Introduction
Materials and Methods
Total RNA extraction and qRT-PCR for gene expression analysis
Phenylpropanoid extraction and HPLC analysis
Statistical analysis
Results
Phenylpropanoid content in different developmental stages of Agastache rugosa
Expression levels of phenylpropanoid-related genes at different developmental stages of Agastache rugosa
Conclusions
Introduction
Agastacherugosa O. Kuntze, commonly known as Korean mint, is a medicinal perennial plant belonging to the Lamiaceae family (Lee et al., 2008; Zielinska et al., 2014; Carmona-Castro et al., 2019). This botanical species is native to East Asia, particularly Korea, China, and Japan. It has a rich phytochemical profile and potential pharmacological properties (Ahn and Yang, 1991). Research on the phytochemistry of the plant has revealed several compounds, including phenylpropanoids, flavonoids, lignans, and terpenoids, each with useful biological properties. The essential oil of the herb contains volatile compounds that have been reported to show biological activity (Oh et al., 1996; Haiyan et al., 2016; Lee et al., 2017).
Polyphenolic compounds present in A. rugosa, including rosmarinic acid (RA), chlorogenic acid, ferulic acid, and caffeic acid, along with their derivatives, have been associated with various potential health benefits based on recent scientific studies, such as exhibiting antioxidant and anti-inflammatory properties (Hakkim et al., 2007). In A. rugosa, rosmarinic acid has been identified as the primary active component. This compound is structurally characterized as an ester derived from caffeic acid and 3,4-dihydroxyphenyllactic acid, as evidenced by a chemical analysis. The main activities of rosmarinic acid include its astringent properties, antiviral effects, and antimutagenic ability, which are achieved by modulating cytokine production and inhibiting inflammatory pathways, as well as its potential neuroprotective and antioxidant properties (Ellis and Towers, 1970; Parnham and Kesselring, 1985; Cuvelier et al., 1996; Chen and Ho, 1997; Takeda et al., 2002).
A. rugosa also contains several flavonoids, including tilianin, acacetin, quercetin, apigenin, and luteolin (Oh et al., 1996; Nam et al., 2006; Hong et al., 2001). Tilianin is a flavonoid glycoside compound present in certain plants and is typically present in plants of the Lamiaceae family, which consists of herbs such as Agastache rugosa. Acacetin is known for its antioxidant, anti-inflammatory, antiviral, and anticancer properties (Hong et al., 2001; Nam et al., 2006). The bioactivity of acacetin can be attributed to its ability to modulate various cellular pathways and to interact with enzymes and receptors (Kim et al., 2015). Agastache rugosa contains large amounts of essential oils, resulting in its unique aroma and potential health advantages. These oils are typically found in plant leaves and flowers. The essential oils of Agastache rugosa contain various volatile compounds, including monoterpenes (e.g., limonene and myrcene), sesquiterpenes (e.g. β-caryophyllene), and phenolic compounds (e.g., thymol and carvacrol) (Hong et al., 2022). The terpenoids in Agastache rugosa, such as limonene, myrcene, and β-caryophyllene, contribute to the aroma of the plant and may also have potential health benefits. The volatile compounds present in Agastache rugosa are responsible for its distinctive scent. These compounds may affect the nervous system and contribute to the traditional use of the plant as a sedative (Hong et al., 2022).
The typical compounds found in Agastache rugosa include rosmarinic acid, tilianin, and acacetin. These compounds are synthesized via pathways involving phenylalanine-(phenylpropanoids) and tyrosine-based branches (Yeo et al., 2021). The phenylpropanoid biosynthesis pathway for the synthesis of rosmarinic acid, tilianin, and acacetin in plants is depicted in Fig. 3. Phenylalanine is converted to caffeoyl-CoA, which reacts with 3,4-dihydroxyphenyllactic acid to form RA. The enzymes involved include phenylalanine ammonia-lyase (PAL), cinnamate 4-hydroxylase (C4H), hydroxycinnamoyltransferase (HCT), and rosmarinic acid synthase (RAS) (Yeo et al., 2021). Tilianin is a flavonoid glycoside synthesized from p-coumaroyl-CoA, which is converted to chalcone-by-chalcone synthase (CHS) and then to flavone by chalcone isomerase (CHI). In the subsequent stage, the enzyme flavone synthase (FS) facilitates the desaturation of naringenin, resulting in the formation of apigenin (Martens and Mithöfer, 2005). This is followed by the action of apigenin 4′-O-methyltransferase (A4OMT), which introduces a methyl group onto apigenin, producing acacetin (Kuroki and Poulton, 1981). Thus, the enzyme glucosyltransferase (GT) orchestrates the addition of a glucose moiety to acacetin, culminating in the synthesis of tilianin (Ogata et al., 2004). Within the specialized metabolic route that directs the biosynthesis of rosmarinic acid, the enzymatic action of hydroxycinnamoylCoA:hydroxyphenyllactate hydroxycinnamoyl transferase (RAS) is of significant importance. RAS facilitates condensation between 4-hydroxyphenyllactic acid and 4-coumaroyl-CoA, resulting in the production of 4-coumaroyl-4'-hydroxyphenyllactic acid. This intermediate subsequently undergoes two consecutive hydroxylation processes to produce rosmarinic acid (Petersen, 1991). These transformations are steered by two specific cytochrome P450 enzymes, specifically 3-hydroxycinnamoyl (3-H) and 3′-hydroxycinnamoyl (3′-H) (Petersen, 1997). The Agastache rugosa plant contains several compounds that enhance its aromatic, medicinal, and functional properties. These compounds have been identified across a variety of plant tissues, including the leaves, stems, and flowers. In order to elucidate the functional benefits of Agastache rugosa comprehensively and establish appropriate dosages and applications for various purposes, further scientific research, including organ-specific phenylpropanoid analyses, is warranted.
