서 언
재료 및 방법
식물 재료 및 온도 처리
RNA 분리 및 real-time PCR 분석
전사체 분석 및 기능 예측
차등발현 유전자 분석
결과 및 고찰
온도 처리에 따른 ‘후지’ 사과나무 잎의 전사체 분석
특이발현 유전자의 GO 기능분석
고온에서 특이적으로 발현되는 사과나무 잎의 유전자 선발
고온 처리에 따른 주요 유전자의 발현 분석
서 언
사과는 전세계적으로 생산되는 주요 온대 과수이며 국내에서 가장 많이 생산되는 과종으로 2021년도에 34.4천ha의 면적에서, 516천톤의 과실이 생산되어 생산액은 1조 3천억에 이른다(KREI, 2023). 농촌진흥청은 기후변화 시나리오를 기반으로 주요 6대 과수의 총 재배 가능지를 2090년까지 10년 단위로 전망한 예측지도에서 사과는 재배 적지와 재배 가능지가 지속적으로 급격히 줄어들고, 2070년대에는 강원지역의 일부에서만 재배가 가능할 것이라고 예측하고 있다(Yun et al., 2020). 따라서, 국내에서 사과를 생산하기 위해서는 지구온난화에 따라 발생할 수 있는 문제점을 해결하기 위한 대응책의 제시가 필요한 실정이다.
지구의 온도 상승은 해수면 상승, 강수량과 강수유형의 변화, 기상 이변의 빈도 및 강도 증가 등의 변화를 야기하며, 영년생 작물인 과수에서는 수체 생육 불량 등으로 인한 생산량과 품질을 저하시키는 요인이 될 수 있다(Lee et al., 2008; Florides and Christodoulides, 2009; Sun et al., 2012; Son et al., 2014). 대부분의 식물에서는 잎의 온도가 38°C를 초과하면 다른 스트레스 증상이 나타나지 않더라도 광합성 기구는 방해를 받게 되며(Edwards and Walker, 1983; Haldimann and Feller, 2004), 생육 기간 중의 광합성 감소로 인해 수체 생육이 억제되고 과실의 품질이 저하된다(Higuchi et al., 1998). 야간의 고온은 원예작물에서 결실 저해를 가져오고, 호흡률의 증가로 인한 양분 소모 등으로 생육을 불량하게 하여 결국 수체 충실도가 저하된다고 보고되어 있다(Acock et al., 1990; Lohar and Peat, 1998; Calderón-Zavala et al., 2004; Son et al., 2014; Ryu et al., 2015). 사과 과실의 발육에 있어서도 온도 상승은 과실의 생장과 성숙 지연, 당함량 저하, 과피의 착색 불량, 불량과 발생, 과실의 저장수명 단축 등을 유발하여 경제적인 손실을 초래한다(Hulme et al., 1994).
일본의 농림수산성에서는 지구온난화와 기후변화에 따른 대책을 품목별로 수립하여 실제 활용이 가능한 기술을 개발하는 연구를 수행하고 있다(MAFF, 2021a, 2021b). 국내에서도 기후 변화와 지구온난화에 의한 지속적인 고온은 과실 생장에 부정적인 영향을 미치고, 생리장해의 한 종류인 밀증상도 생육기의 저온보다는 고온에서 발생률 및 발생 정도가 심한 것으로 보고되어 있다(Park et al., 2009). 우리나라에서도 사과를 대상으로 고온에 의한 과수 생육과 과실의 특성변화를 구명하고자 관련 유전자의 발현을 분석하여 보고하고 있으나(Kim et al., 2015a, 2015b, Kim et al., 2016), 사과나무의 생육과정에서 온도상승에 따른 수체의 생리· 생태적 변화를 구명하는 분자생물학적 수준의 연구는 전무한 실정이다. 따라서, 본연구에서는 생육기에 고온에 노출된 사과나무 잎의 전사체를 분석하여 고온에 대응하여 특이하게 발현이 조절되는 유전자군을 대량으로 선발하고, 선발된 유전자군의 온도 변화에 대응하여 발현되는 양상을 구명하여 향후 기후변화 대응 연구에 요구되는 정보를 획득하고자 하였다.
