR and FJ (difficult-to-root cultivars). Various phenolic acids, such as phloretic acid, coumaric acid, salicylic acid, chlorogenic acid, catechin hydrate, hesperetin, rutin, quercetin, and biochanin, were detected in apple shoots. The hyperaccumulation of chlorogenic acid in M.9 and M.26 and reduced concentrations in HR and FJ could explain the induction and inhibition of rooting in easy-to-root rootstocks and difficult-to-root cultivars, respectively, on a medium supplemented with IBA. Accumulation of lower levels of salicylic acid in the easy-to-root rootstocks (M.9 and M.26) and overaccumulation in difficult-to-root cultivars (HR and FJ) could also explain the inhibition and induction of rooting in these cultivars. This study sheds light on the role of endogenous phenolic compounds in the induction of adventitious roots in apple. Further studies are needed to reveal the overall mechanism by which phenolics and their levels affect oxidative decarboxylation of auxins.