Introduction
Materials and Methods
Plant Materials and Culture Conditions
Determination of Cd and Pb Content
Determination of Nonprotein Thiols (NPTs) and H2O2 Content
Statistical Analysis
Results
Effects of Cd and Pb on Germination and Seedling Growth
Effects of Cd and Pb Accumulation on Cd and Pb Tolerance
Effects of Nonprotein Thiols on Cd and Pb Tolerance
Effects of Cd and Pb on H2O2 Generation
Discussion
Introduction
The development of industrialization and modern agricultural practices worldwide has led to the contamination of air, water, and soil with the heavy metals released from anthropogenic activities, resulting in emissions from motor vehicles and industrial factories, and agrochemicals including insecticides, herbicides, and fertilizers. Heavy metal pollution is becoming a major ecological concern due to its potential adverse impact on human health as a result of the consumption of heavy metal-contaminated food products from different levels of the food chain (Dembitsky and Rezanka, 2003; Balkhair and Ashraf, 2016). Crops accumulate heavy metals from contaminated soil, which causes significant damage to plant growth and compromises the safety of agricultural products (Seregin and Ivanov, 2001; Schmidt, 2003; Maksymiec, 2007).
It is well known that the accumulation of heavy metals in plants severely disturbs various physiological and biochemical processes, modifying several metabolic pathways such as mineral nutrient uptake, photosynthesis, respiration, and protein integrity and synthesis (MacFarlane et al., 2003; Maksymiec, 2007; Hasan et al., 2017). The disruption of cellular metabolic activities causes inhibition of plant growth and crop productivity. Plants have evolved various defense mechanisms to cope with heavy metal stress; some can preferentially avoid heavy metal toxicity by regulating heavy metal accumulation at the root level by reducing uptake and increasing exclusion. Furthermore, toxicity due to heavy metal ions in plant cells can be significantly ameliorated by vacuolar compartmentation or binding with various heavy metal chelators such as amino acids, organic acid including humic acid, glutathione (GSH), phytochelatins (PCs), and metallothioneins (MTs) (Cobbett, 2000; Ro et al., 2018). Aditionally, heavy metals lead to the generation of reactive oxygen species (ROS) that damage lipids, proteins, and DNA in plant cells (Yadav, 2010; Tamás et al., 2014). ROS scavenging machinery comprising enzymatic and non-enzymatic systems decreases ROS accumulation and could confer heavy metal tolerance.
Among the heavy metals, Cd and Pb are very harmful to organisms even in small amounts and may result in various malignant diseases in humans (Storelli, 2008; Jaishankar et al., 2014; Xiao et al., 2017). Cd and Pb overload in plants triggers various reactions leading to the induction of oxidative stress, damage to chloroplasts, and reduction of transpiration and photosynthetic rates, resulting in the inhibition of seed germination and stunted plant growth (Aggarwal et al., 2012; Sethy and Ghosh, 2013). In general, heavy metals tend to accumulate primarily in roots and secondarily in other plant organs such as stems and leaves (Fargasova, 2001; Seregin and Ivanov, 2001; Seregin et al., 2004; Gallego et al., 2012), indicating that root vegetables tend to accumulate higher concentrations of heavy metals in the edible portion compared with leafy vegetables. Therefore, to protect human health, it is important to alleviate Cd and Pb accumulation in root vegetables and reduce their toxicity.
Radish (Raphanus sativus L.) is a major root vegetable in the human diet and is widely consumed as the main ingredient of kimchi in Korea (Lee et al., 2015). Therefore, it is imperative to find a potential radish cultivar with a low accumulation of Cd and Pb for safe consumption and to study Cd and Pb tolerance mechanisms to increase the production of safe radishes. A previous study investigated the characteristics of Cd and Pb accumulation under two different concentrations of Cd and Pb in one radish cultivar (Semhi et al., 2014). However, to our knowledge, there are no published reports focusing on Cd and Pb accumulation and its toxic effects on growth and metabolism in various radish cultivars. In this study, four common radish cultivars were exposed to Cd and Pb stress to determine the relationships between Cd and Pb accumulation and their tolerance during germination and seedling growth stage. To avoid side effects such as heterogeneity of natural soils and atmospheric supplies, we used both agar-solidified plant media and hydroponic system. We show that alleviation of Cd and Pb accumulation, low production of hydrogen peroxide (H2O2), and increasing nonprotein thiol (NPT) content are associated with Cd and Pb tolerance in the radish cultivar CR. The results of this work are expected to provide information for safe breeding of the four common radish cultivars under Cd- and Pb-contaminated cultivable land.
