Introduction
Materials and Methods
Plant Material and Growth Conditions
Treatment Methods and Experimental Groups
Data Measurement
Statistical Analysis
Results
Fruit Development after Fruit Setting
Fruit Characteristics
Seed Characteristics
Discussion
Parthenocarpy Traits of Both Cultivars and Effects of the 2,4-D Application
Fruit Development and Morphology
Fruit Characteristics and Quality
Characteristics of Seeds
Introduction
Cucurbita species are steadily consumed in many countries, including Asia, and provide a variety of minerals and large amounts of amino acids and vitamins. In particular, active compounds such as carotenoids, flavonoids, polyphenols, and tocopherols, which are abundant in pumpkins, can have antioxidant and anticancer properties, lowering blood pressure and the risk of diabetes (Kaur et al., 2019). In Korea, pumpkins, squash, and immature squash (Cucurbita moschata), kabocha squash (Cucurbita maxima), and zucchini (Cucurbita pepo) are widely consumed (Oh et al., 2014).
Species in the Cucurbita family are monoecious crops that rely on insects for pollination and fertilization. When immature fruits are cultivated in winter, a hormone treatment or artificial pollination is required because there is no pollen medium, such as pollination insects, which increases the costs associated with fruit production (Robinson and Reiners, 1999; Pomares-Viciana et al., 2017; Goo et al., 2022). In particular, crops that depend on pollinating insects, such as Cucurbitaceae, are more sensitive to insect population declines caused by pesticides and climate change (Potts et al., 2016). Fruit development is triggered by pollination and fruit setting, which are important phases of crop production. Low or high temperatures, insufficient humidity levels, and ineffective pollination result in decreased fruit set, which lowers the fruit yield (Pomares-Viciana et al., 2017).
Rao et al. (2018) and Knapp et al. (2017) suggested breeding parthenocarpic vegetables as a countermeasure against the impacts of climate change, as they have the potential to reduce the demand for pollination and pollinators. Parthenocarpic traits have gradually become necessary in response to climate change.
Parthenocarpy is a phenomenon in which fruit sets are formed in angiosperms without fertilization. It generally occurs naturally but can be artificially induced using phytohormones (Gustafson, 1942). Cultivars with parthenocarpic traits do not require pollination. Therefore, when cultivated, fruits can be stably set under conditions unfavorable for fertilization (Spena and Rotino, 2001). This can increase production, decrease the cost of using mediating insects, and reduce the labor required for artificial fertilization, resulting in decreased production costs (Knapp et al., 2017). Parthenocarpy can be used to produce seedless fruits and improve the quality of fruit (Marr and Gast, 1991; Pandolfini, 2009; Dhatt and Kaur, 2016). Genetic research to identify parthenocarpy-related loci in cucumbers has revealed that the parthenocarpic genetic inheritance is quantitative (Sun et al., 2006).
Representative cultivars with parthenocarpic traits include banana, tangerine, pineapple, pear, and cucumber. Cucumber (Cucumis sativus) and zucchini (C. pepo) are representative of Cucurbitaceae, and in recent years, genetic resources with parthenocarpy have been found in C. moschata and Cucumis melo L. (Kim et al., 1992; Martínez et al., 2014; Yoshioka et al., 2017; Takisawa et al., 2021). However, because Cucurbita species have very weak parthenocarpic potential, they are difficult to find, apart from C. pepo (Om and Hong, 1989; Takisawa et al., 2021; Goo et al., 2022).
Parthenocarpy can be artificially induced through a variety of processes, such as an external application of hormones and gene manipulation, even in crops lacking parthenocarpic traits (Gustafson, 1942; Subbaraya et al., 2020; Fayaz et al., 2021). Currently, these methods are used to produce marketable fruits from various crops, such as apples, pears, grapes, melons, and tomatoes (de Jong et al., 2009; Dhatt and Kaur, 2016; Kim et al., 2019; Takisawa et al., 2019; Sharif et al., 2022). Additionally, parthenocarpy can be induced using pollen extracts (Peres et al., 2019)
Owing to the various advantages of parthenocarpy, research on parthenocarpy is focusing on various crops worldwide; however, research on the parthenocarpy of C. moschata, including pumpkin and Korean squash, is insufficient compared to that on other crops, especially in Korea. Takisawa et al. (2019) reported a Japanese parthenocarpic C. moschata cultivar and compared its characteristics with those of pollinated fruit. Here, we investigate two parthenocarpic squash cultivars selected from butternut squash (C. moschata) in Korea and compare the development and characteristics of fruit artificially treated with 2,4D hormones and parthenocarpic fruit in this cultivar.