The primary objective of this study was to examine variations in phenylpropanoid levels across distinct tissue types and developmental stages of Agastache rugosa while also assessing the patterns of gene expression linked to phenylpropanoid biosynthesis.
Materials and Methods
Agastache rugosa seeds (Danong Seed Co., Ltd., Seoul, Korea) were sown on a rockwool medium (60 × 40 cm, 240 holes, UR Rockwool, Suwon, Korea) and germinated at 25 ± 2°C for 28 days. Germinated plants were grown on vermiculite under a fluorescent lamp (TL5 14W/865, Philips, Seoul, Korea) with a light intensity of 210 ± 10 μmol·m-2·s-1. The seedlings were cultivated under meticulously controlled conditions, with a light regimen of 16 hours of illumination followed by an 8-hour dark phase, and a maintained relative humidity of approximately 70% ± 5%. The nutrient solution was carefully adjusted, setting the electrical conductivity to 2.0 dS·m-1 and calibrating the pH to a range between 6.8 and 7.3. The plants grown in the hydroponic system for approximately 39 d were harvested, and their roots, leaves, and flowers were collected. Leaves were categorized into the following stages based on their size: L1 (1 cm), L2 (3 cm), L3 (5 cm), L4 (7 cm), and L5 (9 cm). On the other hand, flowers were categorized into the following four stages based on their degree of flowering: F1 (flower bud), F2 (partial flowering), F3 (full flowering), and F4 (senescence) (Fig. 1). The samples were preserved at ‒70°C for a high-performance liquid chromatography (HPLC) analysis of the phenylpropanoid content and for a quantitative real-time polymerase chain reaction (qRT-PCR) analysis of phenylpropanoid biosynthesis-related genes.
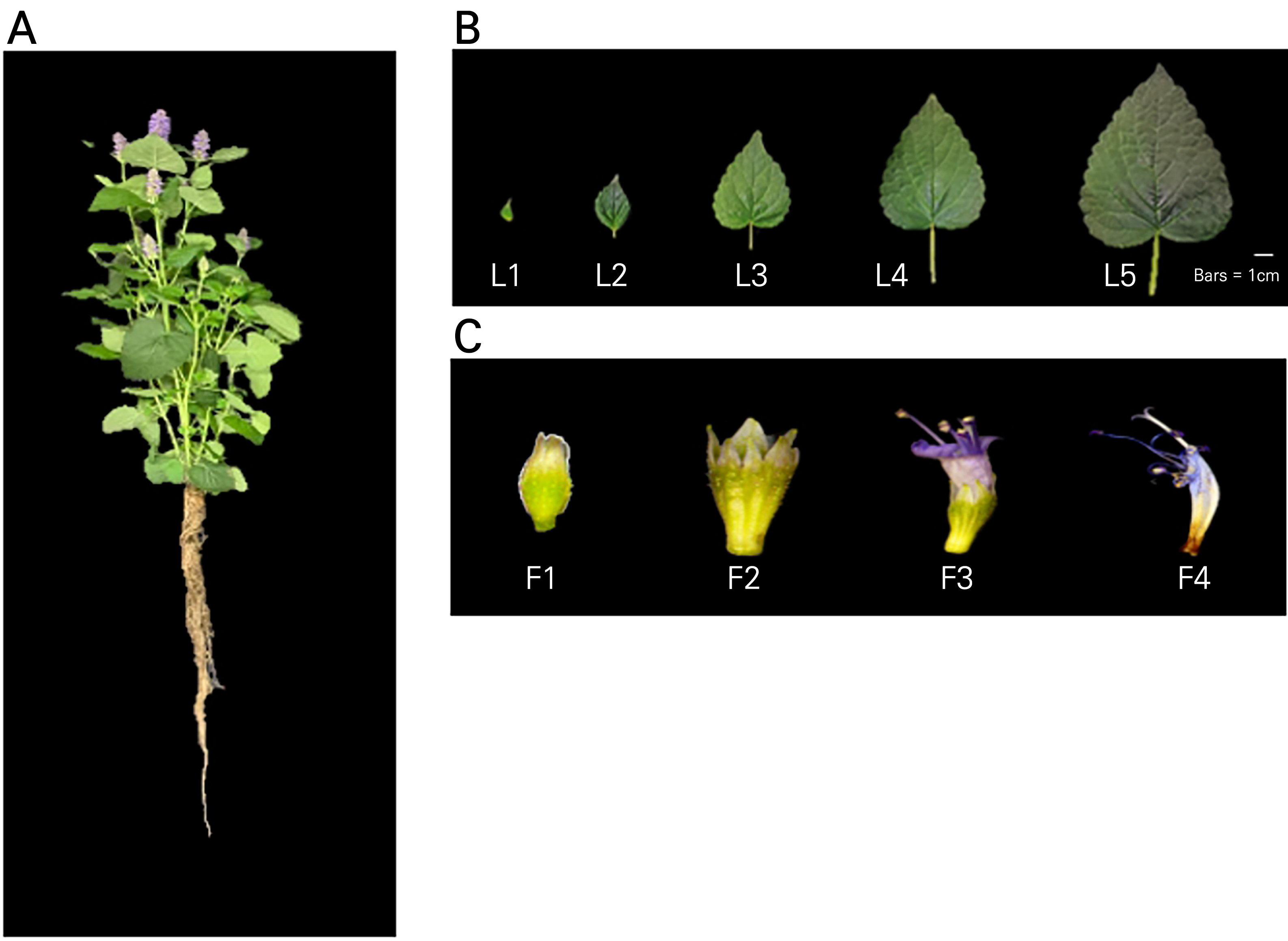
Fig. 1.
Photos of the plants and samples used in this experiment: A, 39-day-old Agastache rugosa plant; B, leaves categorized into five stages based on their size: L1 (1 cm), L2 (3 cm), L3 (5 cm), L4 (7 cm), and L5 (9 cm); and C, flowers categorized into four stages based on their degree of flowering: F1 (flower bud), F2 (partial flowering), F3 (full flowering), and F4 (senescence).
Total RNA extraction and qRT-PCR for gene expression analysis