Materials and Methods
Plant Materials and Culture Conditions
This study was designed to identify radish cultivars tolerant to Cd and Pb stress, with low Cd and Pb accumulation among the four most popular and widely planted radish cultivars in Korea, namely, ‘Gaulgeojang’ (Asia Seed Co. Korea, AG), ‘Iseogaul’ (L&S Seed Co. Korea, IG), ‘Chongryong’ (Koregon, Korea, CR), and ‘Supertogwang’ (Dongbu Farm Hannong, Korea, ST). The seeds were sterilized with 70% ethanol for 1 min and 5% hypochlorite for 10 min and then thoroughly rinsed with sterilized water. The sterilized seeds were sown in half-strength Murashige and Skoog (MS) medium supplemented with a wide range of CdCl2 (0–0.5 mM ) and PbSO4 (0–1 mM) concentrations and grown at 22°C under long-day conditions (16-h-light/8-h-dark cycle) for 10 d to investigate the effects of Cd and Pb stress on seed germination and seedling growth. The MS plates were placed vertically to determine root growth. The length and fresh weight of the plantlets were measured 6 and 10 d after sowing. Each treatment was replicated three times, with five plants per replicate.
Determination of Cd and Pb Content
Radish seeds were pre-cultivated on moist filter paper for 4 d. Healthy and uniform seedlings were transferred to a container with Hoagland’s nutrient solution and grown hydroponically for 3 weeks. At 3 weeks, they were treated with 0.3 mM CdCl2 and 0.8 mM PbSO4 for 24 h. The plants were thoroughly rinsed with ultrapure water and divided into roots and shoots. The plant material was dried at 60°C and used to determine the Cd2+ and Pb2+ content using an inductively coupled plasma optical emission spectrometer (ICP-OES, Optima-2000 DV, Perkin Elmer, Waltham, MA, USA).
Determination of Nonprotein Thiols (NPTs) and H2O2 Content
Ten-day-old radish seedlings were treated with 0.3 mM CdCl2 and 0.8 mM PbSO4 for 24 h and harvested for NPT and H2O2 assays. The NPT content was extracted by homogenizing 1.0 g of plant material in 4 ml of extract buffer, consisting of 6.3 mM diethylenetriamine pentaacetic acid and 0.1% (v/v) trichloroacetic acid, with a cold pestle and mortar. The extract was centrifuged at 12,000 × g at 4°C for 15 min. The reaction mixture containing 200 µL of supernatant, 200 µL of 1.8 mM 5,5-dithio-2-nitrobenzoic acid that contained 6.3 mM diethylenetriamine penta-acetic acid and 200 mM 4-(2-hydroxyethyl) piperazine-1-propanesulfonic acid (pH 7.8), was recorded at 412 nm (Sneller et al., 2000) with a few modifications to the original method.
The quantity of H2O2 was determined according to a previous study (Kim et al., 2013). The plant materials were extracted with 0.1% trichloroacetic acid and centrifuged at 12,000 × g at 4°C for 20 min. The supernatant was mixed with an equal volume of 100 mM potassium phosphate buffer (pH 7.0) and 1 ml of 1 M potassium iodide. The reaction mixture was incubated in the dark for 1 h at 25°C before recording the absorbance value at 410 nm. H2O2 content was quantified from the standard curve of known concentrations of H2O2.
Statistical Analysis
Data represent mean values with standard deviations of three independent replications. Statistical analysis was conducted using the SigmaPlot 10 software program (Systat Software, Inc., San Jose, CA, USA), and the significant differences among the cultivars were determined using a t-test (p < 0.05).
Results
Effects of Cd and Pb on Germination and Seedling Growth
Under normal growth conditions, the four radish cultivars, AG, IG, CR, and ST, germinated with no noticeable differences and were fully germinated at 2 d. All cultivars displayed similar growth, except AG, which showed slightly slower post-germinative seedling growth until 5 d after germination.
Cd and Pb stress severely retarded both seed germination and seedling growth in all radish cultivars compared with the controls (Figs. 1 and 2). Seed germination and seedling growth of all radish cultivars were similar with Cd concentration below 0.1 mM, but all radish cultivars did not germinate with 0.5 mM Cd (data not shown). In the presence of 0.3 mM Cd, the four radish cultivars germinated in the following order: CR > ST > IG > AG. For example, at 2 d under 0.3 mM Cd stress, the germination rates of CR, ST, IG, and AG were 65%, 55%, 50%, and 10%, respectively (Fig. 1B).
