Introduction
Materials and Methods
Plant Material and Growth Conditions
Treatments with Beneficial Elements
Measurements of Growth Parameters
Contents of Leaf Photosynthetic Pigments
Statistical Analyses
Results
Effect of Beneficial Elements on Seedling Growth
Effect of Beneficial Elements on Biomass Production
Effect of Beneficial Elements on Leaf Photosynthetic Pigments
Correlation Among Parameters by Effect of Beneficial Elements
Discussion
Conclusions
Introduction
When supplied in small doses, the beneficial elements have positive effects on plants, such as promoting growth and development, stimulating resistance and tolerance mechanisms to biotic and abiotic stress factors, improving the use of other nutrients, and compensating for or remedying toxic effects caused by other elements (Pilon-Smits et al., 2009). The beneficial effects of these elements are determined by a series of factors, such as the concentration and speciation of the element, the plant species, the growth phase, the method of application (foliar or rooting), and the exposure time, all of which play an important role at the time of use (León-Morales et al., 2019). Elements that have been described as beneficial for plants include iodine (I), lanthanum (La), titanium (Ti), aluminum (Al), cobalt (Co), sodium (Na), selenium (Se), silicon (Si), cerium (Ce), and vanadium (V) (Gómez-Merino and Trejo-Téllez, 2018).
Vanadium is a transition metal that was initially classified as a highly toxic element for plants. However, low concentrations of this element can positively affect plant growth (Larsson et al., 2013). The toxicity of V is associated with its different oxidation states (+2, +3, +4, and +5), with the pentavalent form (+5) being the most toxic and its tetravalent form (+4) the least toxic (Akoumianaki-Ioannidou et al., 2016). In pennyroyal plants (Mentha pulegium L.), an increase in the dry weight of the roots was observed with doses of 340 µM V (Akoumianaki-Ioannidou et al., 2015). In tomato (Solanum lycopersicum L.) and bell pepper (Capsicum annuum L.) plants, doses of 3, 5, and 10 µM promoted the growth of both roots and shoots as well as the dry biomass of the seedlings (Saldaña-Sánchez et al., 2019).
Selenium is an essential element for mammals and some microorganisms (Hernández-Díaz et al., 2020). In plants, Se is considered a beneficial element and is found in four different oxidation states in the soil: selenate (SeO42-), selenite (SeO32-), Se elemental (Se0), and selenide (Se2-); selenate and selenite are the most common and available species for plants (Li et al., 2015). The application of Se in fava bean plants (Vicia faba L.) along with lead (Pb) induced oxidative stress and increased the content of chlorophyll a and b and carotenoids (Mroczek-Zdyrska et al., 2017). The application of Se in turnip plants (Brassica rapa L.) increased seed production by 43% (Lyons et al., 2009). In addition, the application of Se increased the yield of fresh biomass, leaf area, and quality of strawberry (Fragaria × ananassa Duch.) fruit (Mimmo et al., 2017) as well as the height of the seedlings and the growth of the roots of serrano bell pepper (Capsicum annuum L.) and radish (Raphanus sativus L.) plants (León-Morales et al., 2019).
Cerium is a rare earth metal that is present in two oxidation states: +3 and +4 (Rajeshkumar and Naik, 2018). In agriculture, Ce has been documented to have beneficial effects on plants (Ramírez-Olvera et al., 2018). For example, Hong et al. (2002) reported that Ce application led to the increased growth of spinach (Spinacia oleracea L.) plants as well as the proportion of chlorophyll. In addition, Ce has been shown to affect the germination rate, germination index, and vigor index of rice (Oryza sativa L.) seeds (Fashui, 2002). The application of Ce has also been correlated with greater growth, root volume, biomass weight, yield, total sugar content, and nutritional state of several cultivations (Hu et al., 2002; Ramírez-Olvera et al., 2018). In addition, the use of Ce nanoparticles has been suggested to increase the height, photosynthetic pigments, and antioxidant activity in marigold (Calendula officinalis L.) plants (Jahani et al., 2019).
