Introduction
Materials and Methods
Plant Materials
Floral Morphology and Pollination Biology
Scanning Electron Microscopy (SEM)
Light Microscopy (LM)
Fluorescence Microscopy
Statistical Analysis
Results
Floral Morphology
Blooming Sequence and Stigma Morphology at Different Anthesis Durations
Pollen Morphology and Pollination Biology
Discussion
Introduction
Red-fleshed dragon fruit (Hylocereus polyrhizus) is an oblong-shaped berry that is medium-large in size with a scaly pink peel. The exotic fruit is not only appealing on the outside but also is nutritionally rich with betalains, a source of antioxidant and a natural food colourant (Ding et al., 2009). The vine cacti plant is generally cultivated openly in Malaysia, Thailand, and Vietnam, often with grower’s intervention for fruit setting, and has become increasingly important as a commercial value crop (Hafiz et al., 2019; Metz et al., 2000). The plant is a self-incompatible diploid that requires cross-pollination, and the economic value depends on the fruit weight, which can be achieved with assisted pollination (Weiss et al., 1994; Lichtenzveig et al., 2000). Cross-pollination with compatible pollen groups are necessary for efficient fruit set in self-incompatible Hylocereus species.
Besides reproductive biology of the flower and its timing, pollen and/or pollinator availability and the resources required to ensure fecundity are generally involved in pollination and fertilization towards the fruiting journey (Bustamante et al., 2010). There are also other intricate factors involved in the pollination process such as stigma morphology, pollen-stigma interactions, and pollen tube growth (Sharma and Bhatla, 2013; Zheng et al., 2018). In self-incompatible pollination, stigma features play crucial roles that initiate recognition and subsequent perception or rejection of self-pollen (Haasen and Goring, 2010; Allen et al., 2011). The highly selective process in pollen-stigma interaction determines the compatible and/or incompatible pollination (Gao et al., 2010). Once germinated, the downward trip of pollen tube growth towards the female gametophyte involves various cell-to-cell signalling that determines whether the journey is fruitful or not.
Understanding the reproductive biology and pollination mechanism is important to achieve maximum productivity especially in commercial fruit production. Previous studies on H. polyrhizus were carried out in a controlled environment that demonstrated the importance of nocturnal and diurnal visitors in the cross-pollination and mating systems of the plant (Nerd et al., 1999; Lichtenzveig et al., 2000; Valiente-Banuet et al., 2007). In this study, floral structure, morphological characteristic of stigma and pollen, and the reproductive process (pollen-stigma interaction and pollen tube growth) of H. polyrhizus cultivated in an open field were studied to understand the flowering and pollination mechanisms associated with self-incompatibility of the plant.
Materials and Methods
Plant Materials
The study was carried out on an organic farm of 5-year-old H. polyrhizus in Sepang (2.6914° N, 101.7505° E), Selangor, Malaysia. The study area experienced a tropical hot and humid climate with annual rainfall of 736.24 mm and mean annual temperature of 24.8°C (average maximum temperature at 30°C and average minimum temperature at 24°C). The plants were grown in sandy loam soil and planted at a distance of 2.5 m x 2.5 m supported by a cement pole of 1.5 m in height with three to four plants per pole. The flowering morphology and pollination biology of the plant were studied. Thirty flowers from 10 supporting poles were tagged and monitored before, during, and after anthesis for a total of 4 days. Sequential phases of floral biology were digitalized at different intervals during observation.
Floral Morphology and Pollination Biology
The floral characteristics of H. polyrhizus were observed for 4 days at various durations of anthesis. During anthesis, the floral length of 10 individual flowers was measured from petal tip to flower base. Tepals of the flower were carefully removed, and the shape and colour of tepals were observed and categorized. The number of tepals, as well as its length and width, were measured. The distance between anthers and stigma; the length and number of stigma lobes, style length, and diameter; and the number of stamens per flower were also determined.
The morphology of stigmas of unpollinated flowers was studied at different time intervals: 1900 h (pre-anthesis), 2200 h (during anthesis), and 0900 h (the next day as post-anthesis). The pistil of pollinated flowers was also studied for pollen-stigma interaction. At 1800 h pre-anthesis, flowers were tagged, and the stigmas were covered with a plastic tube. Freshly dehisced pollen grains were collected manually with a brush from neighbouring flowers of H. polyrhizus at anthesis, between 2100 and 2300 h, and then transferred onto the stigma and covered with plastic tube again. The pollen-stigma interaction was studied at 0, 1, 2, 3, and 4 days after pollination (DAP), whereas the growth of the pollen tube was studied at 0, 2, and 4 DAP.
