Introduction
Materials and Methods
Plant Materials and Treatments
Determination of Panicle Characteristics and Flowering Dates
Determination of Fruit Characteristics
Statistical Analysis
Results and Discussion
Greenhouse Temperature Conditions
Effects of NAA on Panicle Development and Flowering Patterns
Effects of NAA on Fruit Development
Conclusion
Introduction
Mango (Mangifera indica L.) is a widely consumed tropical fruit known for its delicious taste, vibrant color, and numerous health benefits. Mango production of fruits cultivated in over 100 countries exceeded 57 million tons in 2021, making it a major contribution to the global fruit trade (FAO, 2021). Traditionally grown in tropical and subtropical regions, mango cultivation has expanded to temperate regions, including Far East Asia, owing to the increasing domestic demands and favorable climatic conditions resulting from climate change (Chung et al., 2021; Lim et al., 2021; Jung et al., 2022). However, mango trees are sensitive to cold and require greenhouse cultivation in temperate regions, which limits their cultivar selection and farm expansion.
Mangoes have excessive vegetative growth, a common characteristic of cultivars, which results in reduced fruit yield and lower market value (Yeshitela et al., 2004). Temperature is the most critical factor driving the transition from vegetative to reproductive growth (Ramírez et al., 2014). Mango trees require cool temperatures to initiate inflorescence development, which is associated with changes in plant hormones such as abscisic acid, auxin, and gibberellic acid (Luo et al., 2019). However, during greenhouse cultivation, the ambient temperature during the daytime can unexpectedly increase (Kim et al., 2021), leading to vegetative shoot stages on bearing branches instead of reproductive shoot stages. This can hinder fruit production by decreasing the number of reproductive shoots which undergo stages ranging from floral bud breakage to panicle and flower development.
Various strategies, including pruning and plant growth regulators, have been used to regulate vegetative shoot growth. Pruning involves the removal of unwanted shoots from bearing branches to direct resources toward reproductive shoots (Cheon et al., 2021). However, this strategy is labor-intensive and not cost-effective for large-scale commercial cultivation. Growth regulators have shown promising results for controlling the shoot growth of mango trees, with one example being paclobutrazol as an enzyme inhibitor (Yeshitela et al., 2004) and exogenous auxin, gibberellic acid, and brassinosteroids (Nkansah et al., 2012; Tepkaew et al., 2022). Paclobutrazol has been widely applied to fruit trees to suppress vegetative growth by regulating the biosynthesis of abscisic acid, auxin, and gibberellic acid (Opio et al., 2020). However, paclobutrazol has potential toxic effects on the environment and non-target organisms, including humans, when used in excessive amounts or when residues persist in the soil or water (Zhang et al., 2019). Many countries have imposed strict regulations on the use of paclobutrazol in crop cultivation, setting limits on the amounts that can be applied, the specific crops it can be used on, or the timing of its application. In some cases, countries have completely banned the use of paclobutrazol due to these safety concerns. Therefore, alternative growth regulators that are safe and effective for mango cultivation are required.
One such compound is 1-naphthaleneacetic acid (NAA), which is a synthetic auxin that plays multiple roles in regulating vegetative and reproductive growth. It is considered safe for plants at recommended concentrations and has been used in tissue culture and fruit thinning (Sajjad et al., 2015; Yoo et al., 2016; Tepkaew et al., 2022). As a growth regulator, NAA affects plant reproduction, including panicle development (Jain and Khurana, 2009). Although there is limited research on the use of NAA to inhibit vegetative growth in mangoes, it has been shown to be effective in controlling shoot growth in other crops (Arpaia et al., 2007). Therefore, we hypothesize that a NAA treatment will enhance panicle and fruit development in ‘Irwin’ mango trees cultivated in greenhouses under temperate conditions, thereby improving overall yield and fruit quality. In this study, we aimed to investigate the effects of different concentrations of NAA on the panicle and fruit characteristics of ‘Irwin’ mango trees grown in a greenhouse. Our findings provide valuable insights into the use of NAA as a growth regulator in mango cultivation and may help to improve the yield and quality of mangoes, especially in temperate regions.
