Introduction
Materials and Methods
Plant materials
Treatments
Assessments of fruit quality characteristics
Extraction and determination of anthocyanin contents
RNA extraction and expression analysis
Statistical analysis
Results
Fruit quality characteristics
Fruit skin color and anthocyanin contents in fruit skins
Anthocyanin biosynthetic genes
Principal component analysis (PCA) responses
Discussion
Introduction
Red-colored apples are in great demand and are preferred by consumers in the fresh apple market, and apple peels with a high proportion of red coloration are classified as high-quality apples (Dar et al. 2019). Generally, red coloration in the skin of apples is due to the accumulation of anthocyanin pigments (Honda et al. 2002; Espley et al. 2007). Anthocyanins, a group of flavonoids, are commonly found in plants, particularly in the flowers, leaves, and fruits, and have different colors, such as red, blue, and purple (Jaakola 2013; Khoo et al. 2017). Apple anthocyanins are health-promoting pigments and are also a primary source of dietary flavonoids (Wang et al. 2018). Anthocyanins also contribute to apple quality and other characteristics (Honda and Moriya 2018), and redder fruits with better quality and flavor attributes are in high demand and are more marketable (Chen et al. 2021). Hence, fruit color development is a major focus for apple breeders and growers.
In apples, anthocyanin accumulation is influenced by genetic, plant nutrition, and environmental factors, such as light, temperatures, crop loads, the tree canopy, hormones, and rootstocks, whereas sunlight exposure is the most important factor among those that increase anthocyanin pigments in apple skin (Chagné et al. 2016; Serra et al. 2016; Jing et al. 2020; Gao et al. 2021). Many studies have reported that sunlight exposure induces the production of anthocyanin pigments and the expression of the structural and transcription factor genes (MdPAL, MdCHS, MdCHI, MdF3H, MdDFR, MdANS, MdUFGT, and MdMYB10) involved in the anthocyanin biosynthetic pathway (Kondo et al. 2002; Espley et al. 2007; Telias et al. 2011; Meng et al. 2015; Ding et al. 2021; Do et al. 2021). Furthermore, increasing both the light intensity and wavelength affects anthocyanin accumulation in apples (Jaakola 2013; Lu et al. 2015). Hence, anthocyanin accumulation in apple skin is light-dependent, and enhancing sunlight availability to fruits is important for growers to enhance red skin coloration and anthocyanin pigments in apples.
‘Hongro’ (‘Spur Earliblaze’ × ‘Spur Golden Delicious’), a red-colored apple, accounts for 16.7% of the total apple cultivation area and is the second most cultivated apple cultivar in Korea (KREI 2021). ‘Hongro’ is popular among consumers due to its excellent taste and texture (Koh and Kim 2009; Choi et al. 2014; Chung et al. 2021). It is generally harvested in early September and is often given as a gift during the Chuseok holiday, the Korean equivalent of Thanksgiving (USDA 2021). Therefore, producing high-quality ‘Hongro’ apple fruits with superior red skin coloration is economically important and also increases consumer demand during the annual apple harvesting season. Hence, growers use a wide range of methods to improve the coloration and quality of ‘Hongro’ apples.
The most widely used methods for improving skin coloration in apples in Korea are the application of reflective film mulching and the defoliation of fruit cluster leaves by manual or chemical defoliants (Choi et al. 2000; Lee et al. 2016; Yoo et al. 2022). However, apple trees with reflective groundcover applied show increased sunburn incidence when temperatures are high (Willsea et al. 2023). Moon et al. (2022) recently reported that reflective film mulching severely increased the incidence of sunscald in ‘Hongro’ apples. Therefore, it is essential to protect the fruits from high summer temperatures while also providing sufficient sunlight to maintain the optimum fruit color. The application of chemical defoliants before harvest is a method of defoliating apple trees that can improve the light intensity in the canopy and the color development of apples (Lee et al. 2016; Lim et al. 2019a, 2019b). However, some chemical defoliants at high concentrations have environmental safety concerns and require special safety regulations when used. The removal of fruit cluster leaves by the hand-stripping procedure is a widely used method for improving skin coloration in many Korean apple orchards. This method increased light penetration to the fruits, resulting in enhanced red pigmentation (Yim and Lee 1999; Choi et al. 2000). Additionally, the selective removal of fruit cluster leaves has a less pronounced effect on the tree’s overall photosynthetic capacity compared to the removal of leaves from other parts of trees (Lee et al. 2016; Choi et al. 2000). Therefore, the practice of defoliation of fruit cluster leaves is primarily desirable to achieve better fruit coloration with less of a reduction in photosynthates. However, in ‘Fuji’ apples, Matsumoto et al. (2017) reported that early defoliation reduced the enlargement of the fruit size and weight and that late defoliation did not lead to optimal apple coloration. Therefore, the timing of defoliation can influence both the skin color and quality of apples. There is, however, still limited data on the timing of the defoliation of fruit cluster leaves and how it affects the fruit quality and color, especially for ‘Hongro’ apples.
Therefore, the objective of this study was to determine the ideal timing for defoliating fruit cluster leaves to optimize fruit skin color development in ‘Hongro’ apples. Additionally, the accumulation of anthocyanins in the fruit skin and the related gene expression levels involved in the anthocyanin biosynthetic pathway were studied. Lastly, the effects of different defoliation timing treatments on the changes and differences in the physiological traits of apple fruits were evaluated.