Although I is essential for animals and accumulates strongly in seaweed, this element is considered beneficial for higher plants. In soil, I can be found in inorganic forms, such as iodide (I-) and iodate (IO3- ions), or in organic form, such as methyl iodide (CH3I) (Medrano-Macías et al., 2016). Positive effects of I have been described for celery (Apium graveolens L.) (Dai et al., 2004), lettuce (Lactuca sativa L.) (Smolen et al., 2011), and radish (Strzetelski et al., 2010). In contrast, high concentrations of I have been observed to induce biomass losses in potato (Solanum tuberosum L.) (Mao et al., 2014) and tobacco (Nicotiana tabacum L.) (Caffagni et al., 2011) and to cause phytotoxic symptoms, including chlorosis and necrosis, in lettuce (Lawson et al., 2015).
Marigold is an annual herbaceous plant belonging to the family Asteraceae. It is native to the Mediterranean area and currently occurs in various parts of the world because of its adaptability. Aside from being cultivated for ornamental purposes, it has many other uses because of its chemical composition, which includes terpenes, flavonoids, tannins, coumarins, and essential oils (Ashwlayan et al., 2018). Consequently, marigold is a valuable plant for the cosmetic, food, and pharmaceutical industries (Bragueto et al., 2019). Despite being a species of ornamental and industrial importance, little research has assessed the ability of different elements to promote its growth, development, and quality.
The aim of this study was to evaluate the effects of different concentrations of V, Se, Ce, and I on the growth, biomass accumulation, and concentration of photosynthetic compounds in marigold plants. Our findings could be used to obtain better quality seedlings and ensure the success of marigold cultivation.
Materials and Methods
Plant Material and Growth Conditions
A commercial variety of marigold (Calendula officinalis) (Hortaflor, Mexico) was used; the seeds were sown in flexible polyethylene germination trays with 98 cavities with commercial substrate for germination consisting of peat moss (0-7 mm fiber), wetting agent, dolomite, and NPK fertilizer (20-10-18, 1 kg per 1,000 L of substrate). The trays with the seeds were kept in the dark for 5 days. Afterwards, the experiment was conducted in a greenhouse with a plastic cover under natural light (509 W/m2 in average), with a 12-h photoperiod. The average temperatures during the day and night were 31°C and 16°C, respectively, and the relative humidity ranged from 42-69% (Onset, MX2301, USA).
Marigold seedlings were watered with tap water four days a week and three days a week with 50% Hoagland nutrient solution [0.5 mM (NH4) H2PO4, 2. 5 mM Ca (NO3)2 4H2O, 1 mM MgSO4 7H2O, 3 mM KNO3, 10 µM Fe-EDTA, and microelements (0.1 µM Na2MoO4 2H2O, 0.3 µM CuSO4 5H2O, 0.77 µM ZnSO4 7H2O, 9.1 µM MnCl2 4H2O and 46 µM H3BO3]. Distilled water was used for the preparation of the nutritive solution, and the pH was adjusted to 5.5 using NaOH or HCl.
Treatments with Beneficial Elements
Three concentrations of NH4VO3 (3, 5, and 10 µM V), Na2SeO3 (5, 10, and 20 µM Se), and CeCl3 (25, 50, and 100 µM Ce) and two concentrations of KI (5 and 10 µM I) were evaluated, in addition to the control (0 µM). All of the beneficial elements used were reactive grade (Sigma-Aldrich). The application of treatments began 20 days after sowing by supplying weekly irrigations applied to the root together with the nutritive solution for 26 days, with a total of four applications. Three 98-cavity trays were used per treatment. A completely randomized experimental design was used.
Measurements of Growth Parameters
The plants were measured at the seedling stage. Plant height was measured from the base of the plant to the apex of growth, and the root length from the base of the plant to the tip of the largest root. The root volume was established by the displacement of water with a 50-mL graduated glass probe. The stem diameter was measured at the base of the plant with digital Vernier calipers (Surtek, Urrea, Mexico). The number of leaves per plant was determined. The leaf area was also estimated by digitizing the leaves of each plant in a scanner (MPC3055P Lanier, Ricoh, USA) and measuring the leaf area with Image Processing and Analysis in Java (ImageJ). The weight of fresh biomass from roots, stems, and leaves was determined; the weight of dry biomass was obtained after drying the tissue in an oven (Yamato Scientific, DX402C, Japan) at 70°C for 48 h. Ten plants were measured per tray; the exception was for leaf area, where only five plants were measured per tray.