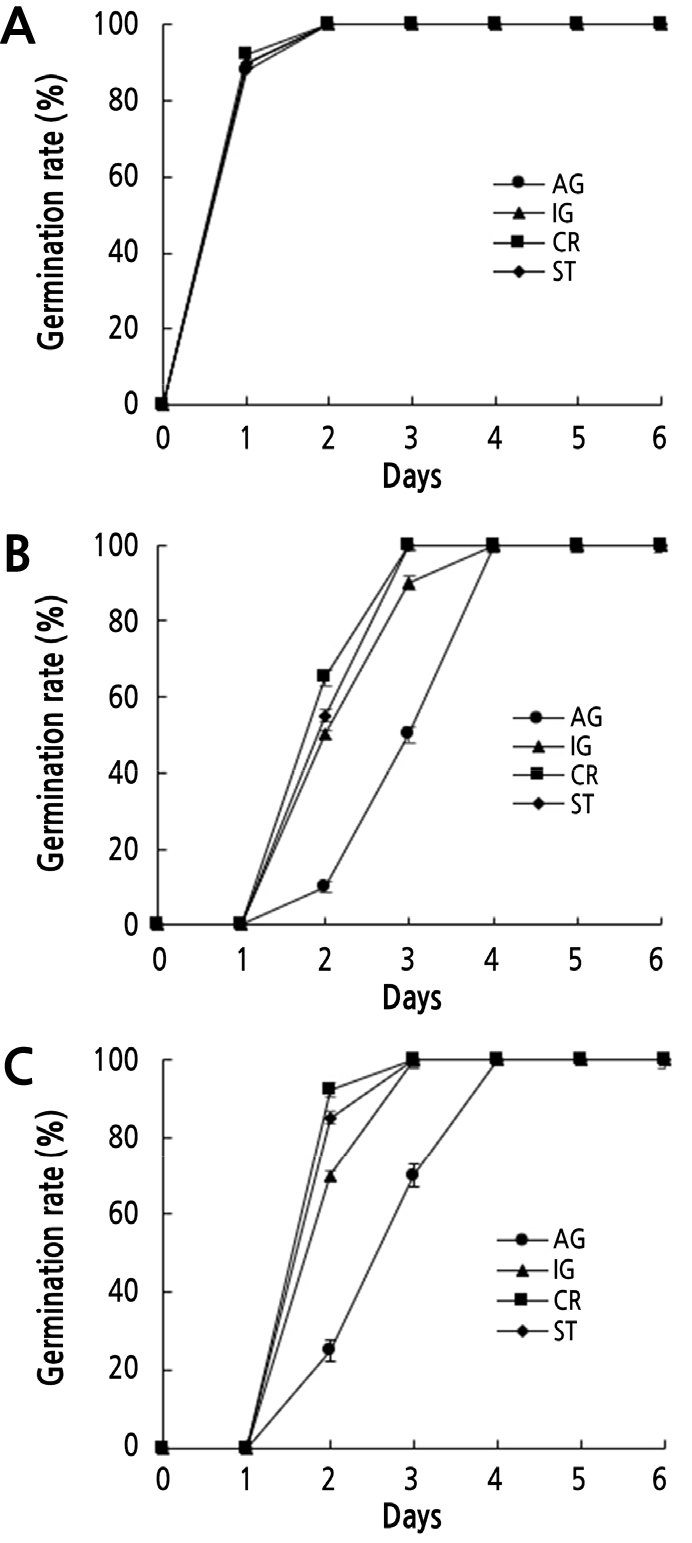
Fig. 1.
Effects of Cd and Pb on the germination of the four radish cultivars. Germination rates of four radish cultivars scored on MS plates (A) containing 0.3 mM CdCl2 (B) or 0.8 mM PbSO4 (C). AG, IG, CR, and ST indicates ‘Gaulgeojang’, ‘Iseogaul’, ‘Chongryong’, and ‘Supertogwang’. Data are presented as the means ± SD of three independent experiments.