Materials and Methods
Plant materials
This study was conducted in an orchard at the Apple Research Institute, Sobo-myeon, Gunwi-gun, Daegu, Korea, in 2022. Seven-year-old ‘Hongro’ apple trees grafted onto M.9 rootstock, with trees planted at a spacing of 3.0 × 1.5 m, were selected for use in this study. The apple trees were planted in the same field under identical soil and environmental conditions. The selected trees were trained into slender-spindle-shaped trees with an average width of 1.0 m and height of 2.8 m. The trees had fully bloomed in the experimental field by April 20, 2022. After 30 days of full bloom, the trees were adjusted to a crop load level of seven fruits per cm2 of trunk cross-sectional area. The experimental field was irrigated regularly with drip irrigation and managed using an integrated pest management system (Win et al. 2023a). Daily meteorological data (temperature, relative humidity, and precipitation) from defoliation to harvest are shown in Supplementary Fig. S1.
Treatments
Thirty-six apple trees for four treatments were used in this study (nine trees per treatment). Each treatment was applied to each apple tree. A completely randomized block design with three replicates was established for each treatment, and three individual apple trees were used in each replicate. Four defoliation treatments, including a control (no defoliation), were assessed in this study. Fruit cluster leaves were manually removed for defoliation (Fig. 1). Defoliation treatments were applied to the fruits located at heights ranging from 0.5 m to 2 m within the canopy. In the present study, the harvesting time of ‘Hongro’ apple was estimated using the starch pattern index (SPI) scoring method based on data from previous years. SPI was measured by cutting the fruits vertically into slices, dipping them into an iodine solution, and scoring them on a scale of one to eight on the Cornell starch index (Blanpied and Silsby 1992). The average SPI score of the ‘Hongro’ apples was 7.50 on September 9 from 2018 to 2021 (data not shown). Therefore, in the present study, ‘Hongro’ apples were harvested on September 9, 2022. Defoliation treatments were performed two, three, and four weeks before harvest (WBH). All defoliation treatments were performed on all apples on the designated treatment dates. These treatments were performed on August 12, 2022, for 4 WBH; on August 19, 2022, for 3 WBH; and on August 26, 2022, for 2 WBH. No defoliation treatment was used as a control.
Assessments of fruit quality characteristics
Firstly, sunburned fruits were counted among the fruits harvested from all apple trees. All types of fruit sunburn disorders, including sunburn browning, sunburn necrosis, and photooxidative sunburn, were used to define a sunburned fruit. The fruit sunburn rate was calculated using the following equation: Fruit sunburn rate (%) = (number of sunburn fruits / total number of fruits before application) × 100, according to Moon et al. (2022). Subsequently, fifteen individual fruits per tree (a total of 135 fruits per treatment from nine trees) were randomly selected for a further analysis of the fruit quality characteristics, in this case the fruit weight, fruit size, fruit skin color, flesh firmness, soluble solid content (SSC), titratable acidity (TA), and starch pattern index (SPI) score. The weight of each fruit was measured using a digital scale. The length and diameter of each fruit were measured using a caliper (CD-15APX, Mitutoyo, Japan). The development of a red-colored area (red-blushed area) on the fruit surface was determined according to the method described by Serra et al. (2016). Fruit skin color values were measured on both sun-exposed and sun-shaded sides of each fruit. The part of fruits with more intense red coloration is referred to as the sun-exposed side, while the part of fruits with less red coloration (more background color) is referred to as the sun-shaded side of the fruit. The fruit skin color values (L*, a*, and b*) were assessed on the sun-exposed and sun-shaded sides of the fruit skin using a chroma-meter (CR-400, Konica Minolta, Japan), following the protocol of Kim et al. (2018).
Next, the peels were removed from three places around the midline of each fruit, and flesh firmness was measured by inserting an 11-mm probe into the fruit using a firmness tester (FT-327, TR Co., Italy). SPI was measured using the Cornell starch method as described above. Next, the fruits were juiced to provide juice samples to assess the SSC and TA. SSC was assessed using a refractometer (PR-201, Atago, Japan), and TA was assessed using a reduction method for malic acid content by titrating the juice sample with a NaOH solution to a pH of 8.1 (Yoo et al. 2014). During the fruit quality assessment, fruit skin tissue samples were collected from the sun-exposed and sun-shaded sides of the fruits for further anthocyanin content measurements.
Extraction and determination of anthocyanin contents
The extraction and determination of the anthocyanin content for each group were conducted using the pH differential method, as described by Elisia et al. (2007) and Giusti and Wrolstad (2001). First, 10 g of each apple skin tissue sample was extracted in 100 mL of 80% acetone, after which this was filtered through filter paper. The filtrate was then evaporated using a rotary evaporator (N-100, Rikakikai, Japan), and the samples were adjusted to 10 mL with distilled water. The samples were mixed with a potassium chloride buffer (pH1.0) and with a sodium acetate buffer (pH4.5). After incubation for 15 min at 20°C, the absorbance was read at 700 and 510 nm using a spectrophotometer (UV-1800, Shimadzu, Japan). The absorbance was calculated as Absorbance = (A510–A700)pH1.0–(A510–A700)pH4.5. Next, the anthocyanin content was calculated as Anthocyanin = (Absorbance × MW × 1000)/ (ε × C), where the molecular weight (MW) is 449.2, the molar mass (ε) is 26,900, and C is the concentration of the buffer solution. Three biological replicates were used for the determination of the anthocyanin content. The unit of anthocyanin content was mg of cyanidin-3-galactoside per 100 g of fresh weight.
RNA extraction and expression analysis
Primers for anthocyanin biosynthesis genes were obtained from Do et al. (2021). The eight major genes (MdPAL, MdCHS, MdCHI, MdF3H, MdDFR, MdANS, MdUFGT, and MdMYB10) involved in anthocyanin biosynthesis were studied. Total RNA was isolated from skin tissues using the CTAB method (Ouyanga et al. 2014). The genomic DNA contamination was removed using a TURBO DNA-free kit (Invitrogen, Carlsbad, CA, USA). Next, the RNA quality and concentration were checked using a UV spectrometer and in 0.8% agarose gel. Total RNA was synthesized to cDNA using a PrimeScriptTM 1st strand cDNA synthesis kit (Takara, Kusatsu, Japan). A qRT-PCR system (LightCycler 48 II; Roche Diagnostics, Mannheim, Germany) was used to analyze the transcript levels of the genes, which were normalized to a reference gene (MdP0000336547) (Zhou et al. 2017). Three biological replicates were used for the gene expression analysis. The primers and PCR conditions are listed in Supplementary Table S1 (Do et al. 2021).