Materials and Methods
Plant Material and Growth Conditions
The cultivars used in this experiment are two pure lines showing parthenocarpic traits among the genetic resources of 20 lines of butternut squash held in a laboratory. For the butternut squash genetic resources, 20 lines were selected using a pedigree breeding method for eight generations up to 2001 from butternut squash genetic resources collected in China in 1993 (Fig. 1).
The seeds were germinated on Petri dishes covered with filter paper on April 20, 2022, in a dark condition with the thermostat set at 25 °C. Seeds with more than 2 mm of radicle roots were sown in 50-hole plug trays (5 × 10) filled with horticultural bed soil. After sowing, approximately 25 days of a seedling period elapsed by May 15 in a plastic greenhouse at a farm run by Kangwon National University (Chuncheon 24204, Korea). Thirty plants of each cultivar were planted in a 120-cm row length, spaced 30 cm apart, and cultivated according to the standard cultivation methods of the Rural Development Administration of Korea. Damage caused by aphids has frequently been reported during the squash cultivation process (Park, 2021). To prevent greenhouse whiteflies and aphids, a solution of a sulfoxaflor suspension concentrate (Straight, Dongbang Agro Corporation, Seoul, Korea) was sprayed during the growing of the seedlings and a solution of imidacloprid (2%) granules (Conido, Bayer Korea, Seoul, Korea) was added to the soil during planting. To control powdery mildew, a solution of a metrafenone (25.2 %) suspension concentrate (Bibando, FarmHannong Co., Korea) was applied to the foliage during cultivation.
Treatment Methods and Experimental Groups
Because the fruit characteristics of the set on the lower nodes vary depending on the physiological conditions, female flowers of the tenth to twelfth nodes were used in the experiment. Three treatments were applied to the two flowers (17R-1 and 19-7), with the names Pollinated Fruit (PF), Hormone-treated Fruit (HF), and Unpollinated Fruit (UF) used. PF was set by artificially pollinating female flowers at anthesis using pollen from male flowers at 6:00 AM. In the UF treatment, the flowers were sutured with a binder clip before anthesis to observe natural parthenocarpy. In HF, a 50 ppm solution of 2,4-dichlorophenoxyacetic acid (2,4-D), a synthetic growth hormone of the auxin family, was sprayed onto the stigma of female flowers on the day of anthesis to induce parthenocarpy artificially (Queiroga et al., 2017; Hu et al., 2019).
Data Measurement
Fruit development was investigated from the day of anthesis to 30 days, and fruit length and diameter were measured every five days. The measurement method involved taking a picture of the fruit aligned with a square ruler and nondestructively obtaining the value by processing the pixels of the digital image using ImageJ (Ver. Java 1.8.0, NIH, USA). Fruit length was measured at the longest part of the fruit and the fruit diameter was measured at the widest part of the line perpendicular to the fruit length line. Unlike the caliper method, the method of measuring the scale of agricultural products using images can be automated and is economical (Igathinathane et al., 2009; Das et al., 2019).
Fruit characteristics were investigated by destroying fruits harvested when the stem was corked for approximately 50 days after anthesis, after allowing them to ripen for one month. The fruit weight, fruit length, fruit diameter, fruit cross-sectional area, placental part area, placental part weight, total soluble solids (TSSs), and firmness were measured. Fruit and placental weights were measured using an electronic scale, and the fruit length, fruit diameter, fruit cross-sectional area, and placental part area were measured using an image analysis program developed by the Korea Seed & Variety Service and Korea Electronics Technology Institute (Park et al., 2021). TSS was measured using a digital refractometer (Pocket Refractometer PAL-1, Atago, Japan) for juice squeezed from gauze after the fruit was uniformly ground on a steel plate. Firmness was measured in the middle of the flesh using a rheometer (Sun Rheo Meter Compac-100II, Sun Scientific Co., Ltd., Tokyo, Japan) and a stainless steel probe with a diameter of ø3 mm; here this is expressed in N (Newton).
The presence or absence of embryos and the formation of seed coats were investigated, and the length, width, and thickness of the seeds were examined using digital Vernier calipers.
Statistical Analysis
All measured values of the traits and characteristics were analyzed for each cultivar using SPSS (version 26.0; IBM, Armonk, NY, USA). Statistical significance was determined by means of a one-way analysis of variance, and mean separation was tested post-hoc using Duncan’s multiple range test.