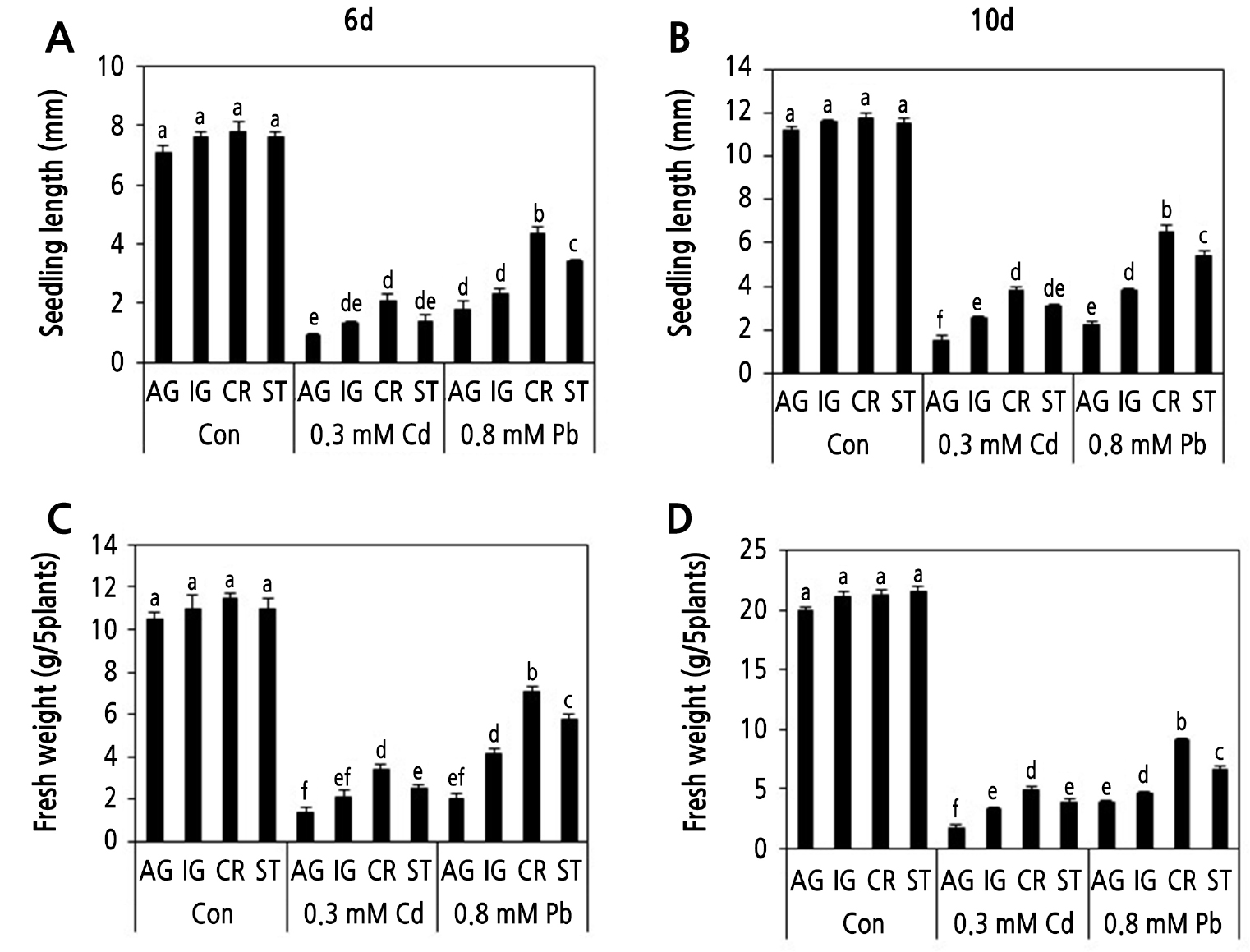
Fig. 2.
Effects of Cd and Pb on the seedling growth of the four radish cultivars. Seedling growth (A, B) and seedling fresh weight (C, D) of four radish cultivars were determined at 6 and 10 d after sowing with 0.3 mM CdCl2 and 0.8 mM PbSO4. AG, IG, CR, and ST indicates ‘Gaulgeojang’, ‘Iseogaul’, ‘Chongryong’, and ‘Supertogwang’. Values are the mean ± SD of three independent experiments. Different lowercase letters above bars within figure indicate significant differences based on Tukey’s multiple comparison at p < 0.05.