Statistical analysis
All data were subjected to an analysis of variance, and mean differences were compared using Tukey’s HSD test at p < 0.05 with SPSS software (Version 26, IBM SPSS Corp., Armonk, NY, USA). The data are expressed as the mean ± standard error. A principal component analysis (PCA) was conducted using MetaboAnalyst 5.0 (Lwin et al. 2023).
Results
Fruit quality characteristics
Defoliation treatments of the fruit cluster leaves did not affect the fruit weight of ‘Hongro’ apples. Similarly, the defoliation treatments did not improve the indicators of fruit enlargement of ‘Hongro’ apples at harvest, i.e., the fruit length, diameter, or shape (length: diameter ratio; Table 1).
Table 1.
Fruit weight and size of ‘Hongro’ apples at harvest after defoliation treatments of fruit cluster leaves four weeks before harvest (WBH) and at 3 WBH, at 2 WBH, and in the control case (no defoliation)
Defoliation treatments | Fruit weight (g) | Fruit size (mm) | ||
Length (L) | Diameter (D) | L/D ratio | ||
No defoliation | 280.10 ± 8.78z ay | 78.18 ± 1.04 a | 87.46 ± 1.14 a | 0.89 ± 0.02 a |
Defoliation at 2 WBH | 274.06 ± 9.07 a | 74.68 ± 1.70 a | 87.42 ± 0.88 a | 0.88 ± 0.01 a |
Defoliation at 3 WBH | 286.11 ± 15.5 a | 78.41 ± 1.91 a | 88.22 ± 1.63 a | 0.89 ± 0.02 a |
Defoliation at 4 WBH | 287.47 ± 8.00 a | 74.91 ± 1.00 a | 84.81 ± 1.78 a | 0.90 ± 0.02 a |
Table 2.
Fruit weight, flesh firmness, soluble solid content (SSC), titratable acidity (TA), SSC/TA ratio, starch pattern index (SPI) score, and sunburn rate of ‘Hongro’ apples at harvest after defoliation treatments of fruit cluster leaves four weeks before harvest (WBH), at 3 WBH, at 2 WBH, and in the control case (no defoliation)
Defoliation treatments | Flesh firmness (N) | SSC (%) | TA (%) | SSC/TA ratio | SPI (1–8) | Sunburn (%) |
No defoliation | 55.84 ± 0.68z ay | 13.40 ± 0.17 a | 0.24 ± 0.01 a | 55.13 ± 0.81 a | 6.93 ± 0.13 a | 3.54 ± 0.63 b |
Defoliation at 2 WBH | 57.29 ± 0.77 a | 13.41 ± 0.13 a | 0.24 ± 0.01 a | 56.98 ± 1.48 a | 6.86 ± 0.21 a | 4.03 ± 0.34 b |
Defoliation at 3 WBH | 56.62 ± 0.40 a | 13.35 ± 0.16 a | 0.23 ± 0.01 a | 58.99 ± 2.42 a | 6.79 ± 0.18 a | 5.57 ± 0.30 a |
Defoliation at 4 WBH | 55.51 ± 0.91 a | 13.44 ± 0.14 a | 0.23 ± 0.00 a | 58.24 ± 1.38 a | 6.86 ± 0.09 a | 6.01 ± 0.44 a |
Defoliation treatments of the fruit cluster leaves did not enhance the fruit quality attributes of ‘Hongro’ apple at harvest, i.e., the flesh firmness, SSC, TA, or SSC-to-TA ratio (Table 2). In addition, the defoliation treatment did not affect the starch pattern index of the fruit (Table 2). However, early defoliation treatments at 4 and 3 WBH significantly increased the rate of fruit sunburn compared to defoliation at 2 WBH and compared to the non-defoliation treatment. The fruit sunburn rate in the defoliation treatment at 2 WBH did not differ from that in the non-defoliation treatment (Table 2).
Fruit skin color and anthocyanin contents in fruit skins
The fruit samples treated with early defoliation at 4 WBH were found to have the reddest skin coloration at harvest, followed by defoliation at 3 and 2 WBH, whereas the fruit samples from the non-defoliation treatment had the least red skin coloration at harvest (Fig. 2).
The development of red-colored areas on the fruit surface and the skin color a* values on the sun-exposed side were significantly higher in the early defoliation treatments at 4 and 3 WBH, followed by defoliation at 2 WBH, and were the lowest in the non-defoliation treatment (Table 3). The red-colored areas were 19%, 17%, and 9% larger in the 4, 3, and 2 WBH treatments than in the non-defoliation treatment, respectively (Table 3). However, on the sun-exposed side, the L* value was lower in the defoliation treatment than in the non-defoliation treatment. In addition, the b* value was highest in the defoliation treatment at 2 WBH and was higher in the non-defoliation treatment than in the defoliation treatments at 4 and 3 WBH (Table 3).
Table 3.