Contents of Leaf Photosynthetic Pigments
The fourth leaf from three plants was selected per treatment. The tissue was cut into small segments; 100 mg of fresh leaves was weighed and then immediately placed in 5 mL of acetone (80%, v/v). The samples were then placed at 4°C for 24 h. Chlorophyll and carotenoid concentration was determined following the methods of Arnon (1949) and Davies (1976). The absorbance of the supernatant was measured in a spectrophotometer (Thermo Scientific™, GENESYS 10uv, USA) at 645, 663, and 480 nm following the procedure of Saldaña-Sánchez et al. (2019).
Statistical Analyses
Analysis of variance was performed, and the difference between means was evaluated by Duncan's multiple range tests (p < 0.05). A Pearson's correlation analysis was performed using the SAS 9.1 statistical package (SAS Institute, Cary NC, USA). The heat map was created in Microsoft Excel (Microsoft Office Professional Plus, 2010).
Results
Effect of Beneficial Elements on Seedling Growth
The beneficial effects of V, Se, and Ce on the growth of marigold seedlings were reflected by increases in plant height under the concentrations of 5 and 10 µM V, 5 and 20 µM Se, and 50 and 100 µM Ce relative to the control. The two I treatments did not result in statistically significant increases in plant height. The concentration of 25 µM Ce reduced the height of marigold plants by 9.2% (Fig. 1).
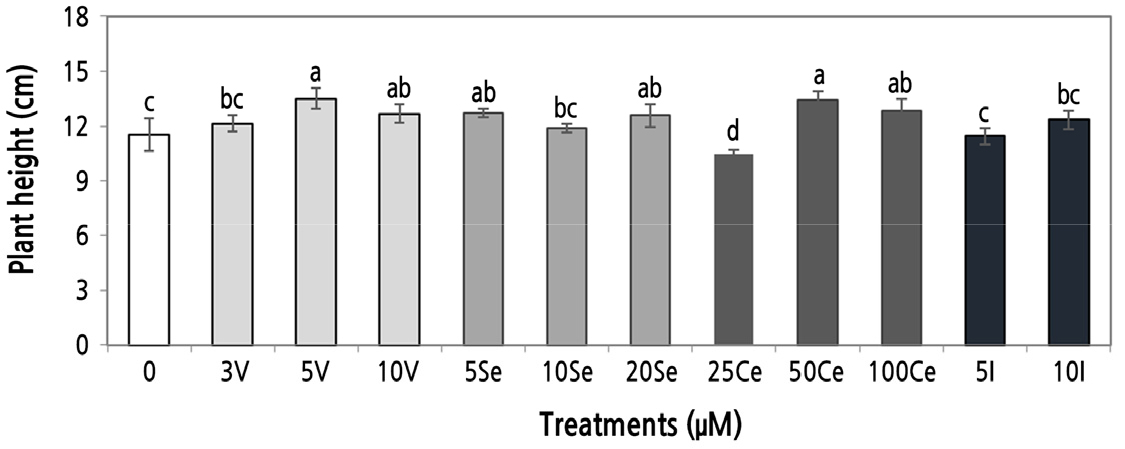
Fig. 1.
Marigold seedling height. The seedlings were watered with different concentrations of vanadium (V), selenium (Se), cerium (Ce), and iodine (I). Mean values ± SD. Different letters in each element section denote statistically significant differences according to Duncan's test (α = 0.05), p < 0.0001.
Most treatments did not significantly differ from the control in root length. In addition, a negative effect on root length was observed for the three concentrations of V and the highest concentrations of Ce (100 µM) and I (10 µM) (Table 1). The root volume did not significantly differ from the control under all of the treatments of V and 50 µM Ce; for the rest of the treatments, a reduction in root volume was observed relative to the control. There were no significant differences observed in the diameter of the stem between all of the treatments evaluated (Table 1).
Table 1.
Root length, root volume, and stem diameter of marigold seedlings with weekly applications of different concentrations of vanadium (V), selenium (Se), cerium (Ce), and iodine (I)
The leaf area increased under the three concentrations of V, 20 µM Se, and 100 µM Ce; the rest of the treatments did not significantly differ from the control. Treatments with 3 and 10 µM V, 20 µM Se, three concentrations of Ce, and 5 µM I showed an increase in the number of leaves per marigold seedling relative to the control. Specifically, 5 µM I and 10 µM V produced 28.2 and 20.5% more leaves than the control, respectively (Fig. 2).