Germination rates were similar in all cultivars below 0.5 mM Pb concentration, whereas all cultivars did not germinate when treated with 1 mM Pb (data not shown). At 2 d after sowing under 0.8 mM Pb stress, CR, ST, IG, and AG showed approximately 92%, 85%, 70%, and 25% germination rates, respectively (Fig. 1C). Further, the results for seedling growth and fresh weight under Cd and Pb stress (Fig. 2), were similar to those obtained for the germination rates. In the presence of 0.3 mM Cd, the seedling growth and fresh weight values of CR were approximately 2-fold higher than those of AG at both 6 and 10 d (Fig. 2). However, IG and ST showed similar seedling growth and fresh weight values that were higher than those of AG. Under 0.8 mM Pb stress, CR showed 2- and 3-fold higher seedling growth and fresh weight, respectively, compared to AG at 6 and 10 d (Fig. 2A). ST displayed higher seedling growth and fresh weight values compared to IG under Pb stress. These results collectively indicate that CR is a Cd and Pb stress-tolerant cultivar, and AG is a Cd and Pb stress-sensitive cultivar.
Effects of Cd and Pb Accumulation on Cd and Pb Tolerance
The Cd and Pb contents of both roots and shoots of the four radish cultivar seedlings treated with 0.3 mM Cd and 0.8 mM Pb were measured to ascertain whether Cd and Pb tolerance is associated with the cellular accumulation of Cd and Pb. All four cultivars accumulated significantly higher levels of Cd and Pb in their roots (>100-fold) compared with shoots under the Cd and Pb treatments (Fig. 3). The Cd content in the roots of CR (38 mg·kg-1 DW) was considerably lower than that in the other three cultivars. AG roots displayed the highest Cd accumulation (50 mg·kg-1 DW) (Fig. 3A). IG and ST accumulated similar Cd content in roots. In contrast to roots, Cd accumulation in the shoots of all cultivars was similar (Fig. 3B).
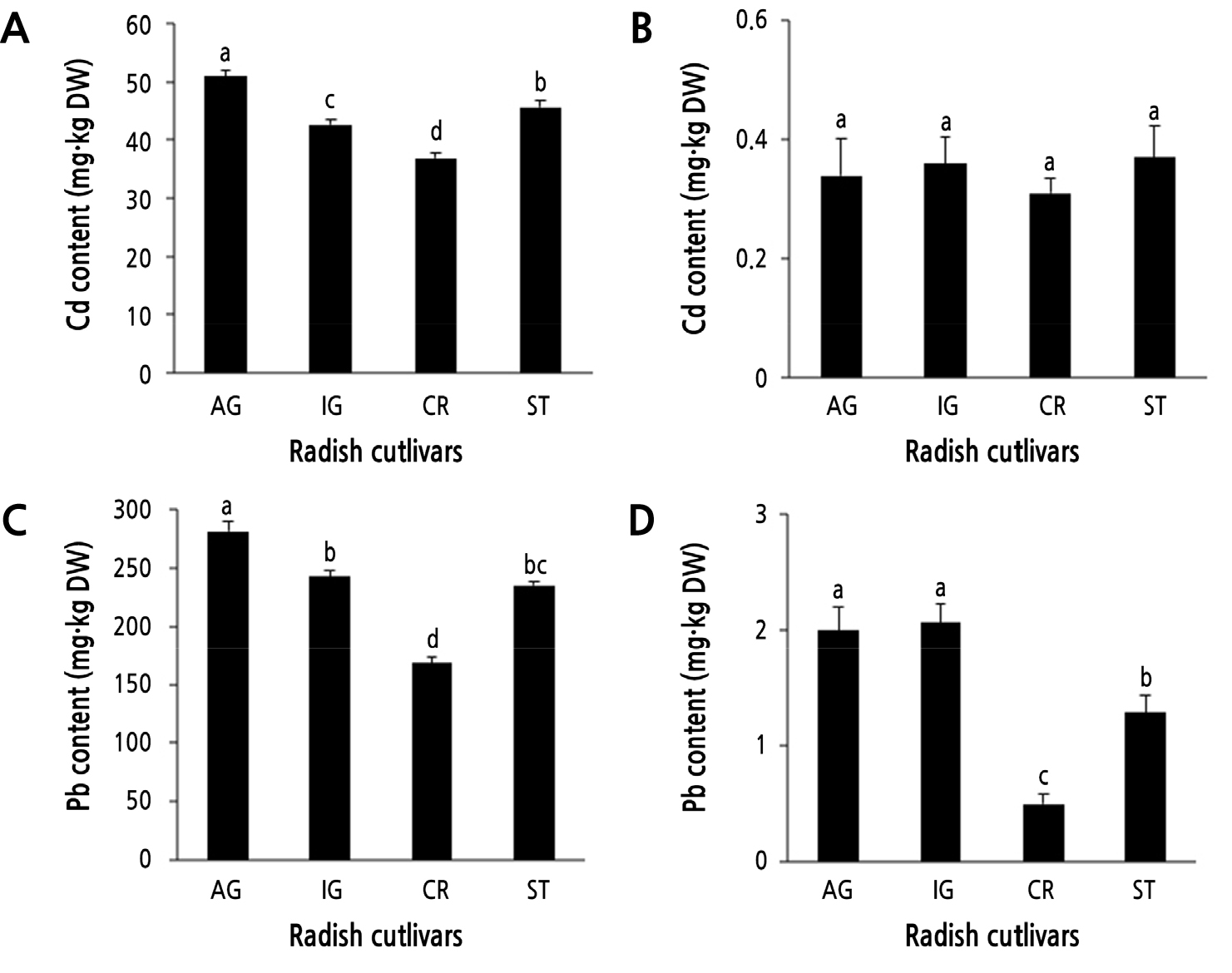
Fig. 3.