재료 및 방법
식물 재료 및 온도 처리
농촌진흥청 국립특작원예과학원의 온난화대응농업연구소에서 화분에 재식한 5년생 ‘후지’/M9 사과나무를 대상으로 RCP 시나리오에 따라 구현된 온도 조건을 48시간 수체에 처리하였다. 사과나무 화분은 생육상(온도 조건; 25 ± 1°C, 30 ± 1°C, 35 ± 1°C, 상대습도; 60 ± 10%, 광조건; 16시간/8시간(광/암))에서 광환경은 800μmol·m-2s-1, 이산화탄소 농도는 450μmol·mol-1의 조건으로 고정하여 단기간 처리한 후 RNA-seq 분석을 진행하였다. 온도구배하우스에서는 각각 3구역으로 구획하여 사과나무 화분을 둔 후 각각 고온(오후 2시) 시간대의 온도 조건(저온, 29.5°C; 중간, 33.3°C; 고온, 35.5°C)과 저온(오후 7시) 시간대의 온도 조건(저온, 22°C; 중간, 25°C; 고온, 27°C)에 잎을 채취하여 초저온 냉동고에 보관하고, 유전자 발현을 분석하기 위한 RNA 추출에 사용하였으며, 각 처리는 3반복으로 실시하였다.
RNA 분리 및 real-time PCR 분석
각각 처리된 온도별로 채취한 사과나무 잎은 막자 사발에서 액체질소를 충분히 유지하면서 완전히 마쇄하였다. RNA 분리는 Chang et al. (1993)의 방법을 수정하여 1, 2차 추출 단계의 원심분리 조건을 3,200rpm, 30분으로 수정하여 사용하였다. 분리한 RNA 품질은 1% 아가로스젤에서 전기영동으로 확인하였고, RNA의 농도 및 순도는 Nano Drop spectrophotometer (ND-1000, NanoDrop Technologies Inc., Wilmington, USA)를 이용하여 측정하였고, RNA 시료의 순도는 Agilent 2100 Bioanalyzer를 사용하였다.
분리한 RNA(500ng)는 역전사효소(GoScriptTM, Promega, USA)를 이용하여 cDNA를 합성하고 PCR 반응을 위한 주형으로 사용하였다. Real-time PCR 조건은 SYBR Premix Ex TaqTM(TaKaRa Bio Inc., Japan) 제조사의 방법을 이용하여 20μL 반응액을 조제하고, C1000TM Thermal Cycler(CFX96TM Real-Time System, BioRad, USA)를 이용하여 분석하였다. 반응 조건은 95°C에서 30초 후, 95°C에서 5초, 60°C에서 30초 반응으로 40회 반복하였다. 발현 분석은 증폭량에 따른 형광 강도를 각 cycle 마다 측정하였고, 각각의 복제유도절편에 의한 발현값은 사과 actin 유전자의 발현값에 비례하여 상대적으로 계산하였다.
전사체 분석 및 기능 예측
전사체 분석은 Ahn et al. (2019)의 방법으로 분석하였다. 표준유전자 정보는 Phytozome(version 10.2) (Goodstein et al., 2012)에서 수집한 사과 표준유전자 서열을 이용하여 전사체 염기서열 데이터의 유전자 발현량 분석을 수행하였다. 각각의 처리구는 3반복으로 잎을 채취하였고, Illumina Hiseq으로 염기서열을 분석하였다.
모든 전사체들은 포도나무/애기장대 데이터베이스에 BLASTN 및 BLASTX을 이용하여 예측을 수행하였다. 각 전사체의 예측 정보는 유전자 온톨로지(GO)와 KEGG(Kyoto Encyclopedia of Genes and Genomes)의 기능 및 대사경로 분석에 사용하였다. RNA-seq데이터에서 서열 품질의 확인과 부정확한 서열의 정제는 SolexaQA 패키지(Cox et al., 2010)의 DynamicTrim과 LengthSort를 이용하여 수행하였다.