Materials and Methods
Plant Materials and Treatments
Twelve-year-old ‘Irwin’ mango trees were selected as the plant materials for this study. The trees were cultivated in a greenhouse located in an experimental orchard of the National Institute of Horticultural and Herbal Science, Rural Development Administration, Jeju (33°28'N, 126°31'E), Republic of Korea. The mango cultivation guideline of Jeon et al. (2013) was followed to maintain optimal tree health and growth. The minimum temperature of the greenhouse was maintained at 10°C to prevent chilling injuries in the mango trees. This was done using hot air blowers during the winter season, as recommended in the guidelines (Jeon et al., 2013). Three different concentrations of NAA (0, 30, and 70 mg·L-1) were applied at the BBCH (Biologische, Bundesanstalt, Bundessortenamt, and Chemische Industrie) scale of 011 (early to mid-September 2021) to the shoot apices of all bearing branches. Treatments were arranged in a randomized complete block design with three blocks. Three trees were used for each treatment and block, with a buffer tree on either side of the record tree to minimize interference. To reduce the risk of drift problems further, two buffer rows were maintained between each block. Temperature conditions in the greenhouse were monitored daily from September of 2011 to August of 2022 using data loggers (WatchDog 2450, Spectrum Technologies, Inc., Aurora, IL, USA).
Determination of Panicle Characteristics and Flowering Dates
Panicle characteristics and flowering dates were determined at a BBCH scale of 615 (mid to late March 2022), which represents the phenological stage of full flowering, as described by Delgado et al. (2011). The panicle emergence rate was calculated as the percentage of bearing branches with panicles compared to the total number of bearing branches. The ratio of panicles to the number of bearing branches was determined by counting the number of panicles on each branch. Panicle malformation was assessed according to the criteria outlined by Hafiz et al. (2008) and was expressed as a percentage. For the index of panicle quality, we created the formula
,
where RPQ is a measure of the relative panicle quality ranging from 0 to 1, with values close to 1 indicating good quality; PER indicates the panicle emergence rate and is expressed as a percentage; PERmax is the maximum value among all samples used in this study; RPB is the ratio of panicles to bearing branches, with a value of 1 being considered best for practical mango cultivation; PM is the percentage of panicle malformations; and PMmax is the maximum PM value among all samples used in this study.
Determination of Fruit Characteristics
The fruits were harvested at a BBCH scale of 809, which corresponds to the phenological stage of fully developed fruits, as defined by Delgado et al. (2011). The fruits were characterized according to the procedure outlined by Chung et al. (2021). Peel color was evaluated using a CM-5 spectrophotometer (Konica Minolta, Tokyo, Japan) and described using the CIELAB L*, a*, and b* color space coordinates. Measurements were performed at three points on the fruit equator. Total fruit and pulp fresh weights (FW) were measured using a digital scale (Jw-1-2000, ACOM, Republic of Korea), and the fruit diameter and length were determined using a digital caliper (CD-15APX, Mitutoyo, Japan).
The fruit soluble solid content (SSC) was determined using a digital refractometer (HI96801, Hanna, Romania) and was expressed as °Brix. The titratable acidity of the pulp was determined via titration and expressed as milligrams tartaric acid (TA) equivalent (Eq.) per liter of aqueous extracts. Peel firmness was determined using a CT3 texture analyzer (AMETEK Brookfield, Middleboro, MA, USA). A puncture test was carried out on one side of the fruit using a 2.0 mm diameter cylindrical probe at three different equidistant points along the fruit equator, with a crosshead speed of 0.5 mm·s-1 and a penetration depth of 4.0 mm. The equatorial region of the mango was chosen as the point at which the textural parameters were measured, as this point provided more consistent results than other regions on the fruit surface. The maximum force required to break the peel was expressed as the peel firmness (N).