Cd and Pb accumulation in the roots (A, C) and shoots (B, D) of the four radish cultivars. Four radish cultivars were grown hydroponically and subjected to 0.3 mM CdCl2 and 0.8 mM PbSO4 for 24 h. AG, IG, CR, and ST indicates ‘Gaulgeojang’, ‘Iseogaul’, ‘Chongryong’ , and ‘Supertogwang’. Data are presented as the means ± SD of three independent experiments. Different lowercase letters above bars within figure indicate significant differences based on Tukey’s multiple comparison at p < 0.05.
Notably, Pb accumulation in the roots of the four cultivars showed similar results to Cd accumulation (Fig. 4C). Among the four cultivars, CR accumulated significantly lower Pb content in its roots compared with the other cultivars, and the roots of AG displayed the highest Pb accumulation (Fig. 4C). IG and ST accumulated similar Pb content in roots. In shoots, the accumulation of Pb in CR was more than 2-fold lower than in the other cultivars. The accumulation of Pb in the shoots of AG and IG was similar and was higher than that in the shoots of ST (Fig. 3D). These results indicate that among the four cultivars, CR accumulates the lowest levels of Cd and Pb.
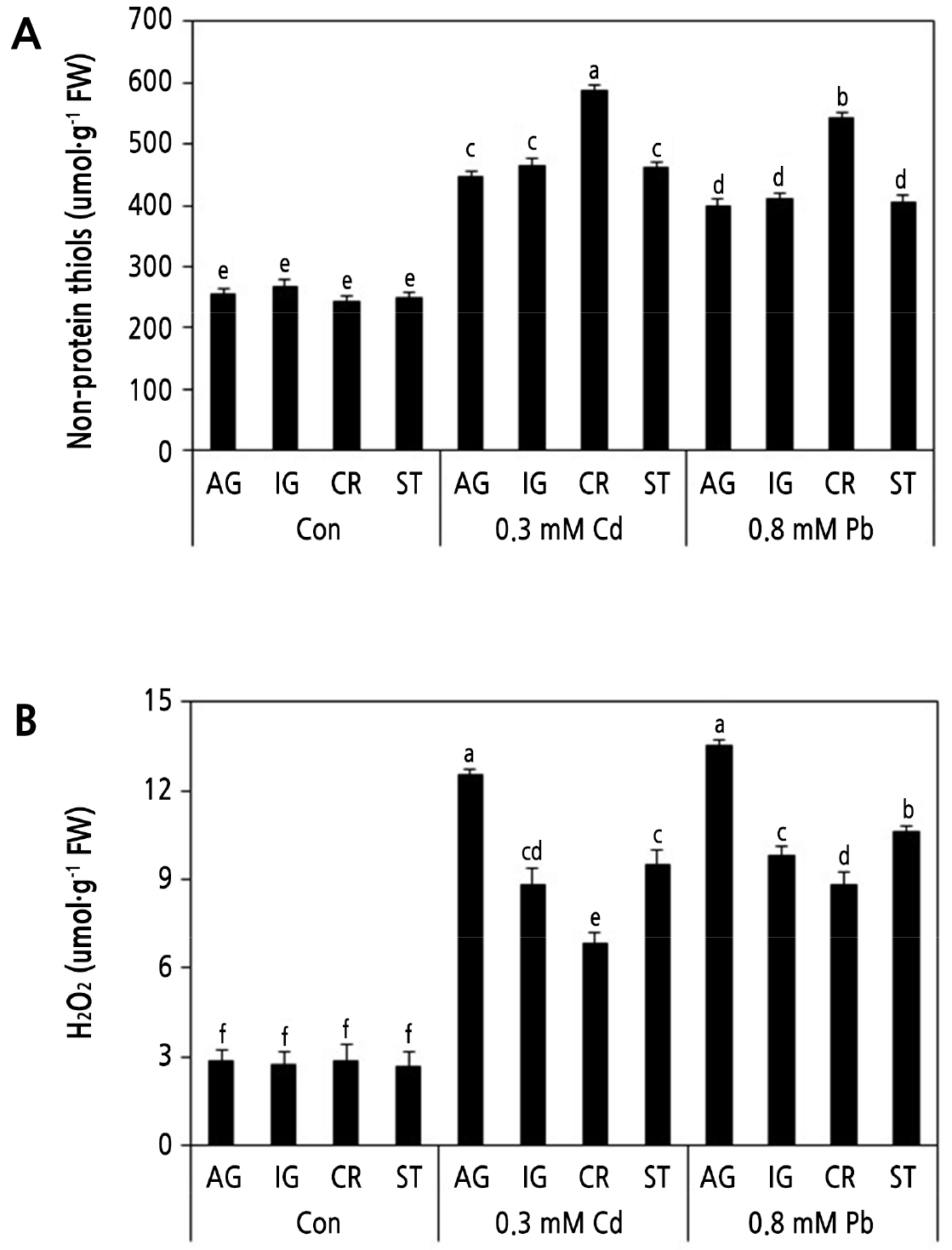
Fig. 4.
Effects of Cd and Pb on the concentrations of nonprotein thiols (A) and H2O2 (B). Four radish cultivars were grown hydroponically and subjected to 0.3 mM CdCl2 and 0.8 mM PbSO4 for 24 h. AG, IG, CR, and ST indicates ‘Gaulgeojang’, ‘Iseogaul’, ‘Chongryong’, and ‘Supertogwang’. Values are the mean ± SD of three independent experiments. Different lowercase letters above bars within figure indicate significant differences based on Tukey’s multiple comparison at p < 0.05.
Effects of Nonprotein Thiols on Cd and Pb Tolerance