차등발현 유전자 분석
전사체 분석의 유전자 발현을 기반으로 하여 25°C와 35°C의 온도 처리구에 의해 차등적으로 발현되는 유전자 확인은 발현차이 값이 1.5배와 2배 이상 차이가 나는 것과 p-value ≤ 0.01을 기준으로 선발하였다. 사과 표준 유전자에 예측 된 애기장대의 GO 정보를 이용하여 functional category 분석을 수행하였다. 분석기준은 기능별 유전자의 총 수가 개 ≥ 5인 것을 사용하였고, GO 심도는 3으로 지정하여 3가지 기능 영역인 분자기능(BP), 세포내외 위치(CC), 분자기능(MF)으로 분류하여 분석을 수행하였다.
결과 및 고찰
온도 처리에 따른 ‘후지’ 사과나무 잎의 전사체 분석
‘후지’ 사과나무 잎의 전사체 분석을 수행하여 25°C와 35°C 처리구에서 각각 총 37,115,590와 44,989,062의 paired-end reads(101bp)를 얻었으며, 품질이 낮은 염기서열 분석 결과를 제거한 후 각각 총 34,158,132와 41,475,948의 전사체를 확보하였다. 전체 길이는 6,812Mb이고, 평균 길이는 25°C와 35°C 처리구에서 각각 90.0bp와 90.2bp이며, 평균 82.9%의 결과 자료가 분석에 활용되었다(Table 1).
Table 1.
Statistics of the sequenced transcripts from ‘Fuji’ apple tree leaves exposed to different temperatures
전사체 분석을 통해 고온에 노출된 사과나무 잎에서 발현 차이가 나타나는 유전자군을 선발하였다. 사과나무 잎에서 25°C 처리구에 비해 35°C 처리구에서 2배 이상의 발현 차이를 보이는 41개의 유전자가 검출되었으며, 발현이 유도된 유전자는 21개, 발현이 억제된 유전자는 20개였고 모두 표준유전체에 예측되었다. 발현차이 값이 1.5이상의 발현 차이를 나타내는 유전자를 대상으로 하였을 경우에는 213개의 유전자가 발현이 유도되었고, 그 중에서 202개의 유전자가 예측동정되었으며, 231개의 유전자는 발현이 억제되었고 225개의 유전자가 예측동정되었다(Table 2).
Table 2.
Differentially expressed genes in ‘Fuji’ apple tree leaves exposed to 35°C and 25°C
Sample (Control vs treatment) |
Regulation pattern | 2-Fold change | 1.5-Fold change | |||
No. of DEGs | No. of DEGs | No. of DEGs | No. of annotated DEGs | |||
25°C vs 35°C | Up | 21 | 21 | 213 | 21 | |
Down | 20 | 20 | 231 | 20 |
특이발현 유전자의 GO 기능분석
전사체 분석을 통해 얻어진 DEG를 대상으로 GO분석을 실시한 결과, DEG는 3개의 주요 영역으로 구분되었다. 25°C 처리구에 비해 35°C 처리구에서 발현이 유도된 DEGs를 영역별로 보면, 세포기작 범주에서는 생합성 관련 기작(19), 질소화합물 대사(17), 화합물 반응 기작(11)이 주요 하위 범주였다(Fig. 1). 세포내외위치 범주에서는 intracellular part(13)와 ribonucleoprotein complex(12)가 주요 하위 범주였으며, 분자기능 범주에서는 organic cyclic compound binding(15)과 hydrolase activity(12)가 주요 하위 범주로 분류되었다. 25°C 처리구에 비해 35°C 처리구에서 발현이 억제된 DEGs는 , 세포기작 범주에서는 비생물적 자극에 대한 반응(33), 질소화합물대사(21), single-organism cellular process(20)가 주로 분류되었고, 세포내외위치 범주에서는 cell periphery(19)와 intracellular part(14)에 주로 분류되었으며, 분자기능 범주에서는 가수분해활성(23), organic cyclic compound binding(18), 이온결합(16)에 주로 분류되었다.