Results
Fruit Development after Fruit Setting
The fruits were set and developed normally in all groups, and there were differences in the fruit scale and morphology between the cultivar and treatment method upon anthesis.
In the 17R-1s cultivar, 30 days post-anthesis (DPA) the fruit lengths of PF, HF, and UF were 23.48, 24.00, and 22.38 mm, respectively. There was no significant differences in the fruit length, with the corresponding fruit diameters being 9.43, 9.38, and 8.79 mm. There were also no significant differences among the groups in terms of the fruit length and fruit diameter at 30 DPA. However, at 5 DPA and 10 DPA, the fruit diameter of UF was significantly shorter than those of PF and HF. The fruit diameters of PF, HF, and UF were 5.27, 6.32, and 6.26 mm at 5 DPA and 8.87, 9.12, and 7.56 mm at 10 DPA (Fig. 2). Additionally, the fruit diameters of PF, HF, and UF were respectively 9.13, 9.12, and 8.43 mm at 15 DPA; 9.29, 9.01, and 8.68 mm at 20 DPA; 9.27, 9.09, and 8.66 mm at 25 DPA; and 9.43, 9.38, and 8.79 mm at 30 DPA. Although there were no statistically significant differences, the fruit diameter in the UF case tended to be shorter than that in the other treatments.
In the 19-7s cultivar, the fruit lengths measured at 30 DPA were 12.25, 11.86, and 10.17 mm in PF, HF, and UF cases, respectively. The fruit length in the UF group was significantly shorter than those of the other groups at 30 DPA, and this difference remained significant from 5 DPA to harvest. The fruit lengths of PF, HF, and UF were 9.84, 9.33, and 7.54 mm at 5 DPA; 11.89, 11.32, and 9.97 mm at 10 DPA; 12.24, 11.72, and 10.06 mm at 20 DPA; and 9.43, 9.38, and 8.79 mm at 30 DPA. The fruit diameters were 11.66, 11.13, and 12.07 mm, respectively, and there were no significant differences among the groups or during development (Fig. 2). The fruit diameters of PF, HF, and UF were 8.06, 7.58, and 7.25 mm at 5 DPA; 10.77, 10.31, and 11.13 mm at 10 DPA; 11.58, 10.93, and 12.03 mm at 20 DPA; and 11.66, 11.13, and 12.07 mm at 30 DPA. Although there were no statistically significant differences, the fruit diameter of UF tended to be thicker than those of the other treatments.
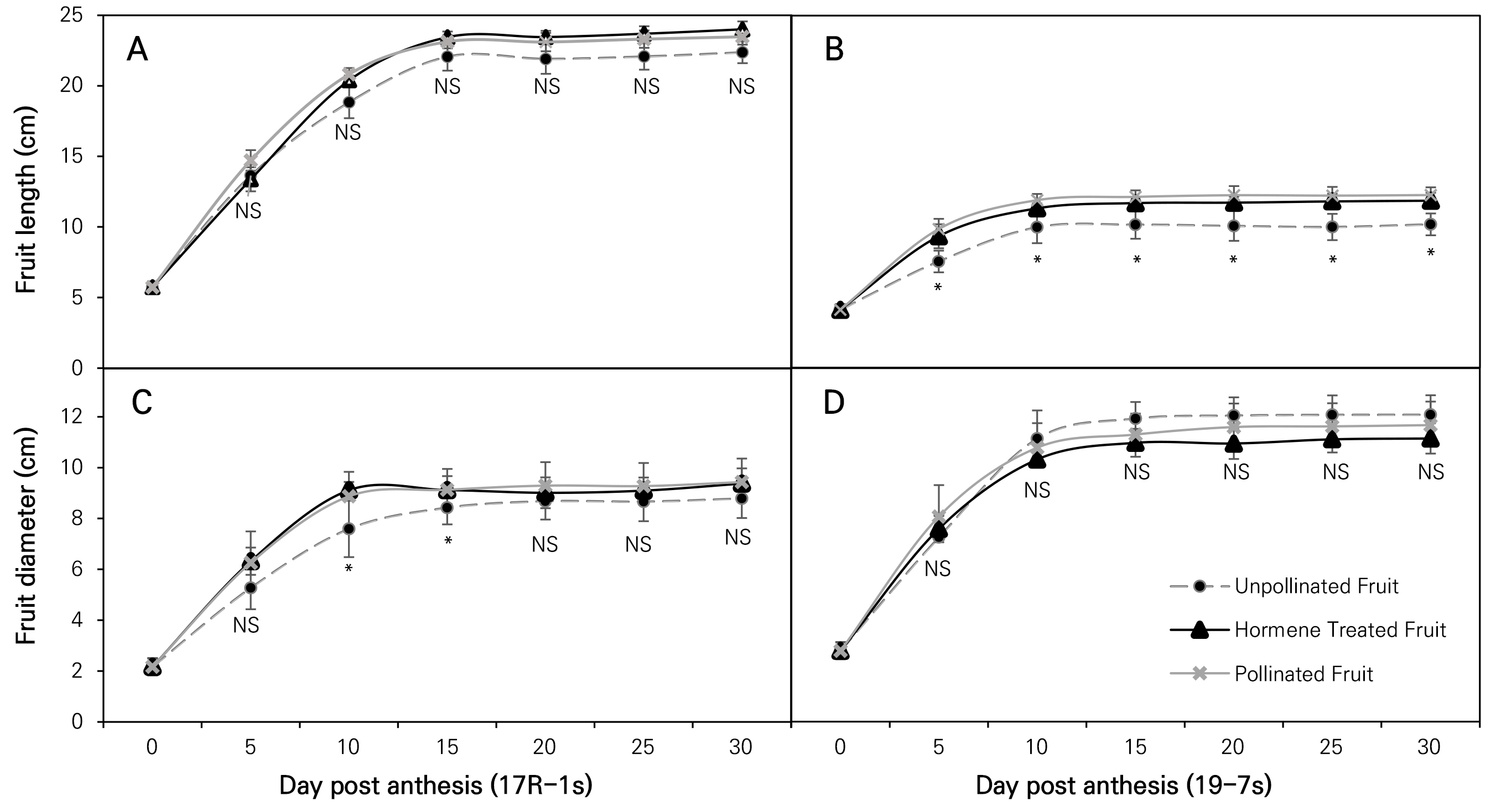
Fig. 2.
Changes in fruit length and fruit diameter by date (up to 30 days) according to the treatment and cultivar in two parthenocarpic butternut squash cultivars, where vertical bars represent ± SD (n = 4‒8) and * indicates a significant difference (p < 0.05) from the mean of each treatment at the same DPA.
Fruit Characteristics
In the 17R-1s cultivar, there were no significant differences in the fruit characteristics among the groups. Fruit weights were 1.06, 1.1, and 1.02 kg for the PF, HF, and UF cases, respectively. The TSS contents of the fruit flesh were 15.93, 16.65, and 16.32 Brix. No differences were observed in other fruit characteristics between the treatment groups (Table 1).
In the 19-7s cultivar, the fruit lengths were 12.22, 11.6, and 10.28 mm for PF, HF, and UF, respectively, and that of UF was significantly shorter than those of the other treatments. There were no significant differences in the fruit diameters among the groups (Table 1). The fruit weights were 943.75, 847.5, and 925 kg and the TSS contents of PF, HF, and UF were 11.22, 11.72, and 12.19 Brix, respectively, with no significant differences among the groups. Additionally, there were no significant differences in the fruit cross-sectional and placental areas among the groups (Table 1). However, the weight of the placenta in UF (16.2 g) was significantly lower than those in the PF and HF groups (32.4 and 24.2 g, respectively) (Table 1).
Table 1.