Total RNA was extracted using Trizol (Invitrogen, MA, USA) from A. rugosa roots, leaves, and flowers at each developmental stage. First-strand cDNA was synthesized from 1 μg of isolated total RNA using oligo (dT) 20 primers (cDNA synthesis kit, Toyobo, STC Co., Ltd.). Primers targeting the conserved region sequences obtained from the NCBI database (Table 1) were designed to analyze the expression patterns of phenylpropanoid biosynthesis-related genes. The PrimeScriptTM RT-PCR Kit (Takara Bio, Shiga, Japan) and gene-specific primers were used for qRT-PCR using 300 ng of cDNA. The gene expression analysis conducted here involved three replicates of qRT-PCR and with calculation of the experimental results with the ΔΔCt method based on the Actin housekeeping gene.
Table 1.
Primers Used in the qRT-PCR Analysis
Phenylpropanoid extraction and HPLC analysis
To analyze the phenylpropanoid content of A. rugosa, samples were dried at ‒70°C for four days using a freeze dryer (TFD5503; Ilshin BioBase, Korea). The dried samples were crushed and powdered. The phenylpropanoid functional material was extracted and a HPLC analysis was conducted using the method described by Park et al. (2020a). The sample powder (100 mg) was mixed with 1.5 mL of methanol (80%, v/v) and sonicated for one hour. The extract was subsequently centrifuged at 15,814 xg for a duration of 10 min. The HPLC analysis was conducted after filtration of the supernatant through a 0.45-μm PTFE syringe filter. HPLC was performed on an Agilent 1260 HPLC system (Agilent Technologies Co. Ltd., Palo Alto, CA, USA) using a C18 column (250 mm × 4.6 mm, 5 μm; RS Tech, Daejeon, Korea). The process employed a 1 mL/min flow rate, a column temperature of 30°C, and a detection wavelength of 340 nm. An automated injector injected 20 μL of every sample and solvent A containing 0.2% (v/v) acetic acid in distilled water and solvent B (100% [v/v] methanol) were used as the mobile phase. The gradient profile was run as follows: 0–15 min: 45% B; 15–20 min: 45%–55% B; 20–45 min: 55%–80% B; 45–50 min: 80% B; 50–52 min: 80%–45% B; and 52–60 min: 45% B (total of 60 minutes). A mixture of 70% solvent A and 30% solvent B was used to separate the materials, and quantification of RA, tilianin, and acacetin standards was performed by means of curve calibration (Lam et al., 2020).