The morphology of pollen was studied by collecting freshly dehisced pollen grains from three individual flowers during anthesis. The polar axis and equatorial diameter were measured and recorded from 25 pollen grains to determine the pollen size and shape according to Erdtman (1986). Surface ornamentation and germ pore of the pollen were also observed.
Scanning Electron Microscopy (SEM)
Pollen grains collected from flowers during anthesis were placed on adhesive tape attached to an aluminium stub and dried in a desiccator. Stigmas of pollinated and unpollinated flowers were fixed in FAA (37% formaldehyde, 100% acetic acid, 80% alcohol) for 24 h and placed in a vacuum pump to remove air from tissue (Lichtenzveig et al., 2000). Samples were rinsed thoroughly with distilled water and then post-fixed in 1% osmium tetraoxide for 2 h, followed by a series of dehydration treatments in graded alcohol (30, 50, 70, 75, 90, 95, and 100%), and then subjected to critical point drying (Baltec CPD 030, Germany). Both samples of dried pollen grains and stigma were sputter-coated (Polaron E5100, USA) with gold and examined using a scanning electron microscope (JEOL JSM-5610 LV SEM, Japan) at an accelerating voltage of 15 kV.
Light Microscopy (LM)
The pistils of pollinated flowers were fixed in FAA according to Lichtenzveig et al. (2000). Samples were dehydrated through a graded series of alcohol (30, 50, 70, 75, 90, 95, and 100%) and then infiltrated with methyl benzoate containing 1% celloidin. The samples were subsequently cleared in xylene and gradually infiltrated in serial ratios of xylene and paraffin (3:1, 1:1, 1:3, and 0:1) overnight for each ratio. The embedded and solidified blocks were sectioned with a thickness of 12 µm using a rotary microtome (SM2000 R, Leica Biosystems, USA). Serial sections of the style were stained with Toluidine Blue O and viewed under a light microscope (Leitz Wetzlar, Germany).
Fluorescence Microscopy
Transverse and longitudinal sections of fixed styles were produced from the top (near the stigma), the middle part of style (10 cm below the stigma), and the style base (near the ovary) using a razor blade. The samples were washed and softened in 1.0 mol·L-1 of NaOH overnight at room temperature (25 ± 2°C) (modified from Martin, 1959). Sections were subsequently washed and soaked in deionized water for 2 h and then stained with 1.0% decolourized aniline blue in 0.1 mol·L-1 of potassium phosphate (K3PO4) solution overnight. Samples were gently squashed under a coverslip and viewed under a Leica DMRA II fluorescence microscope (Germany) equipped with a UV excitation filters at a wavelength of 356 nm.
Statistical Analysis
The collected measurement was analyzed using a standard deviation of Excel Formula where necessary.
Results
Floral Morphology
From our observation, flower buds emerging from the areoles of mature shoots took 3–4 weeks to grow and transform into flowers. The flowers of H. polyrhizus are white and sessile that bloom at night as captured at 2200 h (Fig. 1A). The flower is monoecious, containing both male and female organs and a long floral tube (Fig. 1B) with an average length of 27.8 ± 0.79 cm (unpublished data). The flower perianth is formed by two types of tepals, namely, the sepaloid tepal, which covers the floral tube, and the petaloid tepal, which is attached around the rim of the flower cup. The sepaloid tepals are formed by 33 ± 6 ensiform yellow tepals and 25 ± 7 greenish tepals of various shapes ranging from ensiform to narrowly triangular (Fig. 1C and Table 1). The ensiform sepaloid tepal is about 12 cm long and 1 cm wide, while the narrowly triangular sepaloid tepal is shorter in length and broader in width than ensiform sepaloid tepals. The petaloid tepals, on the other hand, are formed by 24 ± 2 white lance-ovate inner tepals surrounded by 5 ± 1 white-yellowish lanceolate outer tepals. Both white and white-yellowish petaloid tepals are about 10 cm long, but white petaloid tepals are broader than the white-yellowish petaloid tepals.