It is known that NPTs, including GSH, MTs, and PCs, bind heavy metals and alleviate heavy metal toxicity in plant cells. The response of NPTs under Cd and Pb stress was determined to evaluate their effect on Cd and Pb tolerance of radish seedlings. As expected, compared with the control groups, Cd and Pb significantly increased the NPT content in all four radish cultivars; however, the Cd treatment showed a greater increase in NPT levels than the Pb treatment in all cultivars (Fig. 4A). After Cd and Pb treatment, the NPT levels of CR seedlings were significantly higher than those of the other three cultivars. AG, IG, and ST all displayed similar NPT levels (Fig. 4A).
Effects of Cd and Pb on H2O2 Generation
Oxidative stress plays a significant role in stress symptoms, caused by heavy metals in a variety of organisms. In this study, radish seedlings were exposed to Cd and Pb to determine the relationships between heavy metal-induced oxidative stress and plant growth inhibition.
H2O2 levels were significantly increased (approximately 2–4-fold) in all cultivars after treatment with 0.3 mM Cd and 0.8 mM Pb for 1 d (Fig. 4B). Cd and Pb treatments generated the lowest H2O2 levels in CR and the highest in AG, while IG and ST displayed similar H2O2 generation under Cd and Pb stress. These results indicate that CR could ameliorate Cd- and Pb-induced oxidative damage at the cellular level.
Discussion
Heavy metals in agricultural soil threaten food productivity and human health through their excessive accumulation in edible plant parts. Because plant roots take up heavy metals, it is important to find a heavy metal-tolerant radish cultivar that does not accumulate a high concentration of heavy metals when grown in heavy metal-contaminated soil. Cd and Pb strongly inhibited seed germination, seedling growth, and fresh weight of all four tested radish cultivars in the order of CR < ST < I G < AG (Figs. 1 and 2), indicating that CR is tolerant and AG is sensitive to Cd and Pb stress. Because CR showed strong Cd and Pb tolerance during germination and plant growth compared to the other cultivars (Figs. 1 and 2), our data suggest that CR possesses different adaptation or tolerance mechanisms in response to Cd and Pb stress.