Statistical analysis
The phenylpropanoid content of Agastache rugosa was analyzed in triplicate for each treatment group. For the statistical analysis, SASS version 9.3 (SAS Institute Inc., Cary, NC, USA) was used for an analysis of variance (ANOVA; quantitative analysis), with Tukey’s multiple test method (p < 0.05) used to compare the means between each treatment.
Results
Phenylpropanoid content in different developmental stages of Agastache rugosa
The concentrations of rosmarinic acid, tilianin, and acacetin were analyzed separately in various organs of 39-day-old Agastache rugosa plants, in this case the roots, leaves, and flowers, at different developmental stages. High-performance liquid chromatography (HPLC) was employed to extract and quantify the rosmarinic acid, tilianin, and acacetin. The rosmarinic acid content in Agastache rugosa ranged from 5.66 to 15.52 mg·g-1 at different stages of leaf development, displaying a continuous 2.74-fold increase from L1 to L5, corresponding to the increasing leaf size (Fig. 2A). The tilianin content in Agastache rugosa ranged from 0.84 to 4.92 mg·g-1, with the highest content observed at L1, followed by a 5.85-fold decrease until L4 but then a 1.55-fold increase at L5 compared to L4. In contrast, the acacetin and tilianin contents exhibited different patterns compared to rosmarinic acid. The acacetin content ranged from 0.12 to 1.72 mg·g-1, with the highest content observed at L1 and with a subsequent reduction of 14.09-fold by L4 (Fig. 2A).
When the phenylpropanoid content was assessed based on the flowering stage, the rosmarinic acid content showed a 2.6-fold increase from F1 to F4, as illustrated in Fig. 2B. The lowest tilianin content was recorded at the F1 stage (1.23 mg·g-1), while the acacetin content was lowest at the F4 stage (0.07 mg·g-1; Fig. 2B). Notably, the rosmarinic acid concentration increased with the development of leaves and flowers in Agastache rugosa, whereas the concentrations of tilianin and acacetin declined during leaf and flower development. It should be noted that only rosmarinic acid was detectable in the roots of Agastache rugosa (12.12 mg·g-1; Fig. 2C).
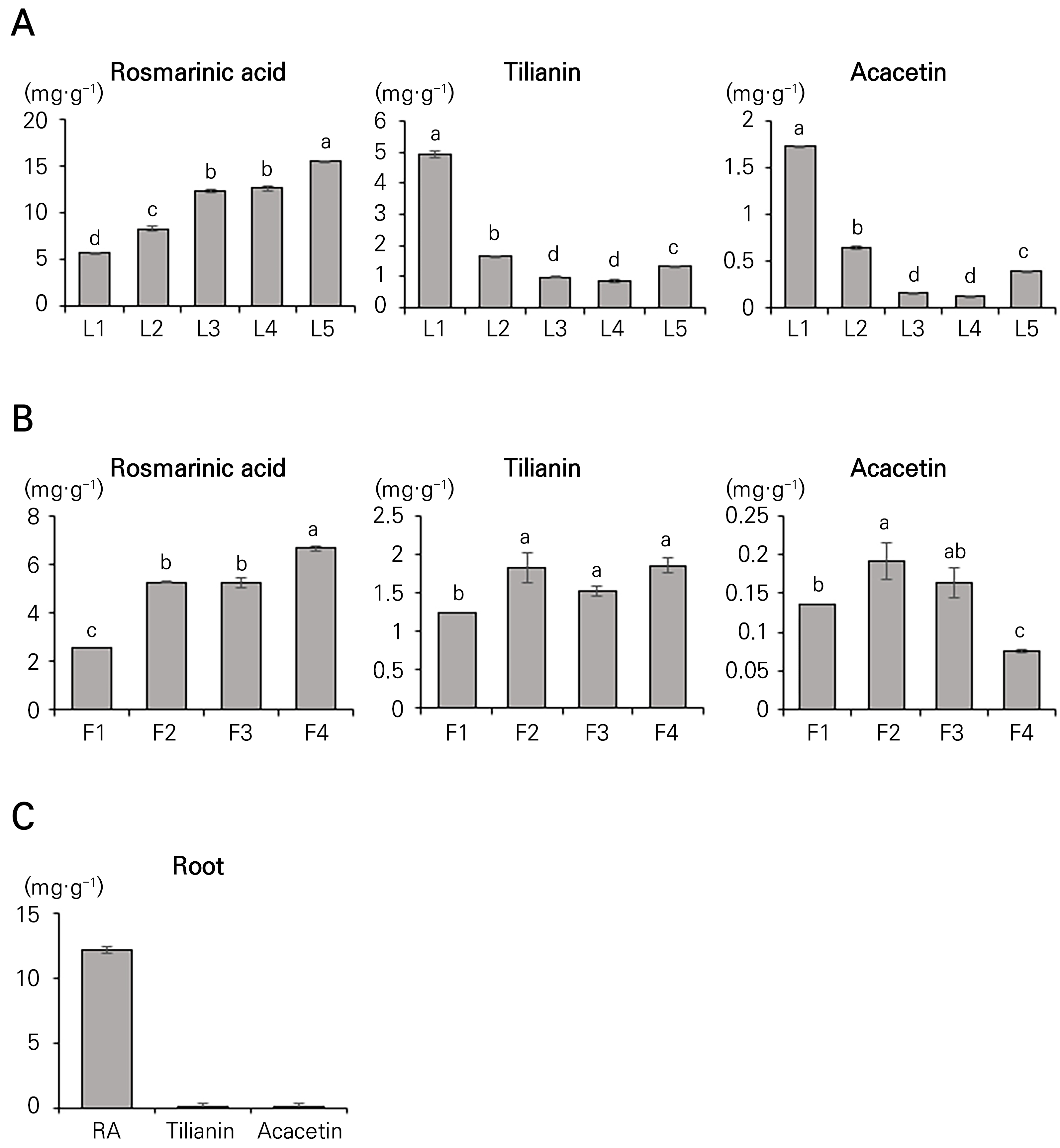
Fig. 2.