Fruit Characteristic after Ripening for Four Weeks in Two Butternut Squash (Cucurbita moschata) Cultivars
Cultivar | Treatmentz |
Fruit weight (g) |
Fruit length (cm) |
Fruit diameter (cm) |
Placenta part weight (g) |
FCSAy (cm2) |
Placenta part area (cm2) |
TSSx (°Brix) |
Firmnessw (N) |
17R-1s | PF | 1058.3 ± 151.1 av | 23.6 ± 0.5 a | 9.4 ± 0.5 a | 33.5 ± 5.7 a | 171.8 ± 16.1 a | 31.4 ± 2.4 a | 15.9 ± 1.0 a | 22.7 ± 1.2 a |
HF | 1095.0 ± 80.7 a | 23.1 ± 0.8 a | 9.2 ± 0.3 a | 35.2 ± 5.1 a | 173.6 ± 7.9 a | 31.2 ± 3.3 a | 16.6 ± 1.0 a | 22.3 ± 2.3 a | |
UF | 1022.5 ± 115.5 a | 22.3 ± 0.9 a | 9.0 ± 0.5 a | 19.2 ± 5.1 b | 165.6 ± 14.5 a | 24.5 ± 6.0 a | 16.3 ± 1.2 a | 22.0 ± 1.8 a | |
Significance | NS | NS | NS | * | NS | NS | NS | NS | |
19-7s | PF | 943.7 ± 176.0 a | 12.2 ± 0.6 a | 11.7 ± 1.0 a | 32.4 ± 12.3 a | 117.1 ± 11.7 a | 21.3 ± 5.1 a | 11.2 ± 0.6 a | 15.6 ± 1.3 a |
HF | 847.5 ± 36.6 a | 11.6 ± 0.5 ab | 11.3 ± 0.4 a | 24.2 ± 4.7 ab | 110.6 ± 1.3 a | 20.0 ± 1.8 a | 11.7 ± 0.4 a | 16.3 ± 0.7 a | |
UF | 925.0 ± 175.9 a | 10.2 ± 1.2 b | 12.3 ± 0.8 a | 16.2 ± 8.6 b | 103.6 ± 17.5 a | 17.6 ± 3.7 a | 12.1 ± 0.9 a | 15.6 ± 1.1 a | |
Significance | NS | * | NS | * | NS | NS | NS | NS |
Seed Characteristics
In both cultivars, UF had significantly lower seed weights relative to the fruit, length, width, and thickness compared to these characteristics of the other experimental groups. In particular, the thicknesses of the seeds were 3.36, 10.3, and 0.14 mm for PF, HF, and UF, respectively, in the 17R-1s cultivar, while they were 3.19, 0.93, and 0.48 mm for PF, HF, and UF, respectively, in the 19-7s cultivar (Table 2). The differences between the cultivars were mainly due to the presence or absence of embryos and the development of seeds. The inside of the PF seeds showed normal development of the seed elements, including the embryos, whereas in the UF seeds, seed elements including embryos were insufficient or degenerated. In HF, the artificially induced parthenocarpy led to seeds that developed without embryos (Fig. 3).
Table 2.
Seed Characteristics of Pollinated Fruit (PF), Hormone-treated Fruit (HF), and Unpollinated Fruit (UF) in Two Butternut Squash Cultivars
Cultivar | Treatment | SWpFz(g) | Seed length (mm) | Seed width (mm) | Seed thickness (mm) |
17R-1s | PF | 22.58 ± 2.41 ay | 14.86 ± 1.06 a | 9.29 ± 0.34 a | 3.36 ± 0.22 a |
HF | 18.64 ± 2.35 a | 13.22 ± 0.99 ab | 7.07 ± 0.69 b | 1.03 ± 0.26 b | |
UF | 4.32 ± 3.66 b | 10.81 ± 3.36 b | 6.42 ± 2.57 b | 0.14 ± 0.09 c | |
Sigx | * | *** | *** | *** | |
19-7s | PF | 10.92 ± 3.26 a | 11.98 ± 0.9 a | 7.46 ± 1.15 a | 3.19 ± 0.52 a |
HF | 4.81 ± 1.77 b | 10.8 ± 0.88 a | 6.84 ± 0.75 a | 0.93 ± 0.20 b | |
UF | 0.96 ± 2.86 b | 7.61 ± 1.58 b | 3.55 ± 0.48 b | 0.48 ± 0.25 c | |
Sig | * | *** | *** | *** |
Discussion
Compared to those of zucchini (C. pepo), reports on genetic resources with parthenocarpic traits in C. moschata species are very rare; therefore, research on genetic resources with parthenocarpic traits in C. moschata for the breeding of parthenocarpic cultivars is required (Takisawa et al., 2021). This study of parthenocarpic squash genetic resources was conducted in Korea through introduced breeding and line breeding. Furthermore, we investigated the characteristics of the fruit of this variety when it develops as a parthenocarpic fruit, with the characteristics of the seeds investigated and compared to those in other works.
Parthenocarpy Traits of Both Cultivars and Effects of the 2,4-D Application
In the two cultivars 17R-1s and 19-7s, PF, UF, and HF were all set and developed normally. In particular, the normal UF setting suggests that both cultivars have parthenocarpic characteristics, and the absence of embryos in the UF seeds excludes the possibility of insect pollination during the experiment.