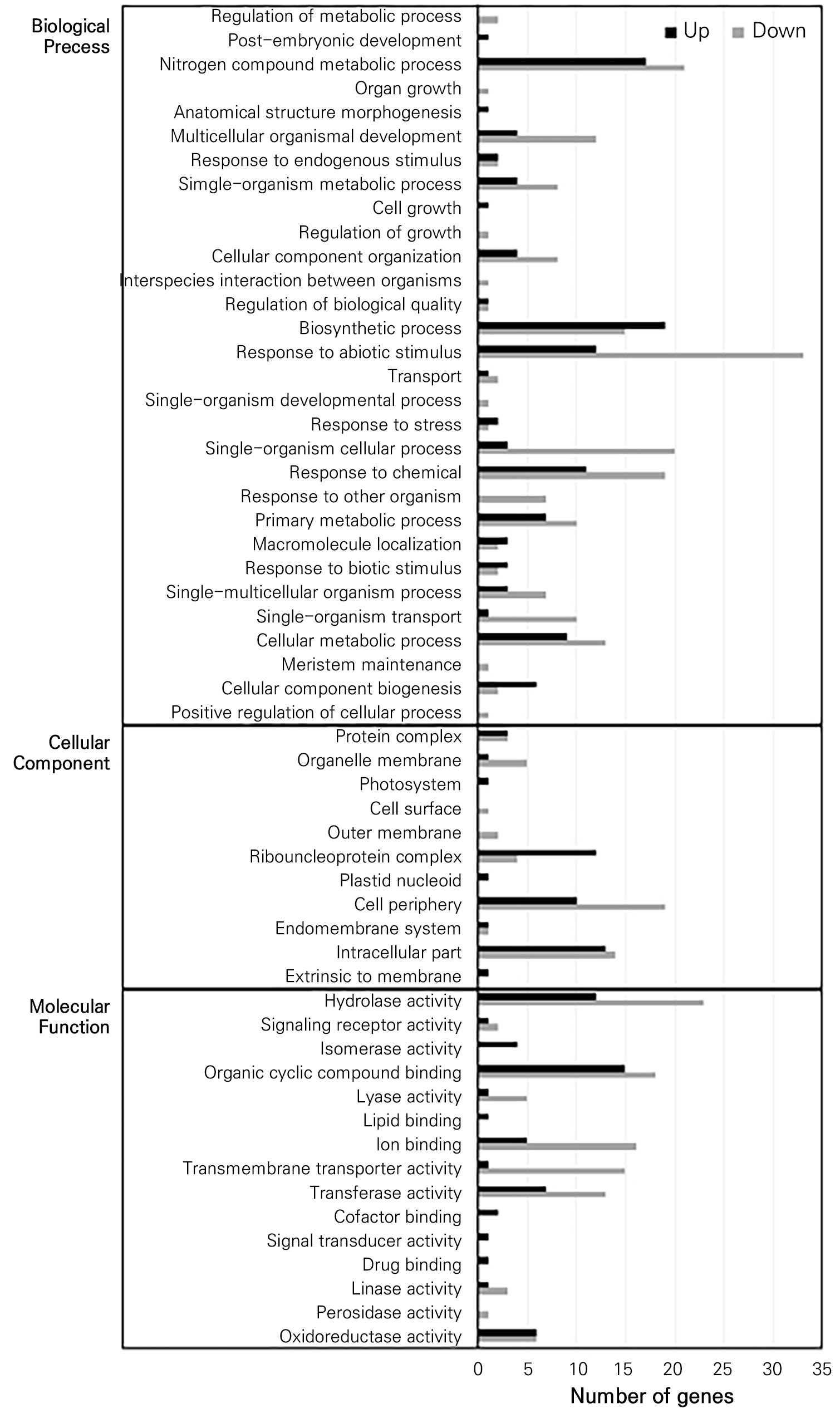
Fig. 1.
GO classification of up- and down-regulated DEGs, and GO classification of up- and down-regulated DEGs in ‘Fuji’ apple tree leaves exposed to 35°C vs 25°C. The DEGs are summarized in three main categories: biological processes, cellular components and molecular functions. The X-axis indicates the number of genes and the Y-axis indicates the GO terms.