We observed that an excess accumulation of Cd and Pb in roots and shoots of the four radish cultivars grown under the Cd and Pb stress treatment resulted in growth inhibition (Fig. 3). Notably, all four radish cultivars accumulated significantly higher levels of Cd and Pb (>100-fold) in their roots compared to shoots (Fig. 3). Previous studies found that high levels of heavy metal accumulation in roots negatively affected heavy metal tolerance (Fargasova, 2001; Seregin and Ivanov, 2001). The lowest and highest Cd accumulation levels were observed in the roots of CR and AG, respectively, demonstrating that Cd accumulation in radish roots is closely associated with Cd tolerance. It is also likely that the Pb tolerance of the four radish cultivars is closely correlated to Pb accumulation in their roots (Fig. 3C). Interestingly, low Pb concentration was found in both shoots and roots of CR (Fig. 3D), suggesting that Pb transportation from roots to the shoots is inhibited, resulting in the enhancement of CR shoot growth under Pb stress. These results indicate that the low level of Cd and Pb accumulation in CR roots is important for the yield and safe consumption of this radish cultivar grown in Cd- and Pb-contaminated soils.
Excess heavy metals in plant cells disrupt numerous plant functions and metabolic processes in a variety of ways, including the disturbance of the protein structure and functional groups of important cellular molecules as well as the functionality of essential metals in biomolecules such as pigments and enzymes, resulting in the repression of vital events such as photosynthesis, respiration, water balance, and enzymatic activities (Malayeri et al., 2008; Hossain et al., 2012; Ali et al., 2013; Hasan et al., 2017). The thiol groups of NPTs bind heavy metals and play a pivotal role in heavy metal detoxification in plant cells (Yadav, 2010). To determine the possible roles of NPT on Cd and Pb tolerance, we investigated the production of NPTs in the four radish cultivars. NPT levels in all cultivars were significantly increased by Cd and Pb stress, suggesting that the synthesis of NPTs is crucial for cellular responses under Cd and Pb stress. Several previous studies have demonstrated that NPTs are involved in heavy metal tolerance in many plant species. It appears that the relatively higher level of NPTs in CR elevated Cd and Pb tolerance by the chelation of free Cd and Pb ions, thus alleviating Cd and Pb toxicity. It has been reported that complexes of heavy metal chelators such as PCs and GSH with heavy metals in the cytosol subsequently facilitate their transport and sequestration in cell vacuoles, thus limiting the circulation of free Cd in the cytosol (Mishra and Dubey, 2006; Yadav, 2010). Therefore, it could be proposed that NPT-Cd or NPT-Pb complexes could be efficiently transported to the cell vacuoles, resulting in reduced Cd and Pb toxicity in CR radish cells. Further investigation is needed to determine the destiny of NPT complexes with Cd and Pb in the CR cytosol.
Furthermore, heavy metals elevate the generation of ROS in plant cells, causing multiple disorders such as the oxidation of proteins and lipids and the denaturation of cell structures and membranes (Maksymiec, 2007; Flora et al., 2008; You and Chan, 2015). Cd and Pb likely stimulate the formation of H2O2 in the four radish cultivars, thereby inhibiting seed germination and seedling growth by the impediment of these metabolic processes (Fig. 4). H2O2 levels were significantly increased in AG and reduced in CR under Cd and Pb stress; these results suggest that CR possesses efficient ROS scavenging ability via enzymatic or non-enzymatic antioxidant systems. It would be interesting to study the antioxidant system response to understand the Cd and Pb tolerance mechanisms.
In conclusion, these results indicate that the Cd- and Pb-tolerant cultivar CR inhibits Cd and Pb accumulation and activates physiological responses such as NPT production and H2O2 scavenging. As root vegetables are increasingly produced in heavy metal-contaminated soils, our results could lay the foundation for breeding radish cultivars with low Cd and Pb uptake. Moreover, it is important to find radish cultivars with low accumulation of a broader range of heavy metals. Further studies are needed to evaluate and screen other edible root and leafy vegetables for heavy metal content to guide consumers on the safe consumption of these vegetables.