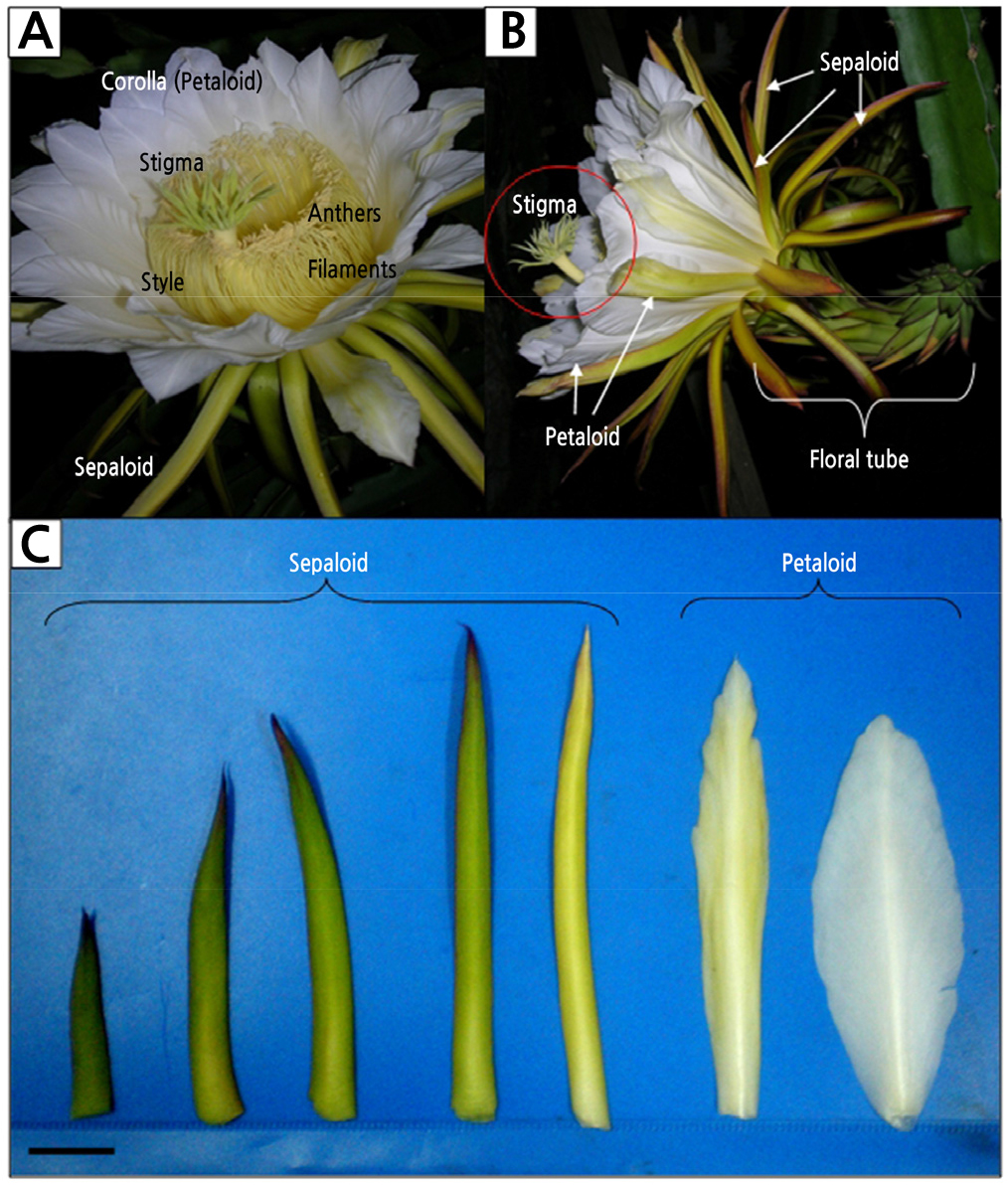
Fig. 1.
Flowers of Hylocereus polyrhizus at anthesis (2200 h). (A) Both male and female organs coexist in a flower. (B) Lateral view of a flower, emphasizing the spatial distance of stigma and anthers (in red circle), suggesting xenogamy behaviour. (C) Various types of sepaloid and petaloid tepals. Scale bar represents 2 cm.