고온에서 특이적으로 발현되는 사과나무 잎의 유전자 선발
사과나무 잎에서 고온 처리에 의해 특이적으로 발현이 변화되는 유전자군을 선발하였다. 발현이 유도되는 상위 10개의 유전자와 발현이 감소하는 하위 10개의 유전자를 선발하였으며, real-time PCR을 통해 선발된 유전자의 발현 양상을 분석하였다(Table 3 and Fig. 2). 고온에 의해 사과나무 잎에서 발현이 유도되는 유전자는 GDSL-like Lipase/Acylhydrolase superfamily protein, cell wall protein precursor, MLP-like protein 423, heat shock protein 18.2, HSP20-like chaperones superfamily protein, phytosulfokin receptor(PSKR) 1, protein phosphatase 2C family protein, microsomal glutathione s-transferase 등이었으며, 3-ketoacyl-CoA synthase(KCS) 19, cysteine proteinases superfamily protein, plant invertase/pectin methylesterase(INVs/PMEs) inhibitor superfamily, heat shock protein 70, ferretin 1, nitrate transporter 1.7, heat shock protein 81-2 등은 고온에 의해 발현이 감소하였다(Table 3 and Fig. 2). Real-time PCR을 통해 이들 유전자의 발현양상을 분석한 결과, 선발한 모든 유전자가 전사체 분석 결과와 일치하는 양상으로 사과나무 잎에서 크게 발현이 유도되거나 억제되었으며, 온도가 높을수록 유전자의 발현양상은 전사체 분석의 결과와 더욱 유사하게 나타났다.
Table 3.
Top 10 genes highly up-regulated and down-regulated in ‘Fuji’ apple tree leaves exposed to 35°C vs 25°C and their primer sequences used for the real-time PCR analysis
식물은 고온스트레스에 반응하는 동안 단백질의 집합을 방지하고 열-손상 단백질의 적절한 재접힘을 도와주는 molecular chaperones으로서의 기능을 하는 열쇼크단백질을 축적한다(Parsell and Lindquist, 1993). HSP70은 chaperone molecules의 주요한 단백질군의 하나이며 특히 고온 및 건조 스트레스 등에 의해 빠르게 유도된다(Rizhsky et al., 2002, Rizhsky et al., 2004; Cho and Choi, 2009). sHSP(small heat shock protein)들은 스트레스에 반응하여 합성되며, HSP70과 함께 세포 단백질의 집합을 방지하고 부분적인 내열성과 스트레스내성 획득에 중요한 요인으로 작용한다(Vierling, 1991; Wang et al., 2004; Grigorova et al., 2011). 고온에 노출된 포도나무 잎에서도 다양한 열쇼크단백질 유전자들이 발현되었다(Liu et al., 2012). 본 연구에서는 사과나무에서 HSP18.2과 HSP20-like chaperones superfamily protein은 고온에서 발현이 유도되었고, HSP70, HSP81-2, HSP81-4 등의 유전자는 고온에서 발현이 감소하였다.
고온에 노출된 애기장대 잎에서 GDSL-like Lipase/Acylhydrolase superfamily protein은 열 스트레스에 의해 유도되며 회복되는 동안에는 정상의 발현으로 돌아가는 경향을 가진 그룹으로 분류되었다(Higashi et al., 2018). 본 연구에서도 사과나무 잎에서 GDSL-like Lipase/Acylhydrolase superfamily protein 은 고온에서 유도되는 경향이었다.
Major latex proteins(MLPs)은 식물체에서 방어와 스트레스 반응에 관여하는 주요 유전자로 알려져 있고 사과에서는 유전체 분석을 통해 36개의 유전자가 보고되어 있지만 스트레스 저항성 반응에 대한 보고는 없으며(Yuan et al., 2020), He et al. (2020)은 MdMLP423이 스트레스 관련 방어유전자와 전사인자의 발현을 억제하여 병원균 감염에 대한 사과의 저항성을 억제한다고 보고하였다. 그러나, 본 연구에서는 이와는 무관하게 MLP-like protein 423은 고온에 의해 발현이 유도되는 경향이어서 향후 연구가 필요한 것으로 여겨진다.
PSKR은 작은 신호전달 펩타이드 PSK에 대한 결합 부위를 갖는 막관통 LRR-RLK family 단백질이다(Nagar et al., 2020). 벼의 PSKR gene family member에 대한 유전체기반 분석에서는 OsPSKR 유전자군이 고온, 가뭄 등의 외부 스트레스에 의해 차등적 발현하는 양상을 확인하였고, 특히 OsPSKR10은 고온과 염분 처리에 의해 발현이 증가하였다고 보고하였는데(Nagar et al., 2020), 본 연구에서도 비슷한 경향을 나타내었으며 PSKR유전자는 사과나무 잎에서도 고온에 반응하여 발현하는 유전자로 확인하였다.