Statistical Analysis
Statistical analyses were conducted using the R 4.0.2 and RStudio 1.3 software packages. Statistically significant differences were determined by means of a one-way analysis of variance with Agricolae v1.3.3. Means were compared using Duncan’s range multiple test at p < 0.05.
Results and Discussion
Greenhouse Temperature Conditions
Greenhouse temperatures fluctuated during mango cultivation (Fig. 1 and Suppl. Table 1). The maximum temperature reached 45°C; the minimum temperature dropped to 5°C owing to severe cold conditions, although air blowers were set up to maintain a temperature of 10°C, as noted earlier. The flower buds of the mangoes initiate mainly in November and December under practical greenhouse cultivation on Jeju Island (Jeon et al., 2013). During flower bud initiation in this case, the maximum temperature exceeded 35°C, and the minimum temperature dropped to 5°C (Fig. 1 and Suppl. Table 1). Lim et al. (2016) reported that the flower bud of ‘Irwin’ mango initiated at an average temperature of 15°C in a greenhouse in Jeju Island. Similarly, Núñez-Elisea and Davenport (1995) found that the floral induction of ‘Tommy Atkins’ mango occurred at 15°C during bud dormancy, while temperatures approaching 30°C hindered the floral induction process. Australian mango cultivars showed that a minimum temperature of 18°C initiated the development of the panicle, but a high minimum temperature caused vegetative growth (Clonan et al., 2021). In this study, although the minimum temperature was the proper condition to initiate floral buds, the maximum temperature delayed and even inhibited their initiation. It was found here that the difference between the daily minimum and maximum temperatures varied from 1.3 to 23.9°C during the period of flower initiation. Differences between the daily maximum and minimum temperatures disrupts flower initiation and development (Clonan et al., 2021). This result indicates that the temperature conditions of greenhouses on Jeju Island, the main mango cultivation area in the Republic of Korea, are unfavorable for reproductive growth during winter.
Effects of NAA on Panicle Development and Flowering Patterns
NAA treatment resulted in the recovery of reproductive growth by improving panicle development in mango trees (Table 1). The emergence rate of panicles increased by 7.2 and 4.4 percentage points in mango trees treated with 30 and 70 mg·L-1 of NAA, respectively, compared to the control. The ratio of panicles to bearing branches remained consistent between the control group and the trees treated with 30 mg·L-1 of NAA. However, the ratio increased significantly to 2.0 for those treated with 70 mg·L-1 of NAA. As the NAA concentration was increased, the percentage of panicle malformation demonstrated fluctuations, with values of 33.3, 31.3, and 45.3% observed. In contrast to the observed differences, the length and diameter of the panicles did not vary after the NAA treatment. In addition to changes in the panicle characteristics, the pattern of flowering bloom in the panicles also changed over time (Fig. 2). The distribution in the control group showed a double peak in late February and mid-March. An NAA concentration of 30 mg·L-1 alleviated the distribution of flowering bloom with three peaks; even that of 70 mg·L-1 induced early full blooming relative to other treatments.
Table 1.