Effect of Beneficial Elements on Biomass Production
The dry root biomass in marigold seedlings was greater under the application of 3 µM V; the rest of the treatments did not significantly differ from the control. The dry stem biomass of the seedlings followed a similar trend to that observed for dry root biomass; the use of 3 µM V increased the dry stem biomass by 62.5% compared with the control, and treatment with 5 µM I was also significantly higher than the control. The rest of the treatments evaluated did not significantly differ from the control. The percentage of dry leaf biomass of 20 µM Se, 100 µM Ce, and 5 µM I increased by 44.4, 41.6, and 39.4%, respectively, relative to the control. The dry leaf biomass increased as the dose of Ce increased; specifically, from 25 to 100 µM Ce, there was an increase from 11.1 to 41.6% in the dry weight of leaves (Fig. 3).
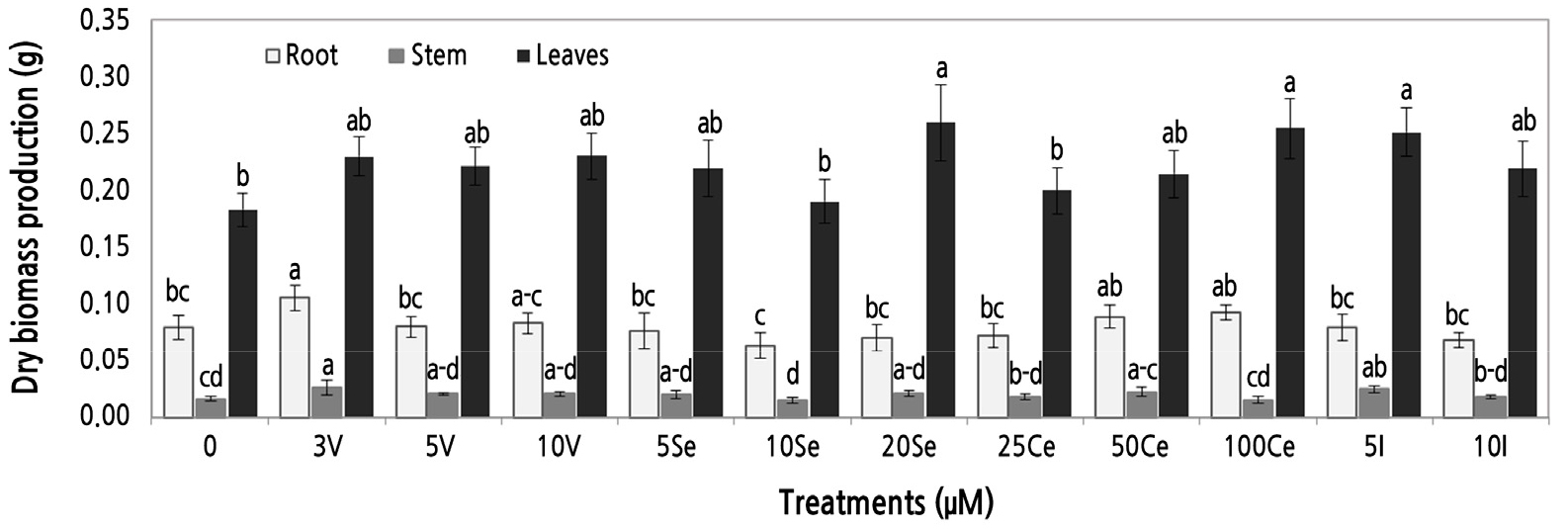
Fig. 3.
Dry biomass of the root, stem, and leaves of marigold seedlings watered with different concentrations of vanadium (V), selenium (Se), cerium (Ce), and iodine (I). Mean values ± SD. Different letters in each element section denote statistically significant differences according to Duncan's test (α = 0.05), root: p < 0.05, stem: p < 0.05, leaves: p < 0.05.
Effect of Beneficial Elements on Leaf Photosynthetic Pigments
The evaluated treatments did not show significant changes in the chlorophyll concentration relative to the control; however, 25 µM Ce had a negative effect and led to decreases of 16.6 and 17.0% in the content of chlorophyll a and total chlorophyll, respectively. Chlorophyll b, total chlorophyll, and carotenoids responded positively to the application of 10 µM V and showed increases of 35.1, 20.5, and 20.4%, respectively, compared with the control. The photosynthetic pigments at all doses of Se and I did not significantly differ from the control (Table 2).
Table 2.