Red-colored (red-blushed) area on the fruit skin surface and fruit skin color values (L*, a*, and b*) on the sun-exposed and sun-shaded sides of ‘Hongro’ apples at harvest after defoliation treatments of fruit cluster leaves four weeks before harvest (WBH), at 3 WBH, at 2 WBH, and in the control case (no defoliation)
Defoliation treatments | Red-colored area (%) | Fruit color values on sun-exposed side | Fruit color values on sun-shaded side | |||||
L* | a* | b* | L* | a* | b* | |||
No defoliation | 68.57 ± 0.92z cy | 44.52 ± 0.67 a | 21.78 ± 0.81 c | 15.30 ± 0.25 a | 62.69 ± 0.56 a | 1.80 ± 0.99 c | 24.29 ± 0.34 a | |
Defoliation at 2 WBH | 77.86 ± 1.01 b | 40.77 ± 0.46 b | 25.41 ± 0.41 b | 16.51 ± 2.74 a | 60.61 ± 1.06 a | 5.32 ± 1.29 b | 23.45 ± 0.56 a | |
Defoliation at 3 WBH | 85.71 ± 1.30 a | 40.15 ± 0.66 b | 29.76 ± 0.68 a | 14.56 ± 0.19 a | 60.43 ± 0.90 a | 9.47 ± 0.83 a | 23.08 ± 0.49 a | |
Defoliation at 4 WBH | 87.14 ± 1.01 a | 39.47 ± 0.26 b | 31.30 ± 0.24 a | 14.41 ± 0.28 a | 56.26 ± 0.90 b | 11.04 ± 1.81 a | 21.03 ± 0.54 b |
Defoliation also increased the red skin color on the sun-shaded side. The a* value was significantly increased after the defoliation treatments at 4 and 3 WBH, followed by defoliation at 2 WBH, and was lowest in the non-defoliation treatment (Table 3). However, the L* and b* values were lower after defoliation at 4 WBH compared to the other treatments (Table 3).
The defoliation treatments significantly increased anthocyanin accumulation in ‘Hongro’ apples (Fig. 3). On the sun-exposed side, the anthocyanin content was highest under defoliation at 4 WBH, followed by defoliation at 3 and 2 WBH, and was lowest in the non-defoliation treatment (Fig. 3A). On the sun-shaded side, the anthocyanin content was highest under defoliation at 4 and 3 WBH, followed by defoliation at 2 WBH, and was lowest in the non-defoliation treatment (Fig. 3B).
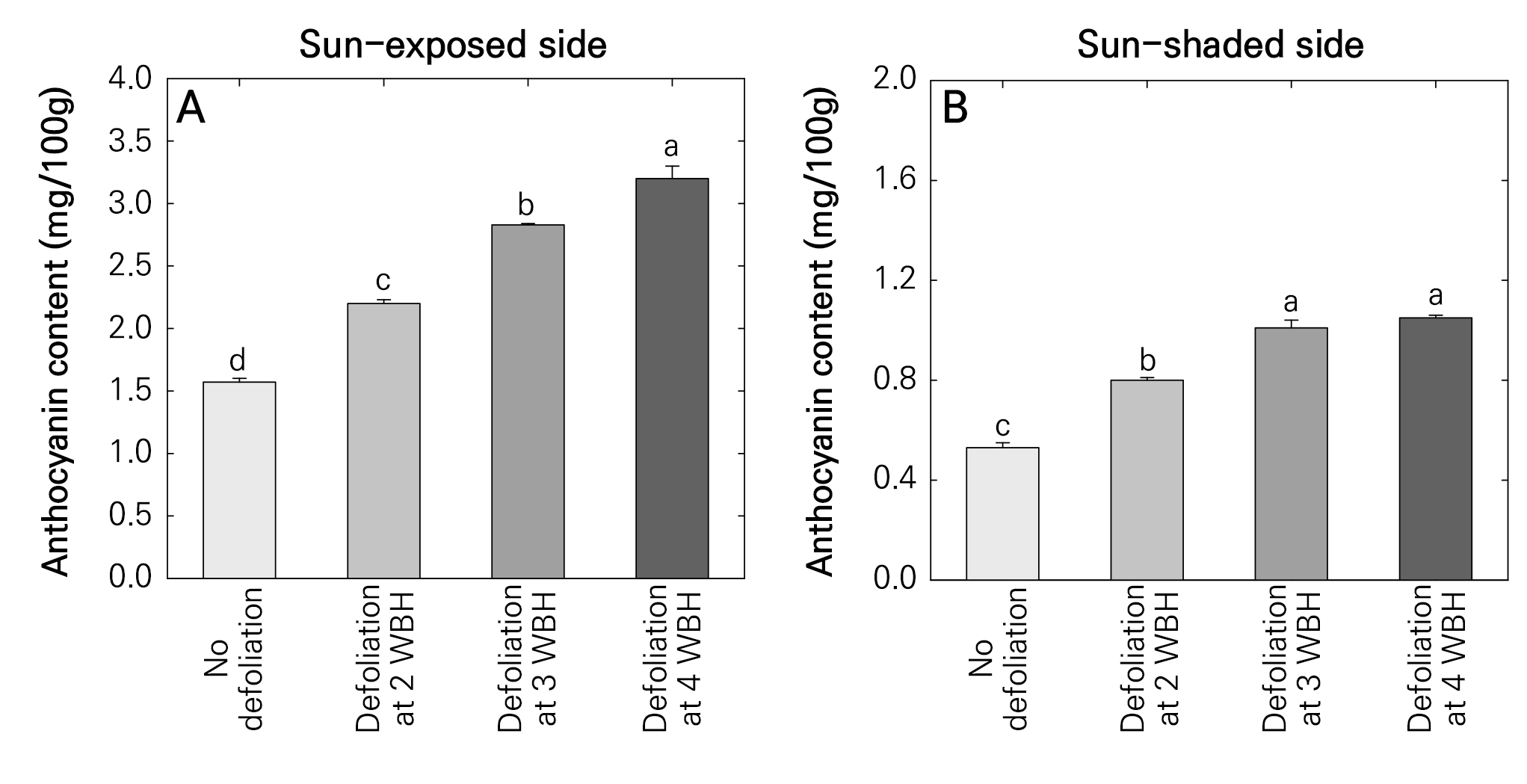
Fig. 3.