Panicle characteristics of ‘Irwin’ mango (Mangifera indica) treated with different concentrations of 1-naphthaleneacetic acid (NAA)
NAA (mg·L-1) |
Emergence (%) | RPB |
Malformation (%) |
Length (mm) |
Diameter (mm) | RPQ |
0 (control) | 85.4 | 1.7 | 33.3 | 320.6 ± 97.27 az | 9.8 ± 2.66 a | 0.33 |
30 | 92.6 | 1.7 | 31.3 | 328.1 ± 107.44 a | 10.0 ± 2.97 a | 0.37 |
70 | 89.8 | 2 | 45.3 | 335.5 ± 100.66 a | 10.4 ± 2.96 a | 0.24 |
Significance | - | - | - | ns | * |
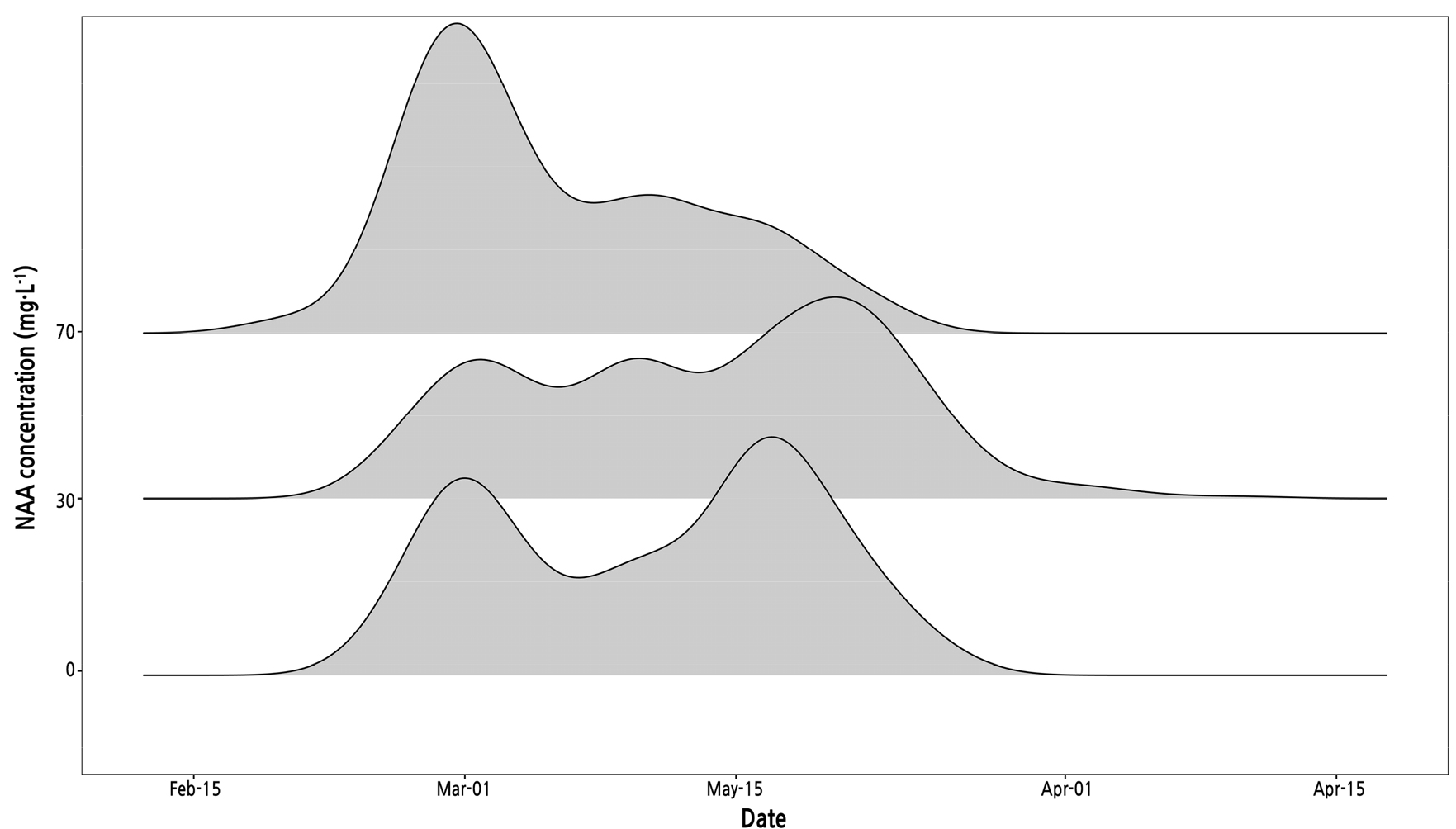
Fig. 2.