Chlorophyll and carotenoid content in leaves of marigold seedlings watered with different concentrations of vanadium (V), selenium (Se), cerium (Ce), and iodine (I)
Correlation Among Parameters by Effect of Beneficial Elements
The correlation analysis revealed significant and highly significant correlations between growth and biomass production parameters, whereas photosynthetic pigments were weakly correlated with the rest of the variables. Specifically, there was a highly significant correlation between the leaf area (LA) and all of the evaluated variables, including photosynthetic pigments. There was a negative correlation between root length (RL) and chlorophylls a (Chla) and b (Chlb), total chlorophyll (Chlt), and carotenoids (Car); however, only the correlation between RL and Chlb was significant. A highly significant positive correlation was also observed between chlorophylls and carotenoids (Fig. 4).
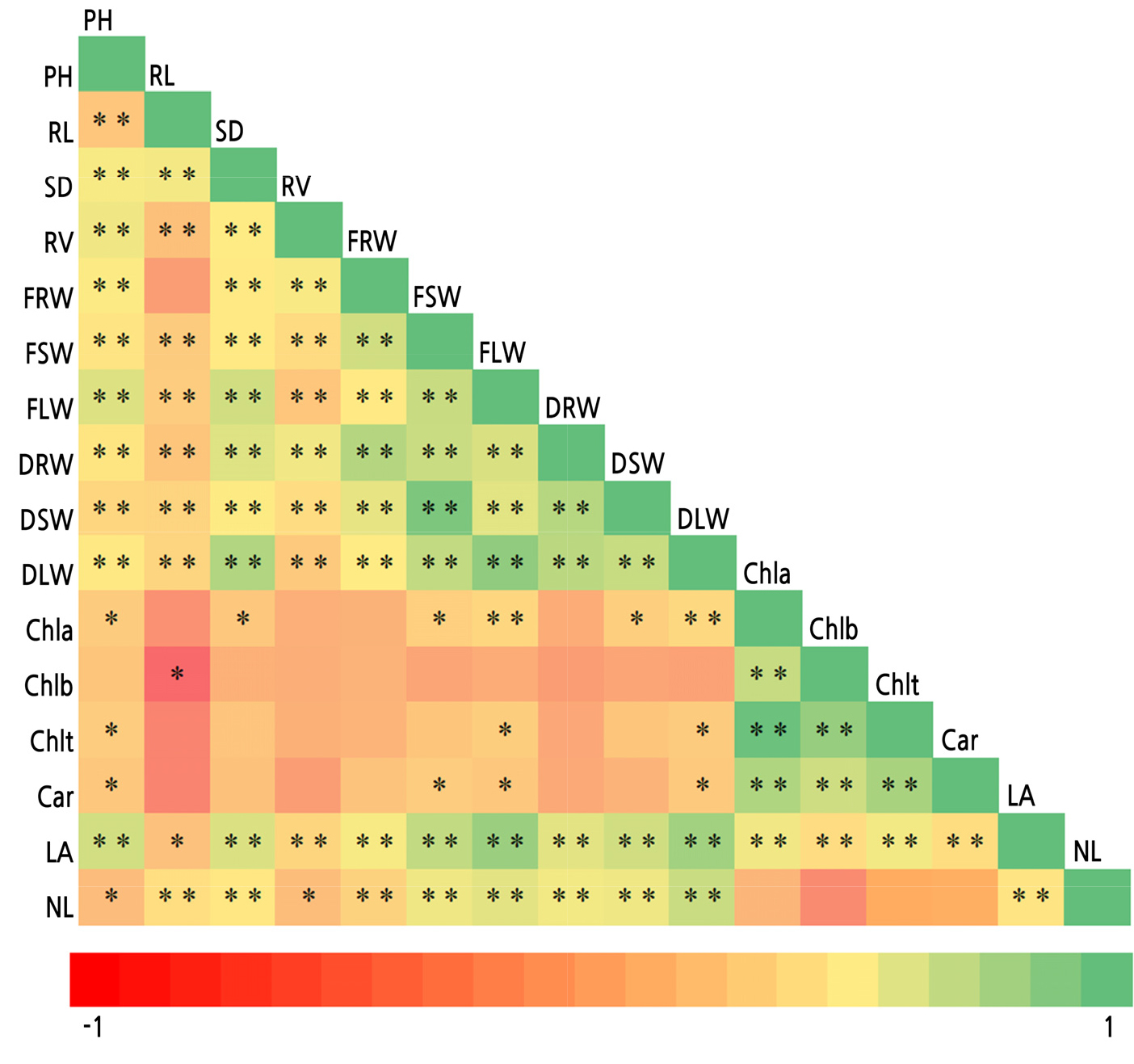
Fig. 4.