KCS는 매우긴사슬지방산(VLCFA)의 합성 과정에서 작용하는 핵심 효소로 긴사슬지방산의 탄소 사슬 길이를 결정하며, 다양한 종류의 스트레스에 반응하여 유도된다고 알려져 있다(Fan et al., 2018; Yang et al., 2023). 열스트레스에 대한 전사체 분석을 통해 미세조류에서 열스트레스에 의해 지방산 신장과 불포화지방산의 생합성과 관련된 KCS등과 같은 유전자의 발현이 억제된다고 보고되었는데(Liang et al., 2019), 이와 유사하게 본 연구에서 고온에 노출된 사과나무 잎에서도 감소하는 경향이었다.고온에 노출된 포도나무 잎의 전사체 분석 연구에서 cysteine proteinases superfamily protein은 유도되는 경향이었으나(Kim et al., 2018a), 본 연구에서는 감소하는 경향을 나타내었는데, 이는 cysteine proteinases의 구조가 매우 다양한고 그에 따라 식물체내에서의 외부스트레스에 대한 반응도 다양하게 나타나기 때문으로 사료되며 구체적인 유전자의 구조분석과 발현에 대한 연구가 수행되어야 할 것으로 사료된다(Grudkowska and Zagdańska, 2004).
INVs/PMEs 는 탄수화물 대사, 스트레스 반응, 당 신호전달을 조정하는 필수적인 효소들이며, 고온내성과 PME34의 관계 구명 연구에서 AtPMEI-PME34는 열 반응 동안에 증산율을 조절할 수 있는데, 세포벽의 유동성과 고온내성을 조절하는데 기여하는 공변세포에서 높게 발현된다고 알려져 있다(Huang et al., 2017; Wu et al., 2017). 그러나 INV와 PME는 식물의 기관발달, 탄수화물대사, 외부스트레스에 대한 방어반응, 화분관 신장 및 기관 발달 등의 다양한 반응에 관여하므로(Coculo and Lionetti, 2022; Lee et al., 2023) 구체적인 향후 연구가 필요할 것으로 여겨진다.
전사체 분석 연구를 통해 밀에서는 산화 스트레스 반응과 관련된 ferritin 등의 유전자는 열 스트레스에 의해서 영향을 많이 받으며, 장기간보다는 단기간의 열쇼크유지 기간 동안 높은 발현량을 보이는 것으로 보고되었다(Qin et al., 2008). 포도의 스트레스 저항성 강화와 관련된 연구에서도 ferritin 2가 스트레스 반응에 있어서 매우 중요한 요인이라고 보고하였으며(Kós et al., 2008), 본 연구에서 ferritin 1 유전자는 고온에 의해 감소하는 경향인데 이는 ferritin 유전자가 여러형태로 존재하여 식물체내에서 고온, 저온, 염류, 병원균 감염 등의 외부 스트레스에 다양하게 반응하기 때문으로 여겨지며(Qin et al., 2008; Briat et al., 2010) 유전자를 대상으로 한 연구가 필요하다. 이와 같이 본 연구에서 고온에 노출된 사과나무 잎의 전사체 분석을 통해 확인된 특이적으로 발현되는 유전자는 외부스트레스에 대해 매우 다양한 기작의 경로에서 반응하는 것으로 여겨지며 방어반응에서의 구체적인 역할을 밝히기 위해서는 각각의 유전자를 대상으로 발현 조절에 대한 추가적인 연구가 필요하다.