Table 1.
Number, length, width, and shape of sepaloid and petaloid tepals of Hylocereus polyrhizus flowers
The flowers are zygomorphic as the style and stamens are located in the ventral part of the flower (Fig. 2A). The basifixed yellow anthers are 0.48 ± 0.04 cm long, borne on projecting yellow filaments with an average length of 8.04 ± 0.48 cm (Fig. 2C and Table 2). Stamens are apostemonous (distinct and free) with an average number of 1016 ± 69 stamens per flower (Fig. 2C). Upon closer observation, the numerous stamens are arranged in a spiral pattern attached to the surface of the floral tube (Fig. 2D).
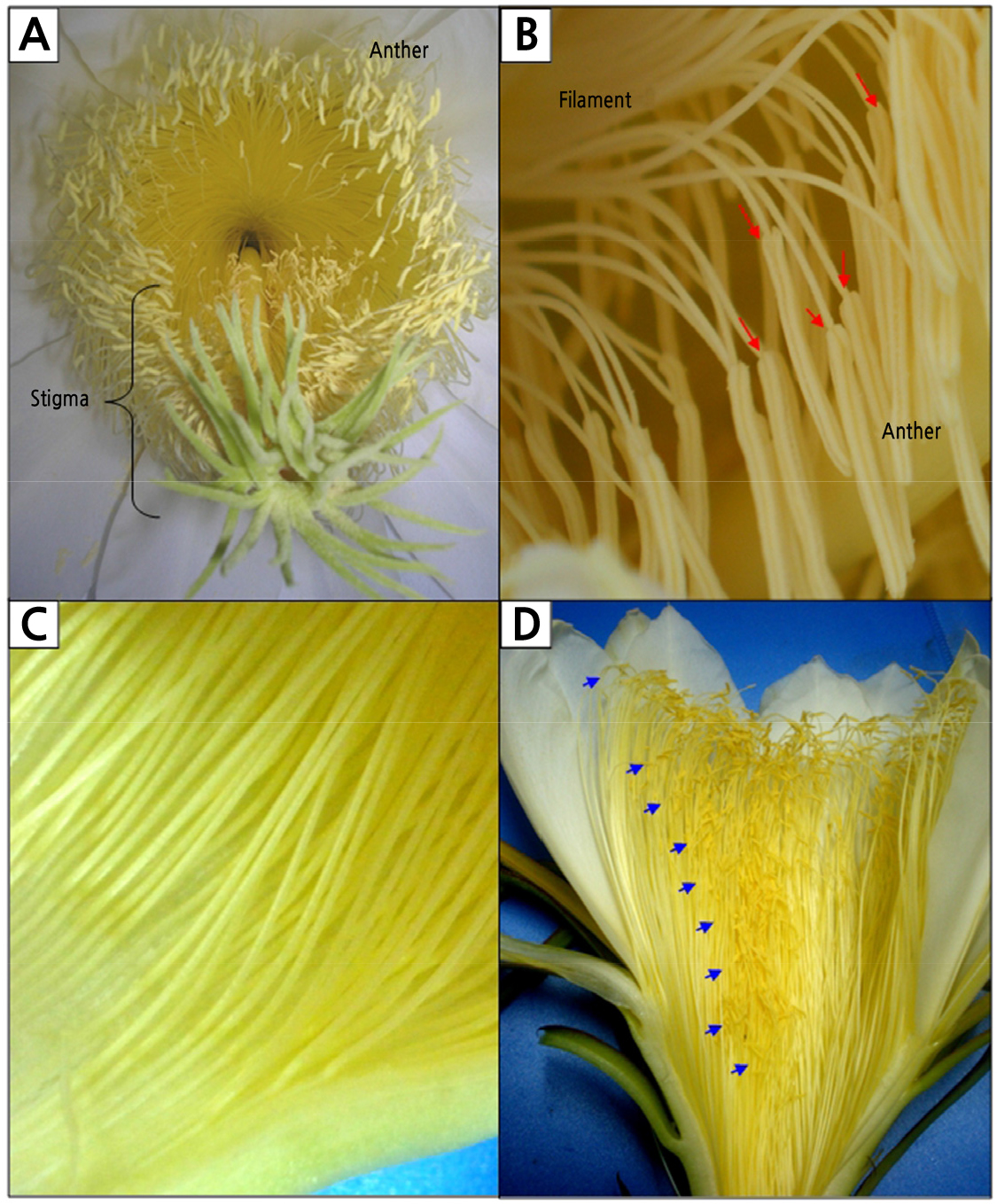
Fig. 2.