Anthocyanin contents in the fruit skin tissues of the sun-exposed (A) and sun-shaded (B) sides of ‘Hongro’ apples produced from defoliation treatments of fruit cluster leaves four weeks before harvest (WBH) on August 12, 2022 and at 3 WBH on August 19, 2022 and at 2 WBH on August 26, 2022. No defoliation was used as the control. Data are the mean ± standard error (n = 3). Different letters indicate significant differences according to Tukey’s HSD test (p < 0.05).
Anthocyanin biosynthetic genes
The expression of all genes (MdPAL, MdCHS, MdCHI, MdF3H, MdDFR, MdANS, MdUFGT, and MdMYB10) was upregulated in the skin from both the sun-exposed and sun-shaded sides in all treatments (Fig. 4). On the sun-exposed side, the expression of all genes, except MdPAL and MdF3H, was significantly higher in the defoliation treatments at 4 and 3 WBH than in the non-defoliation treatments (Fig. 4A–4H). Higher expression of MdUFGT and MdMYB10 was also observed in the defoliation at 3 WBH than at 2 WBH (Fig. 4G and 4H). Except for MdCHS, non-significant expression of all genes was observed between defoliations at 4 and 3 WBH (Fig. 4A–4H).
On the sun-shaded side, the expression of all genes, except MdCHI, was significantly higher in the defoliation treatment at 4 WBH than in the non-defoliation treatment (Fig. 4I–4P). Higher expression levels of MdANS, MdUFGT, and MdMYB10 were also observed after the defoliation treatments at 4 and 3 WBH compared to defoliation at 2 WBH (Fig. 4N–4P). The expression levels of MdPAL, MdCHS, MdANS, and MdMYB10 were higher in the 2 WBH defoliation treatment than in the non-defoliation treatment (Fig. 4I, 4J, 4N, and 4P). A similar expression pattern, without a statistically significant difference, was observed in all genes between the defoliation treatments that were applied at 4 and 3 WBH (Fig. 4I–4P).
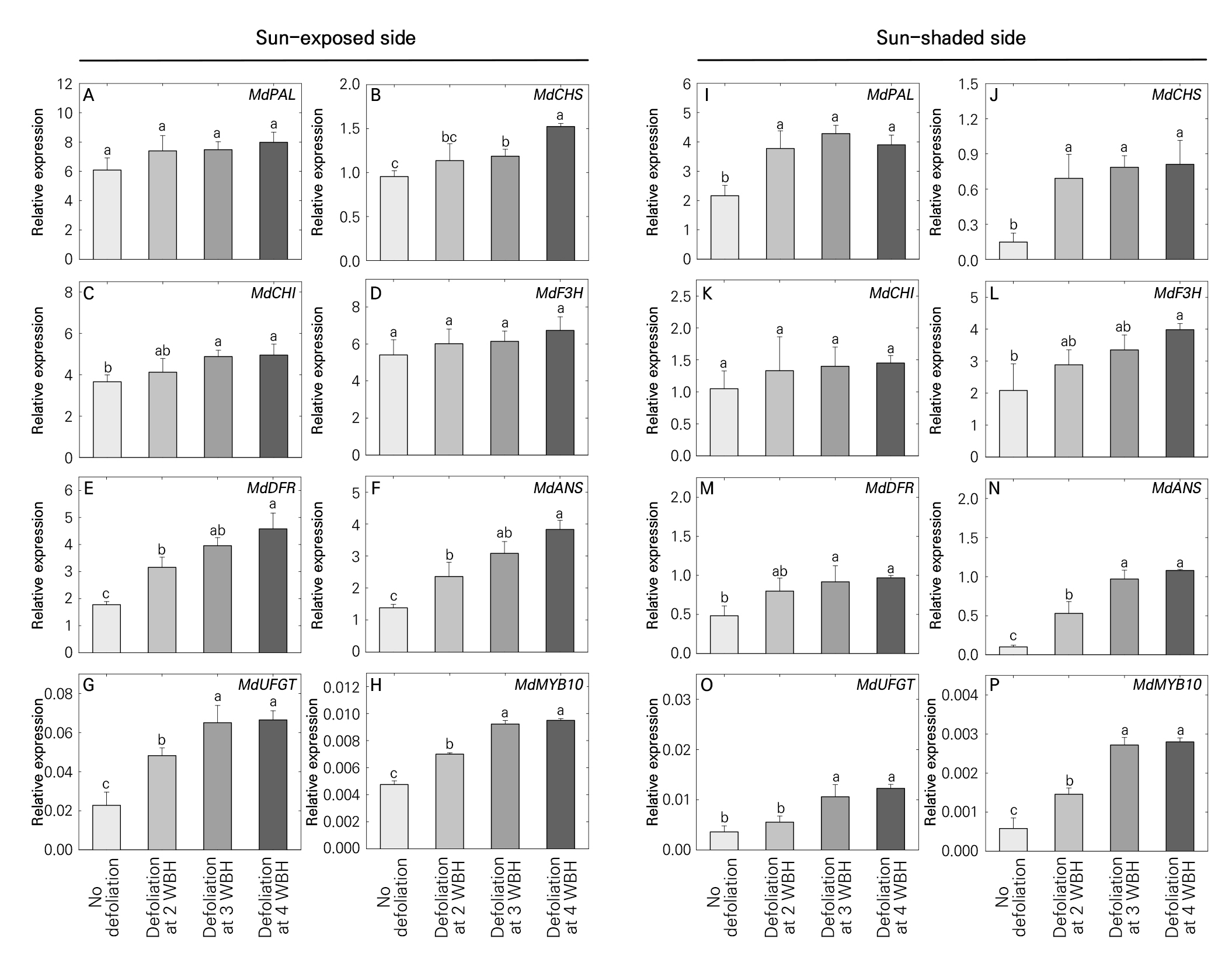
Fig. 4.