Pearson’s correlations between the growth, biomass production, and photosynthetic pigment parameters of marigold seedlings in response to the application of vanadium (V), selenium (Se), cerium (Ce), and iodine (I). PH: plant height, RL: root length, SD: stem diameter, RV: root volume, FRW: fresh root weight, FSW: fresh stem weight, FLW: fresh leaf weight, DRW: dry root weight, DSW: dry stem weight, DLW: dry leaf weight, Chla: chlorophyll a, Chlb: chlorophyll b, Chlt: total chlorophyll, Car: carotenoids, LA: leaf area, NL: number of leaves. *p < 0.05, **p < 0.01.
Discussion
Currently, marigold production faces several challenges ranging from nutrient deficiency to biotic and abiotic factors that limit overall plant development. Beneficial elements such as V, Se, Ce, and I provide an alternative method for helping plants achieve greater height, leaf area, and dry biomass production of the root, stem, and leaf. The growth of the seedlings was enhanced under the application of 5 µM V (Fig. 1). Saldaña-Sánchez et al. (2019) applied the same concentration of V and observed an even greater growth of tomato and bell pepper plants. In addition, an increase in the foliar area of marigold plants at the three concentrations of V was observed. These results are inconsistent with previous findings in chili plants, where the foliar area of 5 and 10 µM V treatments did not significantly differ from the control (García-Jiménez et al., 2018). The positive effect of V on plant growth may stem from the synergistic effect of V with nitrogen metabolism, as V participates in the accumulation of nitrogen in plants (Grzanka et al. 2020).
The dry biomass of marigold root and stem was higher in seedlings treated with 3 µM V (Fig. 3). In pennyroyal, concentrations from 0 to 340 µM V stimulated the accumulation of dry root biomass (Akoumianaki-Ioannidou et al., 2016). In contrast, dry biomass and root length in rice can be reduced under concentrations of 128-590 µM V (Altaf et al., 2020). This finding can be attributed to mitotic cell division, which could restrict root tip development. Similarly, doses of 1.7-8.54 µM V have been reported to reduce fresh and dry root and shoot biomass of lettuce plants (Gil et al., 1995). In general, the application of 10 µM V increased leaf area (Fig. 2A), the number of leaves, and the amount of chlorophyll b, total chlorophyll, and carotenoids (Fig. 2B and Table 2) in marigold seedlings. In contrast, García-Jiménez et al. (2018) found that 5 µM V increased plant height and the number of leaves and flowers and induced chlorophyll synthesis in bell pepper. Thus, the effect of V depends on its concentration and plant species.
There are three possible ways in which V might influence the chlorophyll content. Firstly, V might act as an inducer at one or more sites in the chlorophyll biosynthetic pathway; secondly, V might have a synergistic effect on the bioavailability of Fe and Mg and support chlorophyll biosynthesis; and thirdly, V might prevent the degradation of chlorophylls by affecting certain enzyme systems in plants (Aihemaiti et al., 2020). There is currently no information on the effect of V on marigold plants. Nevertheless, V has been used as an elicitation strategy in in vitro cultures for the synthesis of secondary metabolites (Siatka and Kasparová, 2007). Therefore, the use of V during marigold culture could contribute to the biosynthesis of a greater number of bioactive compounds that are naturally found in low concentrations. This property could be exploited by the food industry in fortified foods, such as yogurt (Bragueto et al., 2019).
In this study, 5 and 20 µM Se resulted in increases in the height of marigold seedlings, which might be related to the capacity of Se to improve the antioxidant activities in plants and thus improve their growth (Boldrin et al., 2016). At the same time, 20 µM Se resulted in greater leaf area of marigold seedlings (Fig. 2A). This finding is consistent with Mimmo et al. (2017), who used concentrations of 0, 10, and 100 µM Se to achieve a higher percentage of leaf area in strawberry plants. Likewise, a positive effect was observed under the application of 20 µM Se on the dry biomass of marigold leaves (Fig. 3). Similar results were observed in turnip (Brassica rapa), where 2.89 µM promoted the accumulation of dry leaf biomass (Li et al., 2018). In some cases, the positive effect of Se is attributed to an increase in the antioxidant activity of plant tissues as well as to the control of reactive oxygen species (ROS) originating from photosynthesis (Thavarajah et al., 2015). In contrast, Se had negative effects on root volume, which might stem from morphological changes in the root system and thus negatively affect the efficiency of water and nutrient uptake (Hartikainen et al., 2001).