고온 처리에 따른 주요 유전자의 발현 분석
온도 처리에 따른 사과나무 잎의 유전자 발현 분석을 위하여 온도구배하우스에서 각각 고온(오후 2시)과 저온(오후 7시) 시간대에 사과나무 잎을 채취하여 quantitative real-time PCR을 이용하여 phenylalanine ammonia-lyase(PAL), chalcone synthase(CHS), glyceraldehyde-3-phosphate dehydrogenase(GAPDH), ribulose-1,5-bisphosphate carboxylase/oxygenase (Rubisco) 유전자의 발현 양상을 분석하였다(Table 4 and Fig. 3). 온도구배하우스에서 고온 시간대의 온도 조건(저온구 29.5°C; 중간온도구 33.3°C; 고온구 35.5°C)에서 사과나무 잎을 채취하여 유전자의 발현을 분석한 경우, 플라본화합물의 생성에 관여하는 PAL과 CHS 유전자는 발현이 감소하는 경향이었다(Fig. 3). PAL 유전자는 저온구와 중간 온도구에서는 발현량의 감소가 많았으나 고온구에서는 발현량의 약간 유도되었다. CHS 유전자의 발현량은 저온구에서 가장 크게 감소하는 경향이었고, 중간온도구와 고온구에서는 약간 감소하는 경향이었다. 광합성에 관여하는 것으로 알려진 GAPDH와 Rubisco 유전자는 모든 온도 조건에서 발현이 유도되는 양상을 나타내었다. GAPDH 유전자는 저온구나 중간온도구에서는 발현이 적었으나 고온구에서는 크게 유도되었다. Rubisco 유전자는 발현이 현저하게 유도되는 경향으로 저온구에서 발현량이 가장 많았으며 고온구와 중간 온도구에서 발현이 적게 유도되었다.
Table 4.
NCBI gene accession numbers and sequences of primers gene used for a quantitative real-time PCR analysis of apple trees kept in a temperature-gradient greenhouse
저온 시간대인 오전 7시의 온도 조건(저온구, 22°C; 중간온도구, 25°C; 고온구, 27°C)에서 채취한 사과나무 잎의 유전자 발현의 분석 결과, PAL, CHS, GAPDH 유전자는 모든 온도 조건에서 발현이 감소하는 경향을 나타내었으며, 각 유전자에서는 온도 조건별로 발현량에서 큰 차이는 없었다(Fig. 3). Rubisco 유전자는 발현이 많이 유도되는 양상을 나타내었는데, 저온구에서 고온구로 갈수록 유전자의 발현량은 감소하는 경향이었다.
고온 시간대와 저온 시간대 모두에서 PAL과 CHS 유전자는 발현이 감소하는 경향을 나타내었다. GAPDH 유전자는 고온 시간대에는 발현이 유도되는 경향을 보였으나 저온 시간대에는 유전자의 발현이 감소하는 경향을 보였으며, Rubisco 유전자는 고온과 저온 시간대 모두에서 발현량이 현저하게 유도되는 경향이었다.
온도가 안토시아닌 생합성에 미치는 영향에 관한 연구는 사과(Lin-Wang et al., 2011; Kim et al., 2015a; Bu et al., 2022), 포도(Dela et al., 2003; Azuma et al., 2012; Kim et al., 2015b; Kim et al., 2018b), red orange(Lo-Piero et al., 2005), 장미(Mori et al., 2007) 등 여러 작물에서 보고되어 있다. 애기장대 잎에서 저온에 의해 안토시아닌 생성과 관련된 CHS와 PAL 유전자가 유도되며 고온에 의해서는 감소한다고 하였으며, PAP1(production of anthocyanin pigment 1) 유전자를 과발현한 연구에서 가역적인 적색의 감소현상을 보고하였다(Leyva et al., 1995; Rowan et al., 2009). 국내에서는 사과(‘후지’와 ‘홍로’) 과실에 관한 연구에서 25°C나 30°C에 비해 35°C의 고온에서 PAL 유전자의 발현이 감소하는 경향을 나타내었다(Kim et al., 2015a, Kim et al., 2016).
꽃사과나무 잎에서는 저온 처리에 의해 안토시아닌 함량은 증가하고 고온에서는 감소하였으며, 유전자 발현을 조사한 결과에서도 안토시아닌 생합성의 중요 유전자인 CHS, F3H, DFR 등의 유전자 발현이 저온에서는 매우 높고 고온에서는 낮은 경향을 보인다고 보고하였는데(Tian et al., 2015), 본 연구에서도 이러한 보고와 유사한 결과를 나타내었다.