Androecium of Hylocereus polyrhizus. (A) Surface view of flowers suggesting zygomorphism as stamens and styles are located in the ventral part of the flower. (B) Basifixed anthers (black arrows) are attached to a long filament. (C) Stamens are apostemonous and attached on the inner surface of the floral tube. (D) Numerous stamens were arranged in a spiral pattern (blue arrows).
Table 2.
Anther and filament length, and stamen number of Hylocereus polyrhizus flowers
Anther length (cm ± SD) | Filament length (cm ± SD) | Stamen number (mean ± SD) |
0.48 ± 0.04 | 8.04 ± 0.48 | 1016 ± 69 |
The flowers contain one long style measuring 27 ± 2.54 cm long and 0.63 ± 0.07 cm wide (Fig. 3A and Table 3). The style is light yellow with the length as long as its floral tube. The style connects the stigma on top to its ovary at the bottom. The mature stigma of H. polyrhizus consisted of 24 ± 2 stigma lobes radiating from the terminal rim of the tubular style (Fig. 3B). Each stigma lobe is 1.65 ± 0.11 cm long. The opening of the style canal could be observed in the centre of the radiating stigma lobes. The lining of the canal of the style was covered with a layer of papillae (Fig. 3C). The spatial difference between stigma and anthers is 1.66 ± 0.53 cm with stigma positioned above the anthers (Fig. 1A and Table 3).
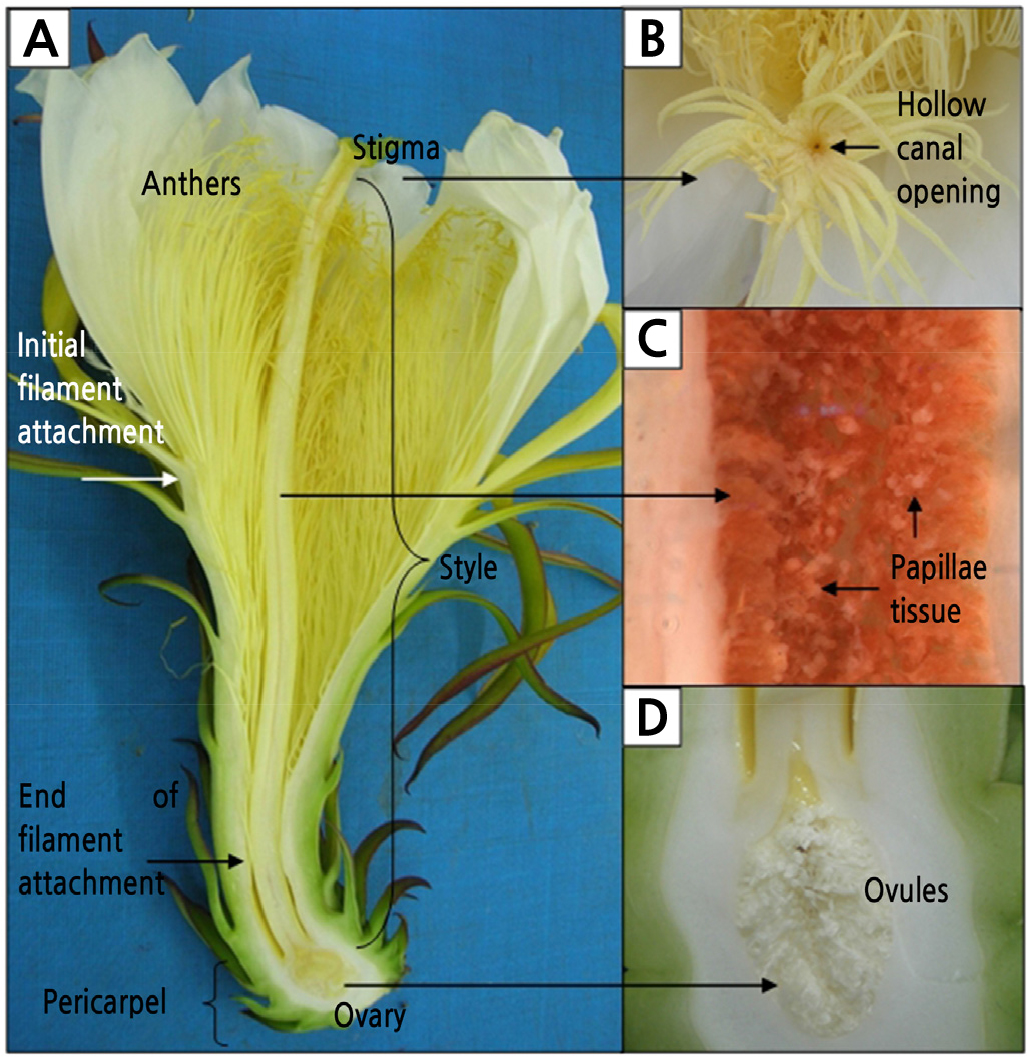
Fig. 3.