Density distribution of flowering dates for ‘Irwin’ mango (Mangifera indica) panicles treated with different concentrations of 1-naphthaleneacetic acid (NAA). The flowering dates were determined at the BBCH scale of 615, according to Delgado et al. (2011).
The effects of a NAA treatment on reproductive growth have been reported in fruit crops (Amrendra et al., 2016). In litchi, the rates of panicle emergence after a treatment with NAA concentrations of 15, 25, and 40 ppm increased compared to the control; however, the difference depended on the NAA concentration used (Braj and Satya, 2011; McArtney et al., 2013; Amrendra et al., 2016; Kaur et al., 2021). In ‘Amrapali’ mango cultivars, panicle malformation was reduced by up to 57.9% in mango trees treated with a NAA concentration of 200 ppm (Braj and Satya, 2011). Aliyu et al. (2011) reported that cashew cultivars treated with NAA bloomed earlier than a control. In addition, NAA can reduce alternate bearing (Kaur et al., 2021) and return to blooming by transiting vegetative buds to floral buds in apple cultivars (McArtney et al., 2013). Exogenous auxins, including NAA, can also substitute for endogenous auxins in the absence of appropriate activation at the tips of lateral organs (Benková et al., 2003; Jain and Khurana, 2009; Cheng et al., 2023). In chestnut, auxin-responsive genes, such as APETALA, were rapidly induced by a NAA treatment (Cheng et al., 2023). In Arabidopsis, PIN proteins are involved in the formation of various plant organs, including roots, leaves, and flowers (Benková et al., 2003). NAA has been shown to restore PIN expressions in pin mutants (Benková et al., 2003). However, due to the gradient module of plant organ formation (Benková et al., 2003), using the correct concentration is important for the application of NAA on farms. In this study, RPQ, the index of overall panicle quality, indicated that an NAA concentration of 30 mg·L-1 positively affected panicle development in mango cultivation.
Effects of NAA on Fruit Development
NAA treatments affected fruit development, as evidenced by changes in the morphological and physicochemical characteristics (Tables 2, 3, and 4). The fruit lengths of mango trees treated with NAA did not differ from that of the control. However, the diameters increased from 80.4 mm to 85.8 mm regardless of the NAA concentration (Table 2); the total weight of the fruit increased correspondingly by 116%. The peel characteristics of the fruit, specifically the chromaticity and thickness, were similar between the control and mango trees treated with NAA (Table 3). However, there was a noticeable difference in the peel thickness between the mango trees treated with the two different concentrations of NAA (30 and 70 mg·L-1). The fresh weights of the pulp increased in mango trees treated with NAA concentrations of 30 and 70 mg·L-1 with values of 325 and 339 g FW, respectively, compared with that of the control (279 g FW). Pulp firmness decreased in mango trees treated with NAA regardless of the concentration. SSC and TA were not different among all the mango trees, with values of 13.5°Brix and 4.6 mg TA Eq·L-1, respectively.
Table 2.
Length, diameter, and fresh weight of ‘Irwin’ mango (Mangifera indica) fruit treated with different concentrations of 1-naphthaleneacetic acid (NAA)
NAA (mg·L-1) |
Length (mm) |
Diameter (mm) |
Total fruit weight (g FW) |
0 (control) | 103.6 ± 4.74 az | 80.4 ± 5.41 b | 366.0 ± 54.65 b |
30 | 108.4 ± 8.83 a | 84.9 ± 4.89 a | 421.9 ± 68.46 a |
70 | 105.7 ± 6.04 a | 85.8 ± 4.19 a | 424.7 ± 50.29 a |
Significance | ns | * | * |
Table 3.