GAPDH와 Rubisco는 식물체의 광합성과 관련된 중요한 유전자로 알려져 있다. GAPDH는 일반적으로 열감수성 품종에서는 감소하며 열내성 식물에서는 고온 조건에서 증가한다고 보고되어 있다(Wang et al., 2017). 그러나, 이러한 연구 결과와는 다르게 벼의 단백질체 분석 연구에서는 고온에 의한 벼의 잎과 유묘에서 GAPDH 효소가 고온에서 증가하였다고 보고하였다(Lee et al., 2007; Han et al., 2009). 인삼의 단백질체 분석 연구에서 인삼은 고온에 민감한 식물인데 GAPDH가 증가하였다고 보고되었는데(Kim et al., 2019), 이것은 식물이 외부 온도 상승에 대해 최소한 부분적으로 대처하려고 반응하는 것이라고 주장하였으며, 본 연구에서도 고온 시간대에서 GAPDH 유전자 발현이 소폭으로 유도되는 경향이었다.
Rubisco 활성은 RuBisCO activase(RCA)에 의해 조절되고 고온에 대한 RCA의 안정성은 RCA의 활성화 유지에 필수적이며(Salvucci and Crafts-Brandner, 2004; Li et al., 2013), RCA의 특정 동형단백질은 벼, 밀, C. spinarum 식물에서 온도에 의해 증가한다고 한다(Han et al., 2009; Zhang et al., 2010; Majoul-Haddad et al., 2013; Wang et al., 2015). 대부분의 식물은 2가지 형태의 RCA 동형단백질을 가지며 Rubisco의 활성화를 촉진할 수 있으나 효소활성과 열변성에 대한 민감성은 확연하게 다르다고 보고되었다(Majoul-Haddad et al., 2013; Wang et al., 2015). 벼 잎에서 2개의 RCA 동형단백질이 광합성 열순응에서 다른 역할을 하는 것이 보고되었는데, heat stress에 의해 RCAL(RCA large subunit)의 발현이 mRNA와 단백질 수준에서 강하게 유도되었다(Wang et al., 2017). 일반적으로 고온에 의해 rubisco 활성은 저해되지만 본 연구에서 rubisco 유전자 발현이 유도된 것은 벼의 사례와 같이 RCA 동형단백질의 형태에 따른 고온에 대한 반응이 다르게 나타난 가능성에 대해 향후 연구가 필요할 것으로 생각된다.
본연구에서는 고온에 노출된 사과나무 잎의 반응을 전사체 수준에서 분석하였으며, 주로 고온 스트레스에 반응하는 유전자군이 특이적으로 발현되는 것을 확인하였으며, 스트레스에 대한 유전자의 반응이 기존의 보고와 일치하지 않는 것은 체내에 다양한 상동유전자가 존재하여 각기 다르게 조절되는 영향으로 여겨진다. 또한, 사과나무의 잎에서도 색소 축적과 관련한 다양한 유전자의 발현이 특이적으로 조절되는 것을 확인하였다. 사과나무 잎은 물론 사과 과실의 착색과 관련한 품질도 수체의 생장기간 동안의 광합성 효율에 의해서 결정되며 온도와 같은 조건에 의해서도 영향을 받는다고 보고되어 있다(Tomana and Yamada, 1988; Saure, 1990; Song et al., 2003; Massonnet et al., 2007).
최근 기상이변으로 인해 사과를 비롯한 과수에서 겨울철 동해, 이른 봄의 냉해, 여름철 고온에 의한 과실의 성숙 및 착색 불량 등의 장해가 발생하고 있다. 이를 극복하기 위하여 생육에 불리한 기상 조건에서도 적응할 수 있는 과수 품종을 육성하거나 정상적으로 생육이 진행될 수 있는 생리적 반응을 유도하는 전략을 제시할 수 있다. 본 연구에서는 고온에서 특이적으로 발현되는 유전자군을 선발하고 온도 변화에 따라 발현이 조절되는 것을 확인하였다. 선발된 유전자는 향후 기후변화에도 정상적으로 생육할 수 있는 신품종의 육성에 중요한 육종 소재로 활용될 수 있으며, 선발 유전자의 특이적인 발현 양상 분석 결과는 고온에서도 과수의 생육과 과실의 성숙을 유도할 수 있는 방안을 모색하는 데에 유용한 정보로 활용될 수 있을 것이다.