The normal development of HF supports that 2,4-D, a synthetic auxin hormone, induces fruit set and parthenocarpy in cucurbits. Many studies have attempted to set fruit using growth regulators in the Cucurbitaceae family. For example, Ogawa and Takisawa (2022) treated female flowers at anthesis with 1-naphthaleneacetic acid (NAA), N-(2-chloro-4-pyridyl)-N'-phenylurea (CPPU), gibberellic acid 3 (GA3), and brassinolide, suggesting that NAA and CPPU can effectively induce parthenocarpy artificially in C. moschata. Moreover, 2,4-D effectively induces parthenocarpy in cucumber (Hu et al., 2019). GA3 effectively induces parthenocarpy in fruit crops such as grapes, apples, and pears but has no effect on cucurbit crops, and GA4+7 effectively induces parthenocarpy in cucumbers (Qian et al., 2018).
In general, it is normal for fruits to set after pollination and fertilization. Fruit development involves the mutual regulation of endogenous hormones, such as auxins, gibberellins, and cytokinins (Kim et al., 1992; Knapp et al., 2017; Ozga and Reinecke, 2003; Sharif et al., 2022). After fertilization, seeds play an important role in the generation and regulation of endogenous hormones (Gillaspy et al., 1993; Dhatt and Kaur, 2016; Ozga et al., 2002). Therefore, pollination methods such as insect mediation, blowing wind, and artificial pollination are required for fruits to set normally. After fertilization, the levels of hormones that are positive for fruit development, such as auxin, increase, whereas the levels of ethylene in the fruit decrease (Pandolfini, 2009). Additionally, even when an exogenous hormone treatment is utilized, the expressions of auxins, cytokinins, and gibberellins increase, enabling fruit setting (Li et al., 2014). This process increases the labor costs but is not necessary when a cultivar with a parthenocarpic trait is used. In the absence of pollination or a treatment, the production of plant-aging hormones, such as ethylene and ABA, in the ovaries of the fruit soars and the fruit falls naturally (Martínez et al., 2014; Pomares-Viciana et al., 2017). Cultivars with parthenocarpic traits have a higher generation level of endogenous growth hormones than non-parthenocarpic cultivars, whereas ethylene generation levels are lower than those of non-parthenocarpic cultivars; therefore, fallen fruits do not occur, even when pollination and treatments are not performed (Kim et al., 1992; Gillaspy et al., 1993; Li et al., 2014; Martínez et al., 2014).
In a parthenocarpy study of cucumbers, parthenocarpic cucumbers showed high transcriptional activity of genes involved in pollination and gametophyte development, suggesting that parthenocarpy mimics pollinated fruits, resulting in increased transcript levels of genes involved in auxin, cytokinin, and gibberellin biosynthesis in the ovary, similar to those in PF, and increased levels of endogenous auxins and gibberellins (Li et al., 2014). Furthermore, using genetic resources with parthenocarpic traits, studies are being conducted to identify the genes and genetic signals involved in hormone regulation in parthenocarpy and to analyze transcription factors. The reporting of genetic resources with parthenocarpic traits in various crops is required (Sun et al., 2006; de Jong et al., 2009; Li et al., 2014; Pomares-Viciana et al., 2017; Joldersma and Liu, 2018; Sharif et al., 2022).
A common breeding practice for parthenocarpy is to examine the fruit setting of non-pollinated fruits and judge the parthenocarpic ability using the difference in size from pollinated fruits (Robinson and Reiners, 1999; Pomares-Viciana et al., 2017). Considering the seed development and fruit characteristics of non-pollinated fruits in both cultivars, the two cultivars (17R-1s and 19-7s) used in this experiment were considered to have parthenocarpic traits that suggest high ability (Figs. 2 and 4).
Fruit Development and Morphology
The morphology of UF, developed by means of vegetative parthenocarpy, differed from that of PF in both cultivars (Fig. 4). These differences emerged at the beginning of fruit development after fruit setting (Fig. 5). In general, differences in fruit morphology due to parthenocarpy are different for each cultivar, and fruits developed owing to parthenocarpy have various morphological differences compared with fertilized fruits (Pomares-Viciana et al., 2017; Yoshioka et al., 2017; Hu et al., 2019; Takisawa et al., 2019).
In HF, artificial parthenocarpy was induced, and HF developed a morphology similar to that of PF in both cultivars (Fig. 4). In cucumbers, fruits induced by 2,4-D treatment have morphological differences compared to PF (Hu et al., 2019), and fruits treated with NAA, CPPU, and GA4+7 have shapes and weights similar to those in the PF case (Qian et al., 2018). In immature zucchini parthenocarpic cultivars, HF and UF are similar in shape and size to PF, but in non-parthenocarpic cultivars, HF develops to a size similar to that of UF and is smaller than PF (Pomares-Viciana et al., 2017). Therefore, this suggests that the effects of treatments with growth regulators not only vary depending on the type of hormone used but also on the parthenocarpic ability of the cultivar.