Peel characteristics of ‘Irwin’ mango (Mangifera indica) treated with different concentrations of 1-naphthaleneacetic acid (NAA)
NAA (mg/L) | Chromaticity |
Thickness (mm) | ||
L* | a* | b* | ||
0 (control) | 43.2 ± 7.16 az | 21.7 ± 7.97 a | 25.9 ± 11.02 a | 6.6 ± 1.05 ab |
30 | 39.3 ± 8.04 a | 21.5 ± 5.32 a | 23.0 ± 10.91 a | 6.1 ± 1.25 b |
70 | 39.3 ± 7.13 a | 22.3 ± 3.49 a | 19.7 ± 10.88 a | 7.5 ± 1.62 a |
Significance | ns | ns | ns | * |
Table 4.
Pulp characteristics of ‘Irwin’ mango (Mangifera indica) treated with different concentrations of 1-naphthaleneacetic acid (NAA)
NAA (mg/L) |
Pulp weight (g FW) |
Pulp firmness (N) |
Soluble solid content (°Brix) |
Titratable acidity (mg TA Eq.·L-1z) |
0 (control) | 279.4 ± 49.84 by | 9.2 ± 1.04 a | 13.2 ± 2.10 a | 4.5 ± 1.37 a |
30 | 325.5 ± 55.68 a | 6.9 ± 2.12 b | 13.5 ± 1.31 a | 4.6 ± 1.33 a |
70 | 339.2 ± 44.83 a | 6.3 ± 1.98 b | 13.7 ± 0.99 a | 4.8 ± 0.83 a |
Significance | * | ** | ns | ns |
Exogenous plant hormones can affect fruit characteristics when applied at flower bud initiation in mango (Singh and Dhillon, 1986; Singh and Dhillon, 1990; Nkansah et al., 2012). Ethylene treatments in ‘Dashihari’ mango have been reported to reduce fruit weights and yields (Singh and Dhillon, 1990). Exogenous auxins, including NAA and IBA, increase fruit yields in ‘Dashihari’ mango, with varying responses depending on the concentration (Singh and Dhillon, 1986; Nkansah et al., 2012). In the present study, the NAA treatment affected fruit size, weight, and firmness (Tables 2 and 4), which could be explained in the context of cell development given that auxin plays a role in promoting cell division and expansion (Pattison et al., 2014; Majda and Robert, 2018). Auxin, in particular, regulates cell wall properties by initiating wall loosening (Majda and Robert, 2018). Auxin decreases the stiffness of the cell wall through the demethylesterification of pectins, resulting in organ outgrowth (Majda and Robert, 2018). With the NAA treatment, accelerated cell expansion increases the fruit size and the capacity to store water and metabolites (Majda and Robert, 2018). However, firmness may be reduced due to changes in cell turgor pressures (Thomas et al., 2008). Further analyses are needed to clarify the effects of exogenous auxins on cell structures.
Conclusion
Temperature fluctuations of greenhouses during mango cultivation on Jeju Island were not ideal for reproductive growth, although the controlled environment ensures that there is little variation in environmental conditions from year to year, minimizing the impact on the variance of reproductive growth. However, a NAA treatment showed promise in improving the development of panicles, as indicated by increased emergence rates, changes in flowering patterns, and fewer panicle malformations. Moreover, the NAA treatment affected fruit development, particularly the size and weight, which could be attributed to its ability to promote cell expansion. Our findings provide valuable insight into the use of NAA as a growth regulator in mango cultivation, particularly in regions with challenging environmental conditions. These results have practical implications for enhancing the yield and quality of mango, which can benefit both growers and consumers. However, further research is needed to gain a full understanding of the effects of exogenous auxins on cell structures and to optimize the NAA treatment for different cultivars and cultivation conditions. Further studies should also explore the potential long-term impacts of NAA on mango tree health and productivity, as well as the environmental consequences of its use.
Supplementary Material
Supplementary materials are available at Horticultural Science and Technology website (https://www.hst-j.org).
- HORT_20230033_Table_1s.xlsx
Daily temperatures and the difference of maximum and minimum temperatures at the greenhouse of the experimental site (33° 28ʹ N, 126° 31ʹ E) from the 1st September 2021 to the 31th August 2022.