Results of relative expression analyses of anthocyanin biosynthetic genes (MdPAL, MdCHS, MdCHI, MdF3H, MdDFR, MdANS, MdUFGT, and MdMYB10) from the sun-exposed side (A–H) and sun-shaded side (I–P) of ‘Hongro’ apple skins. Defoliation treatments of fruit cluster leaves were applied four weeks before harvest (WBH) on August 12, 2022, at 3 WBH on August 19, 2022 and at 2 WBH on August 26, 2022. No defoliation was used as the control. Data are the mean ± standard error (n = 3). Different letters indicate significant differences according to Tukey’s HSD test (p < 0.05).
Principal component analysis (PCA) responses
PCA scores and loading plots were used to evaluate the overall responses of the fruit quality, fruit skin color, anthocyanin content, and expression of anthocyanin genes induced by the different defoliation timing treatments (Fig. 5). The total variance of the PCA scores and the loading plots accounted for 51.2% and 16.5% for PC-1 and PC-2, respectively (Fig. 5). The plot of the PCA scores showed a spatial segregation of treatments, indicating that the application timing of the defoliation treatments distinctly impacted the fruit quality and color of ‘Hongro’ apples (Fig. 5A). Additionally, defoliation at 4 WBH exhibited the most dispersion, while defoliation at 2 WBH exhibited the least dispersion compared to the non-defoliation treatment. Defoliation at 3 WBH resulted in more dispersion than defoliation at 2 WBH and the control group but less than defoliation at 4 WBH (Fig. 5A). The PCA loading plot showed a strong relationship between anthocyanin biosynthetic genes and the anthocyanin content (Fig. 5B). The tight grouping of anthocyanin biosynthesis genes in the same region indicated that these genes are likely co-regulated. Additionally, clustering of the anthocyanin biosynthetic genes and anthocyanin content was observed as well in the same region of the PCA loading plot. This result also suggested that the genes associated with anthocyanin biosynthesis are upregulated in treatments with higher anthocyanin contents, with these also likely being responsible for the biosynthesis of anthocyanin in the fruit skin (Fig. 5B).
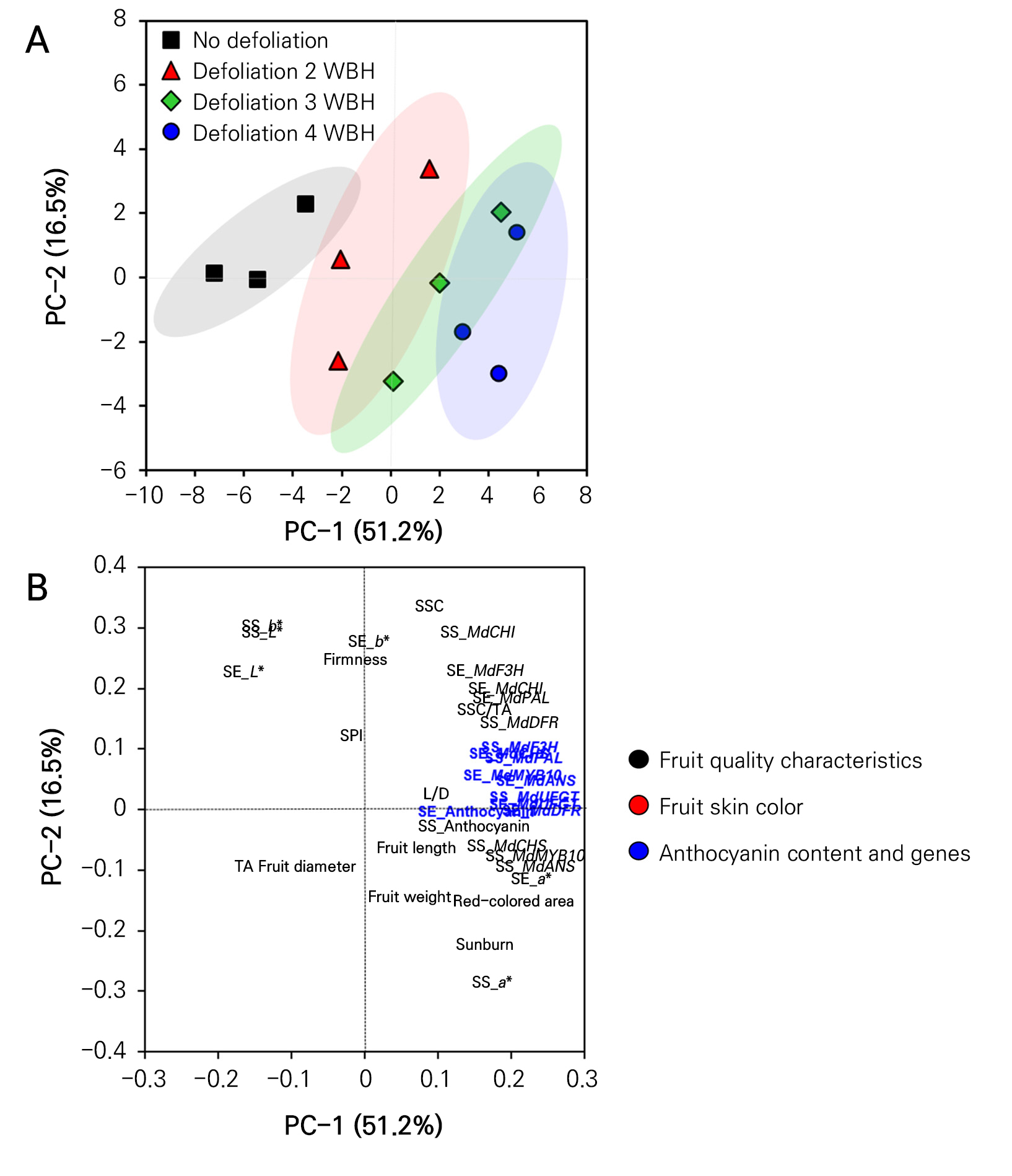
Fig. 5.