Morphological differences between the UF and other groups tended to vary by cultivar. In the 17R-1s cultivar, PF and HF generally developed pear-shaped fruits that were long and broad in the placenta, whereas UF developed narrow or poorly developed fruits in the placenta (Fig. 4). Therefore, it was determined that there was a difference in the fruit diameter on the fifth and tenth days after anthesis (Figs. 2 and 5).
The 19-7s cultivar also showed differences in their developmental morphology. The fruits of the other groups had a globular shape, whereas those of the UF group were wider (Fig. 4). The results showing that the fruit diameter development of parthenocarpic fruits was the same as that of general fruits and that the fruit length was shorter than that of general fruits are similar to those reported by Takisawa et al. (2019). In the case of strawberries, when ethinyl estradiol and progesterone were applied foliarly at low concentrations, morphological characteristics and TSS tended to be better (Kalantari et al., 2020). Hormone production is activated during seed development after fertilization, leading to fruit development (Ruan et al., 2012; Li et al., 2014). Fruits develop and expand during cell division. During the development of pollinated fruit, the cell division activity is highest in the pericarp and placenta, and developing seeds regulate and promote cell division in the surrounding fruit tissues (Gillaspy et al., 1993; Quinet et al., 2019). Seed development was not observed in UF (Fig. 4). Owing to the lack of seed development, the activity of cell division in the placental region was relatively low, which is thought to have caused morphological differences in UF. When ovules do not generate seeds in parts of a fruit, such as tomato or apple, they form lopsided fruits, in which normal and delayed organ development closely coincides with the presence or absence of seeds, respectively. This finding supports the idea that pollination affects fruit morphology (Varga and Bruinsma, 1986; Gillaspy et al., 1993).
The seeds of 2,4-D-treated fruits developed to the extent that there were no morphological differences between them and the seeds of pollinated fruits (Figs. 2 and 5). Seed development is affected by multi-hormonal regulation by auxins, cytokinins, gibberellins, and brassinosteroids (Ruan et al., 2012). Hormones facilitate the synergistic activity of hormone regulation, and the exogenous application of 2,4-D can increase the expression of gibberellin biosynthesis genes (Sharif et al., 2022). Thus, placental regions can develop normally in HF owing to seed development through a hormone application. In the present study, the application of 2,4-D exogenous hormones to squash effectively facilitated the development of embryos without seeds and formed fruits with shapes and weights identical to those of pollinated fruits.
Fruit Characteristics and Quality
In this study, there were no significant differences in fruit quality, including the TSS content and weight. Knapp et al. (2017) reported that in some species, the quantity and quality of parthenocarpic cultivars are improved relative to those of non-parthenocarpic cultivars, and the TSS content is increased. In this study, there were no significant differences in the TSS, firmness, or weight between the experimental groups within the same cultivar. In a study involving C. moschata in Japan, there was no significant difference in quality between parthenocarpic fruit and pollinated fruit of parthenocarpy cultivars, and the carotene content was relatively low in parthenocarpic fruit during storage (Takisawa et al., 2021). When artificial parthenocarpic fruits were developed through a growth regulator application in non-parthenocarpic squash, the quality of the fruits developed through NAA and CPPU treatments differed, while fruits induced from NAA had higher TSS and myo-inositol contents than general fruits (Ogawa and Takisawa, 2022). In cucumbers, NAA, CPPU, and GA4+7 treatments induced normal parthenocarpy, and the compositional changes during the storage of NAA-induced cucumber fruits were similar to those of pollinated fruits. The fruit firmness of CPPU-treated cucumber fruits increased during storage, but vitamin C and phenolic acid contents decreased (Qian et al., 2018). Additionally, NAA can also improve mango quality in negative environments (Chung et al., 2023). Seedless fruits developed by parthenocarpy have differences in some components compared with normal fruits, but the results differ by season and cultivar (Makrogianni et al., 2017). In this experiment, there were differences between cultivars but there were no significant differences among groups within the same cultivar; therefore, an additional component analysis using samples was considered necessary.