Quantification of the levels of rosmarinic acid, tilianin, and acacetin in the (A) leaves: L1 (1 cm), L2 (3 cm), L3 (5 cm), L4 (7 cm), and L5 (9 cm), (B) flowers: F1 (flower bud), F2 (partial flowering), F3 (full flowering), and F4 (senescence), and (C) roots: growth stage after 39 days of Agastache rugosa. Data are presented as the mean ± SD of three independent experimental sessions (p < 0.05).
Expression levels of phenylpropanoid-related genes at different developmental stages of Agastache rugosa
To understand the gene expression profiles at different developmental stages of Agastache rugosa, in this study we assessed the transcriptional profiles of genes implicated in the phenylpropanoid biosynthetic pathway. To this end, we analyzed the expression levels of seven phenylpropanoid biosynthesis-related genes (ArPAL, ArC4H, Ar4CL, ArCHS, ArCHI, ArTAT, and ArHPPR) associated with the phenylpropanoid content at different developmental stages of the leaves and flowers of Agastache rugosa by means of qRT-PCR. Ar4CL expression was 1.82-fold higher in L2 than in L1 but gradually decreased by 2.84-, 5.11-, and 8.96-fold from L3. ArTAT expression decreased by 2.53-fold from L1 to L3 and then increased at L4 and L5. The expression levels of ArC4H and ArPAL decreased by 7.55- and 1.09-fold, respectively, from L1 to L4 and then increased by 44.1% and 9.28%, respectively, at L5. However, the expression levels of ArCHI and ArCHS decreased by 1.95- and 1.98-fold, respectively, at L5 as the flowering stage progressed (Fig. 4A). The examination of phenylpropanoid biosynthesis-related genes in Agastache rugosa flowers at different developmental stages revealed that the expression levels of ArPAL, ArCHS, and ArCHI decreased by 1.98-, 1.99-, and 1.88-fold, respectively, between F1 and F3, followed by corresponding increases of 200.31-, 98.06-, and 1.89-fold, at F4. The expression levels of ArC4H and Ar4CL increased by 1.05- and 1.31-fold, respectively, from F1 to F2 and then decreased by 1.66- and 1.71-fold, respectively, at F3. ArHPPR expression decreased by 1.61-fold from F1 to F3 and then increased by 2.42-fold at F4 (Fig. 4B). Ar4CL, ArC4H, and ArPAL were the only genes that were significantly expressed in the roots in comparison with the other organs (Fig. 4C). With an increase in the rosmarinic acid concentration, the levels of tilianin and acacetin decreased, indicating the involvement of genes involved in phenylpropanoid biosynthesis.
Phenolic compounds are found extensively throughout various plant species and showcase a range of biological characteristics linked to plants' natural mechanisms for protection against both living and non-living environmental challenges (Douglas, 1996; Higdon et al., 2007; Park et al., 2017). In addition, phenolics show beneficial effects on human health, including radical-scavenging, antioxidant, antiviral, antispasmodic, anti-inflammatory, and antimicrobial properties (Bily et al., 2004; Diaz et al., 2004).
Earlier studies have indicated that the primary active constituents in Agastache rugosa are rosmarinic acid, tilianin, and acacetin, which shows remarkable pharmacological properties, specifically anti-allergic, antioxidant, antimicrobial, antitumor, antidepressant, anticancer, antiviral, and anti-asthmatic activities (Tuan et al., 2012; Wei et al., 2016). The quality of these herbal plants allows an evaluation of their secondary metabolite concentrations. Every component of Agastache rugosa has applications in traditional medicine across diverse civilizations to address a spectrum of ailments (Zielinska et al., 2014). Our findings showed that the rosmarinic acid content in the roots, leaves, and flowers exceeded the levels of tilianin and acacetin. We also observed decreases in the tilianin and acacetin contents with an increase in the rosmarinic acid content as the leaves developed (Fig. 2). In a study by Tuan et al. (2012), the flowers contained the highest levels of rosmarinic acid, tilianin, and acacetin, while acacetin was not detected in the stems or roots, similar to our study. The rosmarinic acid, tilianin, and acacetin contents in fennel have also been shown to differ depending on the temperature of the root zone (Tuan et al., 2012).