Principal component analysis (PCA) scores (A) and loading (B) plots of response variables of ‘Hongro’ apples treated with non-defoliation (control) and defoliation treatments of fruit cluster leaves four weeks before harvest (WBH) on August 12, 2022, at 3 WBH on August 19, 2022, and at 2 WBH on August 26, 2022. Three replicates were used for the response variables of the PCA score plot. SSC: soluble solid content, TA: titratable acidity, SPI: starch pattern index, L/D: fruit length: diameter ratio, SE: sun-exposed side, SS: sun-shaded side.
Discussion
The main function of leaves is to produce nutrients for plants through photosynthesis (Kang et al. 2023), and the nutrients produced from leaves are generally translocated to the fruits during the fruit maturation period (Loescher et al. 1990). Hence, defoliation influences fruit growth and nutrient content outcomes, and excessive defoliation of plant leaves can decrease the enlargement, biochemical characteristics, and nutritional values of fruits when they are harvested (Iqbal et al. 2012). In Korea, the selective removal method of fruit cluster leaves in apple trees before harvest is commonly practiced by growers to improve fruit skin coloration of apples (Yim and Lee 1999; Choi et al. 2000; Lee et al. 2016).
In this study, defoliation of fruit cluster leaves did not affect the fruit size or weight of ‘Hongro’ apples. Matsumoto et al. (2017) reported that defoliation of fruit cluster leaves of ‘Fuji’ apples at 1.5 and 2.0 months before harvest produced small-sized fruits at harvest. However, Lim et al. (2019a) observed that chemical defoliants applied 30 days before harvest did not reduce the weight of ‘Fuji’ apples. Win et al. (2023a) also reported that defoliation before 3 WBH did not affect fruit growth or development in ‘Picnic’ apples at harvest. Therefore, decreases in fruit weight and size are strongly influenced by the defoliation timing, the cultivar, and the defoliation rate in apple trees.
Different defoliation timings of fruit cluster leaves did not decrease the fruit quality characteristics of the flesh firmness, SSC, TA, the SSC-to-TA ratio, or the starch pattern index score. Similar to this study, defoliation of fruit cluster leaves 1.5 and 2.0 months before harvest did not affect flesh firmness, acidity, or the sugar-to-acid ratio in ‘Fuji’ apples at harvest (Matsumoto et al. 2017). However, it was also reported that early defoliation decreased the SSC at harvest. Unlike in earlier work, SSC was not affected by the defoliation timing treatment of ‘Hongro’ apples in this study. However, Lim et al. (2019a) found that the application of chemical defoliants one month before harvest did not reduce the SSC in ‘Fuji’ apples. Win et al. (2023a) observed that pneumatic defoliation applied at 3 WBH did not affect the SSC or TA values in ‘Picnic’ apples. Additionally, the responses of the fruit quality characteristics to defoliation could vary depending on the apple cultivar used and the timing of the defoliation treatment applied, and defoliation near harvest does not affect apple quality outcomes, as observed in this study. Moreover, the selective removal of fruit cluster leaves contributes less to the tree’s overall photosynthetic efficiency, with the leaves remaining on the tree still able to produce sufficient carbohydrates for fruit development (Lee et al. 2016; Choi et al. 2000). Therefore, the ineffective results of fruit enlargement and sugar accumulation in apples may be due to the aforementioned factors observed in this study. Defoliation of fruit cluster leaves, regardless of its timing, did not noticeably affect the fruit growth or quality of ‘Hongro’ apples.
Sunburn is a physiological disorder caused by excessive light irradiance and excessive temperatures, resulting in oxidative damage to fruits (Felicetti and Schrader 2008; Munné-Bosch and Vincent 2019). Overexposure to direct or reflected sunlight induces sunburn disorders in apples (Chen et al. 2008; Xue et al. 2021; Moon et al. 2022). In this study, the sunburn rate of fruits increased slightly in the early defoliation treatments at 4 and 3 WBH compared to those at 2 WBH and in the control group. Therefore, the increased occurrence of sunburn after the defoliation treatments at 4 and 3 WBH may be due to the increased exposure of the fruit skin to direct sunlight and the high-temperature threshold levels that arose when the fruit cluster leaves that covered the surfaces of the fruit skin were removed. The lower sunburn rate of fruits in the late defoliation treatment at 2 WBH may be due to the removal of fruit cluster leaves at a lower temperature threshold level or to the treatment application close to the harvest time.
In apple skin, cyanidin-3-galactoside is the main type of anthocyanin identified, accounting for more than 85% of all anthocyanins (Treutter 2001; Kondo et al. 2002; Feng et al. 2014). Lee et al. (2016) reported that defoliation of fruit cluster leaves improved apple fruit quality and red skin coloration in ‘Fuji’ apples. Additionally, Matsumoto et al. (2017) reported that fruit cluster leaf defoliation in ‘Fuji’ apple trees induced anthocyanin accumulation. In this study, the monomeric cyanidin-3-galactoside anthocyanin content was significantly increased during early defoliation at 4 WBH on the sun-exposed side and at 4 and 3 WBH on the sun-shaded sides. The levels in the defoliation treatment at 2 WBH were also higher than those of the non-defoliation treatment but were lower than those that at 4 and 3 WBH on both the sun-exposed and sun-shaded sides. Consequently, the development of red-colored areas and the a* values in apple skin also increased in defoliated apple fruits. Similar to the results of this study, higher a* values and lower L* and b* values were also reported in chemically defoliated ‘Fuji’ apple trees (Lee et al. 2016; Lim et al. 2019a). Thus, defoliation of fruit cluster leaves enhances a* values and red color pigments in apple skin, and early defoliation induces the production of more pigments than late defoliation or non-defoliation treatments.