Measuring the area of a fruit cross-section based on digital images (Park et al., 2021) showed no significant differences in the cross-sectional areas of the fruit or placenta among the groups (Table 1). Takisawa et al. (2021) reported that the placental area of parthenocarpic fruit is smaller than that of pollinated fruit, a finding that differs from the results of the present study. The finding that the weight of the placenta of parthenocarpic fruit was relatively low is similar to that obtained in this experiment. The weight of the placenta in the UF group was significantly lower than those in the PF and HF groups; this stemmed from the difference between the amount of placenta and the moisture content due to the inferior development of the placenta in the UF group.
Cell division, seed formation, and early embryonic development occur simultaneously after fruit setting. When cell division proceeds for up to ten days after fertilization in normal fruit, it proceeds in the embryo peripheral integument layers and vascular tissues for seed development, and the activity of cell division is highest in the pericarp and placenta (Gillaspy et al., 1993). In pollinated fruits, the development of the embryo, peripheral integument, and placenta is promoted by seed development, but parthenocarpic fruit, unlike fertilized fruit, does not have developing seeds; thus, the development of the placenta is inferior in UF. In general, the placenta is lost during the cooking and processing of squash. Therefore, the lower weight, narrow placental area, and undeveloped seeds suggest the possibility of reducing the rate of squash flesh loss.
Characteristics of Seeds
Embryos do not develop in fruits generated through parthenocarpy due to the absence of double fertilization. However, depending on the type of parthenocarpy and the specific crop, it is possible to produce seeds without the ability to germinate. While numerous studies have examined fruit morphology and quality with respect to both naturally occurring vegetative parthenocarpy and artificially induced parthenocarpy (Li et al., 2014; Hu et al., 2019), research that focuses on seeds remains relatively insufficient. In this experiment, there were differences in the characteristics between HF seeds developed with artificial parthenocarpy and UF seeds developed via vegetative parthenocarpy. This suggests that the degree of seed development may vary depending on the type of parthenocarpy, and that a treatment with exogenous hormones can promote seed coat development.
The induction of parthenocarpy through a growth regulator treatment not only develops fruits but also seeds without embryos in cucumber, melon, and watermelon (Elassar et al., 1974; Hayata et al., 1995; Ogawa and Takisawa, 2022). The seeds of fruits induced with artificial parthenocarpy by NAA and CPPU are hollow inside, but there are no morphological differences from normal seeds (Ogawa and Takisawa, 2022), whereas in fruits developed by natural parthenocarpy, only traces remain, as seeds do not develop (Takisawa et al., 2021). Seed development is affected by multi-hormonal regulation by auxins, cytokinins, GAs, and brassinosteroids (Ruan et al., 2012). Hormones facilitate the synergistic activity of hormone regulation, and the exogenous application of 2,4-D can increase the expression levels of GA biosynthesis genes (Sharif et al., 2022). This explains why exogenous hormone treatments can affect seed development. However, because this path of seed development is not a normal path in which double fertilization occurs, it develops without an embryo (Elassar et al., 1974; Hayata et al., 1995; Ogawa and Takisawa, 2022). Compared to work focusing on fruit setting, research that involves transcriptomic and metabolomic analyses of seed setting is insufficient and more on seed setting should be conducted (Ruan et al., 2012).
Seeds are an impediment to the consumption of fruits and vegetables, and the production of seedless fruits using parthenocarpy improves the quality of fresh fruits and their processing (Marr and Gast, 1991; Pandolfini, 2009; Dhatt and Kaur, 2016). To produce seedless fruit, such advances are being studied in various crops, such as watermelon and grapes (Heo et al., 2007; Park, 2011; Kim et al., 2019). In grapes, stenospermocarpy, in which seeds degenerate during development after the pollination of triploid cultivars, is used to produce seedless fruits commercially (Heo et al., 2007; Heo and Park, 2016). Watermelon also uses triploids, and recently efforts have been made to improve the quality of this process by producing seedless fruits using irradiated pollen (Kim et al., 2019). Therefore, research on seedless squash using parthenocarpy may improve the quality of pumpkins used for cooking or processing.
In conclusion, 17R-1s and 19-7s as used in this experiment were judged to be cultivars with parthenocarpic traits, and fruits developed with parthenocarpy had morphological differences from normal fruits. However, there were no significant differences in terms of quality. Additionally, seeds developed by parthenocarpy develop at trace levels and may not interfere with eating. Globally, reports of genetic resources with parthenocarpic traits in C. moschata are scarce. The results here can serve as a valuable genetic resource to be used as materials for genetic analyses of the parthenocarpy of Cucurbitaceae.