The three concentrations of Se did not significantly differ from the control in the concentration of chlorophylls and carotenoids (Table 2). In contrast, with doses of 200 µM of Se, the content of these photosynthetic pigments in Ulva australis increased greatly; this effect was related to Se toxicity, suggesting that it might be a mechanism of tolerance for this type of toxicity (Schiavon et al., 2016). However, D’Amato et al. (2018) noted that low concentrations of Se could have a positive effect on chlorophyll and carotenoid biosynthesis. To date, there are no studies that have examined the possible effects of Se on marigold production. One study reported a low Se content in marigold plants (Sotek et al., 2018); thus, studies on Se supplementation would be highly relevant to this plant species.
The application of 50 and 100 µM Ce in marigold increased the height of the seedlings (Fig. 1). In other crops, doses of 50 and 100 µM Ce increased the height of tomato plants by 15% (Saldaña-Sánchez et al., 2019). In marigold roots, 100 µM Ce reduced root length and root volume (Table 1). Similarly, Ramírez-Olvera et al. (2018) indicated that doses of 100 µM Ce affected root length in rice. In contrast, root growth inhibition caused by Ce might be attributed to various factors, such as loss of cell turgor or decreased cell wall extensibility, which might be related to deficiencies in certain enzymes involved in energy utilization (Shyam and Aery, 2012). D’Aquino et al. (2009) attributed the reduction in roots after Ce treatment to a low rate of cell division.
However, 25 µM Ce reduced the chlorophyll a and total chlorophyll content of marigold seedlings (Table 2). These results are consistent with those of Zicari et al. (2018) for water lentil (Lemna minor L.), where concentrations of 500 µM Ce decreased the concentration of chlorophyll a and b. This pattern might stem from the fact that high concentrations of Ce can be associated with losses in the proportion of chlorophyll a induced by the activation of chlorophyllase, an enzyme responsible for the degradation of chlorophyll (Shyam and Aery, 2012). In the case of marigold, information is lacking on the possible effect that this element might have on photosynthetic parameters, given that these are smaller doses than those reported by Zicari et al. (2018) However, there is evidence that the use of Ce nanoparticles at concentrations of 290-581 µM increased the fresh and dry biomass of leaves as well as the foliar area and chlorophyll content in marigold plants (Jahani et al., 2019). Although Ce doses were higher than the concentrations used in this study in this last case, nanoparticles have drastically different effects, including reduced toxicity, compared with ionic forms of elements (Hernández-Díaz et al., 2020).
Finally, with the treatment of 5 µM I, marigold plants developed a greater number of leaves (Fig. 2B). In contrast, 10 µM I had a negative effect on root length and root volume (Table 1). Consistent with these results, Zhu et al. (2003) reported that concentrations greater than 10 µM I had detrimental effects on spinach plant growth. This phenomenon has been suggested to occur because of the excessive accumulation of I in plant tissues. In contrast, a synergistic effect was recently reported using 10 µM I combined with 0.1 µM V in corn plants, which led to an increase in plant height by 12% (Grzanka et al., 2020). The application of 5 µM I significantly increased the dry biomass of the stem and leaves (Fig. 3). Similar results were observed for dried cabbage leaf biomass, but these data contrast with data from tomato where no significant differences were observed under concentrations of 0.4-2.33 µM I (Dobosy et al., 2020). This finding is also consistent with results obtained in marigold at concentrations of 10 µM I (Fig. 3). In marigold, no studies to date have established beneficial or harmful effects of I application during its cultivation.
Conclusions
The results of this study indicate that the application of low concentrations of I, Se, and V, as well as high concentration of Ce, had a positive effect on the growth, biomass accumulation, and content of photosynthetic compounds during the initial stage of marigold growth. Low doses of these beneficial elements could be applied throughout the crop cycle to improve the growth of marigold plants, as well as to promote the biosynthesis of bioactive compounds. To our knowledge, this is the first study to examine the use of beneficial elements such as V, Se, and I in marigold, a species of great importance to the cosmetic, food, and pharmaceutical industries.