The ripening phase is an important aspect of pigment accumulation, and a progressive increase in anthocyanin accumulation generally occurs in apples (Kondo et al. 2002). The molecular analysis in this study also confirmed that the transcript levels of MdPAL, MdCHS, MdCHI, MdF3H, MdDFR, MdANS, MdUFGT, and MdMYB10 were upregulated on both the sun-exposed and sun-shaded sides of apple skin in all defoliation treatments and that early defoliation induced higher expression levels than late defoliation or non-defoliation treatments. Similar to this study, Win et al. (2023b) reported that defoliation before harvest increased light availability to the fruit, thereby enhancing anthocyanin contents and the expression of anthocyanin biosynthetic genes in ‘Picnic’ apples. Telias et al. (2011) also reported that the transcript levels of anthocyanin biosynthetic genes were upregulated when the anthocyanin content in ‘Honeycrisp’ apple skin increased. Anthocyanin synthesis is controlled by structural genes (Kitamura et al. 2004), and the expression levels of five major genes (MdCHS, MdF3H, MdDFR, MdANS, and MdUFGT) are responsible for anthocyanin accumulation (Ubi et al. 2006); the MdUFGT gene is particularly important for anthocyanin accumulation in apple skin (Meng et al. 2015).
MdMYB10 is a transcription factor gene in apples, with the corresponding gene expression influenced by light exposure (Meng et al. 2015). Light-induced upregulation of the MdMYB10 gene further stimulates the expression of the key genes (MdPAL, MdCHS, MdPAL, MdCHS, MdCHI, MdF3H, MdDFR, MdANS, and MdUFGT) involved in the anthocyanin biosynthesis pathway (Espley et al. 2007; Meng et al. 2015; Chen et al. 2019). Moreover, Espley et al. (2007) and Telias et al. (2011) reported that red coloration in apples is mainly due to the activity of the MdMYB10 transcription factor and that a higher expression level of the MdMYB10 gene results in more intense red coloration in apple skins. In the present study, the expression levels of anthocyanin biosynthetic genes were upregulated, especially in the early defoliation treatments at 4 and 3 WBH, compared to those at 2 WBH and in the non-defoliated treatments. Hence, the higher expression levels of anthocyanin biosynthetic genes observed in ‘Hongro’ apples from defoliated trees are attributable to the increased light exposure to the fruit surface stemming from the removal of the fruit cluster leaves, resulting in increased anthocyanin levels in the apple skins. Consequently, the higher anthocyanin content observed in early defoliated treatments compared to late or non-defoliated treatments may be due to the greater light exposure from the time of the defoliation treatment application until harvest. In addition, Do et al. (2021) reported that the transcript levels of anthocyanin genes increased more on the sun-exposed side than on the sun-shaded side of ‘Arisoo’ apples, as observed in the present study with ‘Hongro’ apples. The PCA loading plot showed that most anthocyanin biosynthetic genes were closely associated with the anthocyanin content. These results indicate that anthocyanin biosynthetic genes regulated the activity of key enzymes in the anthocyanin biosynthesis pathway to produce increased anthocyanin pigments in apple skins. Moreover, the plot of the PCA scores showed a clear separation between non-defoliation and defoliation treatment groups, whereas early defoliation treatments at 4 and 3 WBH showed higher dispersion levels, especially compared to the non-defoliation treatment. These results also suggest that the defoliation timing of fruit cluster leaves greatly influences the fruit quality and skin coloration of apples and that the higher dispersion observed in the early defoliation treatments may be due to the increased skin coloration and associated anthocyanin pigments resulting from the early defoliation timing.
In conclusion, the timing of fruit cluster leaf defoliation strongly influenced the development of red skin coloration in apples. Early defoliation at 4 and 3 WBH enhanced red skin color, a* values, and anthocyanin content on both sun-exposed and sun-shaded sides remarkably. Additionally, the expression levels of anthocyanin biosynthetic genes were upregulated in all defoliation treatments, particularly in the defoliation treatments at 4 and 3 WBH. However, the L* and b* values decreased in the apple skins and the fruit sunburn rate increased in the early defoliation treatments. Importantly, defoliation timing did not affect fruit enlargement or the fruit quality characteristics. Overall, this study indicates that defoliation of fruit cluster leaves applied at 4 and 3 WBH caused anthocyanin pigments to accumulate in the apple skins, thereby enhancing the red skin coloration of ‘Hongro’ apples. Therefore, the results here can help growers and researchers to identify the ideal defoliation timing of fruit cluster leaves to use to achieve optimal skin coloration of ‘Hongro’ apples, though the defoliation timing should be carefully considered so as to minimize the increased sunburn incidence, depending on the light and temperature conditions of the apple orchard in question.
Supplementary Material
Supplementary materials are available at Horticultural Science and Technology website (https://www.hst-j.org).
- HORT_20250010_Fig_S1.pdf
Supplementary Fig. S1. The meteorological data (from defoliation to harvest time) including daily air temperature (A), relative humidity (B), and precipitation (C) of the experimental field located in the Gunwi-gun, Daegu, Korea, in 2022. Black-, red-, and blue-colored arrows indicate the application dates of defoliation timing treatments of fruit cluster leaves at four weeks before harvest (WBH) on August 12, 2022, at 3 WBH on August 19, 2022, and at 2 WBH on August 26, 2022. No defoliation was used as the control. Green-colored arrow indicates the harvesting time of ‘Hongro’ apples on September 9, 2022.
- HORT_20250010_Table_S1.pdf
Supplementary Table S1. Primer sequences of the genes and PCR conditions used in this study