There is a correlation between the expression of genes involved in phenylpropanoid biosynthesis and the flavonoid content in plants (Xu et al., 2008; Rani et al., 2009; Singh et al., 2009). The activation of pivotal enzymes triggers phenolic biosynthesis, resulting in the upregulation of key genes, specifically PAL and CHS, within the phenylpropanoid pathway (Tuan et al., 2012; Yeo et al., 2021). In particular, changes in the content of rosmarinic acid have been reported to have a direct correlation with the expression of PAL (Yong-Sheng et al., 2009). Downregulation of PAL affects the expression of C4H and 4CL, leading to a decrease in the content of rosmarinic acid (Song and Wang, 2011). The synthesis of rosmarinic acid, acacetin, and tilianin during phenylpropanoid synthesis is controlled by the 4-coumaroyl-CoA pathway (Fig. 3). Yeo et al. (2021) found that the expression of CHS increased from stage I (bud) to stage III (full flowering) and that the tilianin content was highest among all flower development stages. However, although the rosmarinic acid level was the highest at stage III (full flowering), the levels of tilianin and acacetin were higher at stage II. These results are consistent with the findings of Yeo et al. (2021). Within medicinal plants, enzymes such as PAL, C4H, and 4CL play a crucial role in phenylpropanoid biosynthesis, contributing significantly to the production of rosmarinic acid (Petersen, 1997). In the rosmarinic acid biosynthesis pathway of Salvia miltiorrhiza, PAL serves as a pivotal enzyme, and alterations in rosmarinic acid levels are directly associated with the expression of PAL (Hu et al., 2009). Furthermore, the suppression of PAL leads to a decrease in rosmarinic acid levels in Salvia miltiorrhiza, influencing the expression levels of C4H and 4CL (Song and Wang, 2011). PAL and 4CL expression levels have also been shown to be correlated with the enzymatic activity and rosmarinic acid content in Melissa officinalis (Weitzel and Petersen, 2010). In Agastache rugosa leaves, the genes responsible for phenylpropanoid biosynthesis, specifically PAL, 4CL, CHS and CHI, exhibited elevated expression levels that were directly correlated with an increase in the rosmarinic acid content. The expression patterns of CHS and CHI were notably congruent with the accumulation patterns of acacetin and tilianin across various organs of A. Rugosa. CHS and CHI catalyze the first and second steps in the plant phenylpropanoid pathway, respectively, leading to the production of acacetin, tilianin, and other flavonoids. Previous studies have revealed that CHS and CHI act as rate-limiting enzymes in the flavonoid biosynthesis pathway in certain plants (Knogge et al., 1986; Muir et al., 2001; Li et al., 2006). This suggests that CHS and CHI may regulate the synthesis of not only acacetin and tilianin but also other flavonoids in A. Rugosa. The roots also showed significant accumulation of rosmarinic acid in Agastache rugosa, although the expression levels of PAL, C4H, and 4CL were low (Fig. 4). Tuan et al. (2012) showed that CHS and CHI are mainly present in the flowers and contribute to the production and transport of tilianin from the roots and stems to the leaves and flowers. In our experiment, the highest tilianin content was observed at F1 among the developmental stages of CHS and CHI (Fig. 4). The second-highest level was detected at F2, suggesting that CHS and CHI play roles in the regulation of tilianin.