Flower parts of Hylocereus polyrhizus. (A) A longitudinal section of a flower. (B) Stigmas consisted of numerous stigma lobes connected to the ovary through a canal with an opening in the centre of the stigma. (C) The canal was densely covered with papillae tissue. (D) Unilocular ovary consisted of thousands of ovules in parietal placentation.
Table 3.
Style length, style diameter, stigma lobe number and length, and the distance between stigma and anther of Hylocereus polyrhizus flowers
Style length (cm ± SD) |
Style diameter (cm ± SD) | Stigma lobe number | Stigma lobe length (cm ± SD) |
Distance between stigma and anther (cm ± SD) |
27 ± 2.54 | 0.63 ± 0.07 | 24 ± 2 | 1.65 ± 0.11 | 1.66 ± 0.53 |
The pericarpel of the flower is covered with bracteoles (modified leaves) arranged in phyllotactic spirals that subsequently become the peel of the developing fruit. The ovary is epigynous with the perianth positioned above the receptacle (Fig. 3A). The unilocular ovary is filled with thousands of small, white, unfertilized ovules. Each ovule is borne on a funiculus attached to the inner ovary wall (parietal placentation) (Fig. 3D). The number of carpels in the Cactaceae is difficult to determine due to the fusion of the pericarpel with the ovary. Nonetheless, the average number of stigmas determined in H. polyrhizus flower is 24 ± 2 (Table 3).
Blooming Sequence and Stigma Morphology at Different Anthesis Durations
At 1900 h, the flower of H. polyrhizus remained closed with its stigma slightly protruding from the tip of the flower (Fig. 4A). Upon closer observation, the stigma with its multiple lobes remained clasped, resembling a small closed flower (Fig. 4B). In the later evening, at 9:30 pm, the flowers started to bloom gradually and achieved full bloom in an upright position at 2300 h (Fig. 4C). During this period, the multiple stigma lobes unclasped, resembling a small flower, and were ready to receive pollen grains (Fig. 4D). By 0900 h, the following morning, flowers remained in full bloom and bees were visiting the flowers (Fig. 4E). By midday (1200 h), the flowers were fully closed and deposited pollen grains could be observed on the surface of the stigma lobes (Fig. 4F).