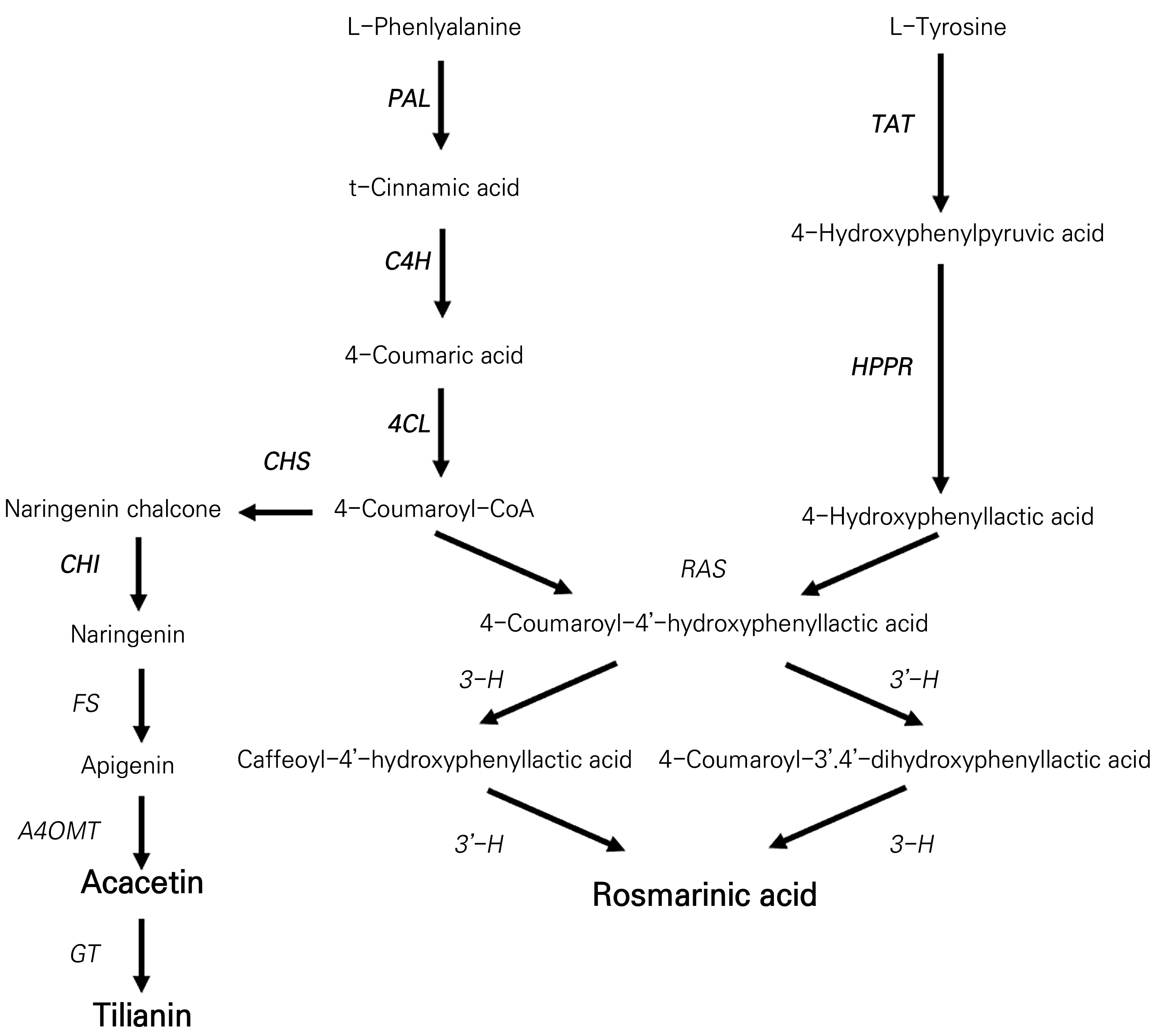
Fig. 3.
Proposed biosynthetic pathways of acacetin, tilianin, and rosmarinic acid. The genes in bold are those for which the expression level was analyzed in this experiment. PAL, phenylalanine ammonia-lyase; C4H, cinnamate 4-hydroxylase; 4CL, 4-coumaroyl-CoA ligase; TAT, tyrosine amino transferase; HPPR, hydroxyl phenylpyruvate reductase; CHS, chalcone synthase; and CHI, chalcone isomerase; FS, flavone synthase; A4OMT, apigenin 4’-O-methyltransferase; GT, glucosyltransferase; RAS, hydroxycinnamoyl-CoA:hydroxyphenyllactate hydroxycinnamoyl transferase; 3-H, hydroxycinnamoyl; 3’-H, hydroxycinnamoyl.
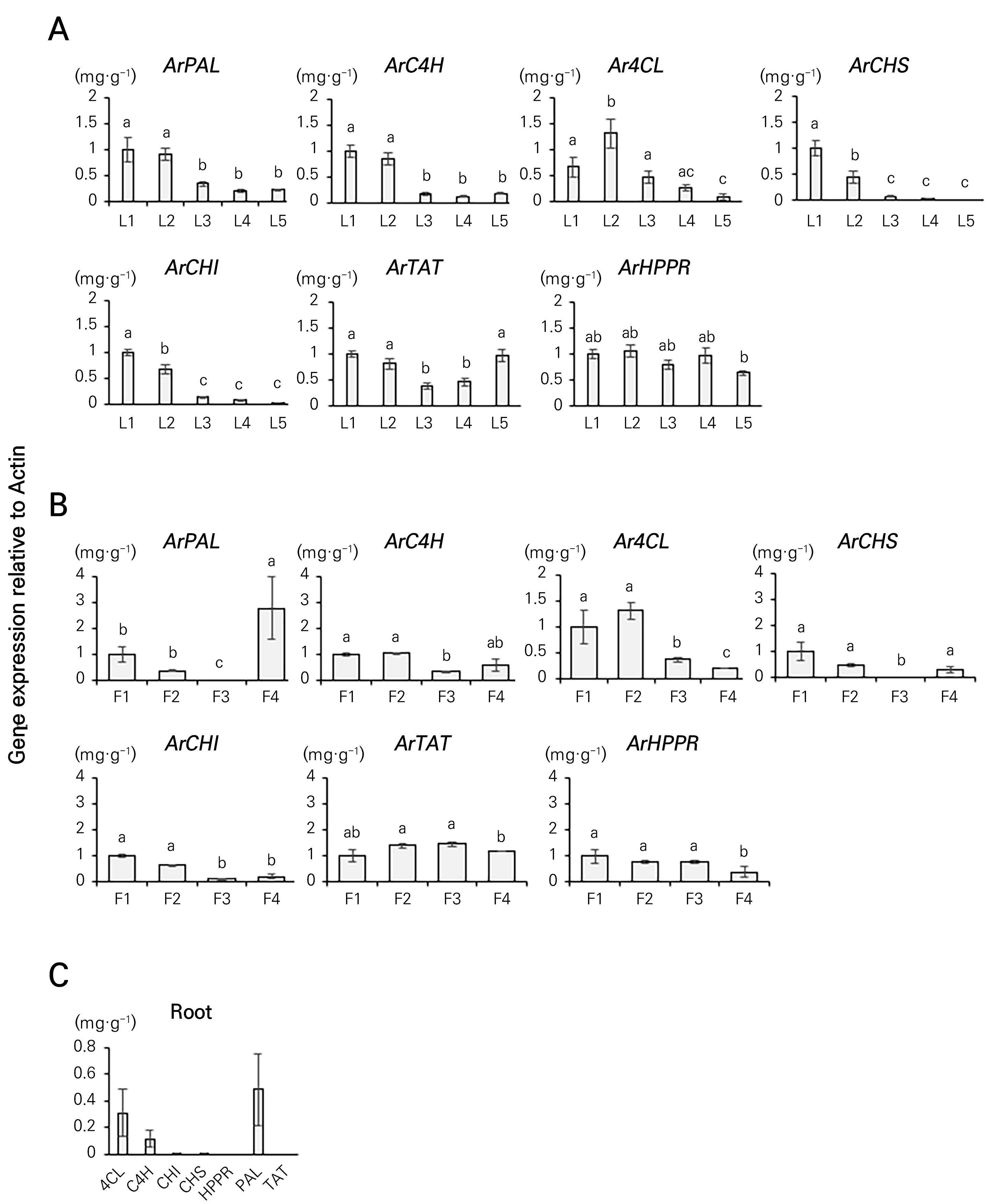
Fig. 4.
The expression levels of ArPAL, ArC4H, Ar4CL, ArCHS, ArCHI, ArTAT and ArHPPR were analyzed in various organs of Agastache rugosa. (A) Leaves: L1 (1 cm), L2 (3 cm), L3 (5 cm), L4 (7 cm), and L5 (9 cm); (B) flowers: F1 (flower bud), F2 (partial flowering), F3 (full flowering), and F4 (senescence); and (C) roots: growth stage after 39 days of Agastache rugosa. Data are presented as the mean ± SD of three independent experimental sessions (p < 0.05).
These data are significant for future research aimed at enhancing the production of bioactive compounds from A. rugosa. In other studies of Agastache rugosa, various concentrations of yeast extract and nitrate were found to induce the accumulation of rosmarinic acid in A. rugosa and enhance the expression levels of genes related to phenylpropanoids (Tuan et al., 2012). Among differently colored LEDs, white light was reported to be most effective in inducing the synthesis of phenylpropanoids in A. rugosa (Park et al., 2020b). Based on these findings, further research is deemed necessary to explore the enhancement of genes related to phenylpropanoids using substance treatments and light treatments. Based on the findings here, it is evident that the leaves and flowers of Agastache rugosa exhibit promising attributes, suggesting their potential applicability as biomaterials across a spectrum of industries, encompassing pharmaceuticals, cosmetics, food, and other relevant sectors.
Conclusions
This study analyzed the phenylpropanoid content in relation to different stages of development of the roots, leaves, and flowers of 39-day-old Agastache rugosa plants. In the leaves, the rosmarinic acid content increased steadily while the tilianin and acacetin contents decreased gradually across the five developmental stages. In the flowers, the rosmarinic acid levels gradually increased, but the tilianin and acacetin levels were highest at the F2 stage. Only rosmarinic acid was detected in the roots. An analysis of genes associated with phenylpropanoid biosynthesis revealed that as the rosmarinic acid content increased, the expression levels of ArPAL, ArC4H, ArCHS, and ArCHI decreased. This investigation establishes a foundational framework for subsequent research endeavors, specifically targeting augmentation of the phenylpropanoid content by elucidating the roles of genes implicated in its biosynthetic pathway.