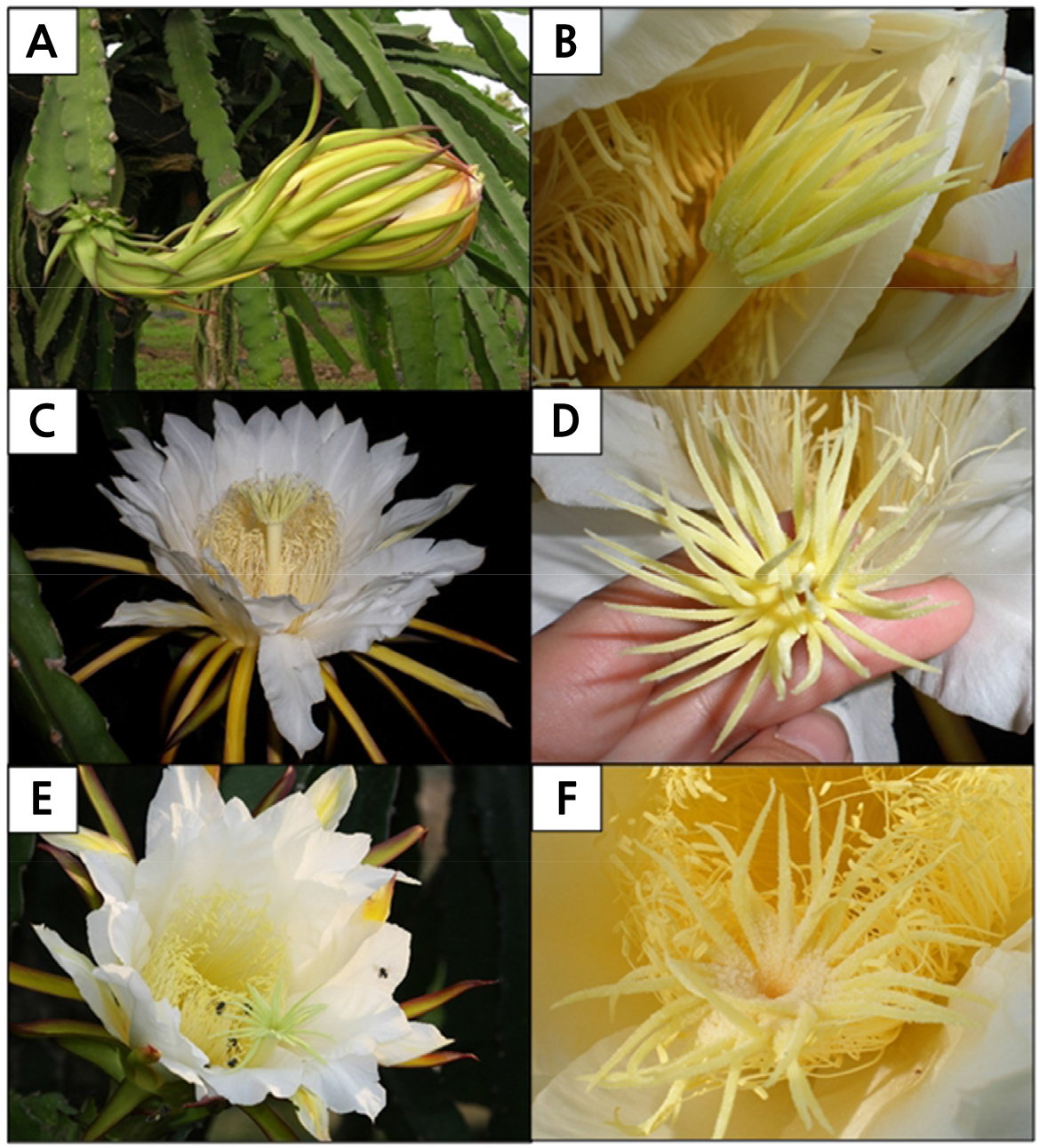
Fig. 4.
The blooming sequence of Hylocereus polyrhizus flowers. (A) At 1900 h, the flower remained closed with stigma slightly protruding from the tip of the flower (red arrow). (B) At 1900 h, the multiple stigma lobes remained clasped, mimicking a small, unopened flower. (C) At 2130 h, the flower bloomed in an upright position. (D) At 2300 h, the multiple stigma lobes unclasped and were ready to receive pollen grains. (E) At 0900 h (the following morning), flowers remained open with bees busy collecting pollen grains. (F) At 1200 h (the following midday), stigma lobes of flowers remained open, although flowers were fully closed. Deposited pollen grains (red arrow) were seen on the stigma.
During anthesis at 2200 h, the multiple stigma lobes of H. polyrhizus were located along the rim of the tubular style, forming an inverted conical cup shape (Fig. 5A). This unique form is of special importance to pollination efficiency as it is a means of preventing freshly deposited pollen grains from falling off the stigma. Besides, the numerous stigma lobes create a relatively large area that increases the probability of pollen grain deposition. When viewed with a stereomicroscope, pollen grains were found to be attached at the end of the papillae surface of one of the stigma lobes (Fig. 5B). A scanning electron microscopy (SEM) examination showed that each stigma lobe was densely covered with elongated, unicellular club-shaped papillae (Fig. 5C). These numerous papillae on the stigma surface provide a receptive surface for compatible pollen grains to adhere, hydrate, germinate, and grow.
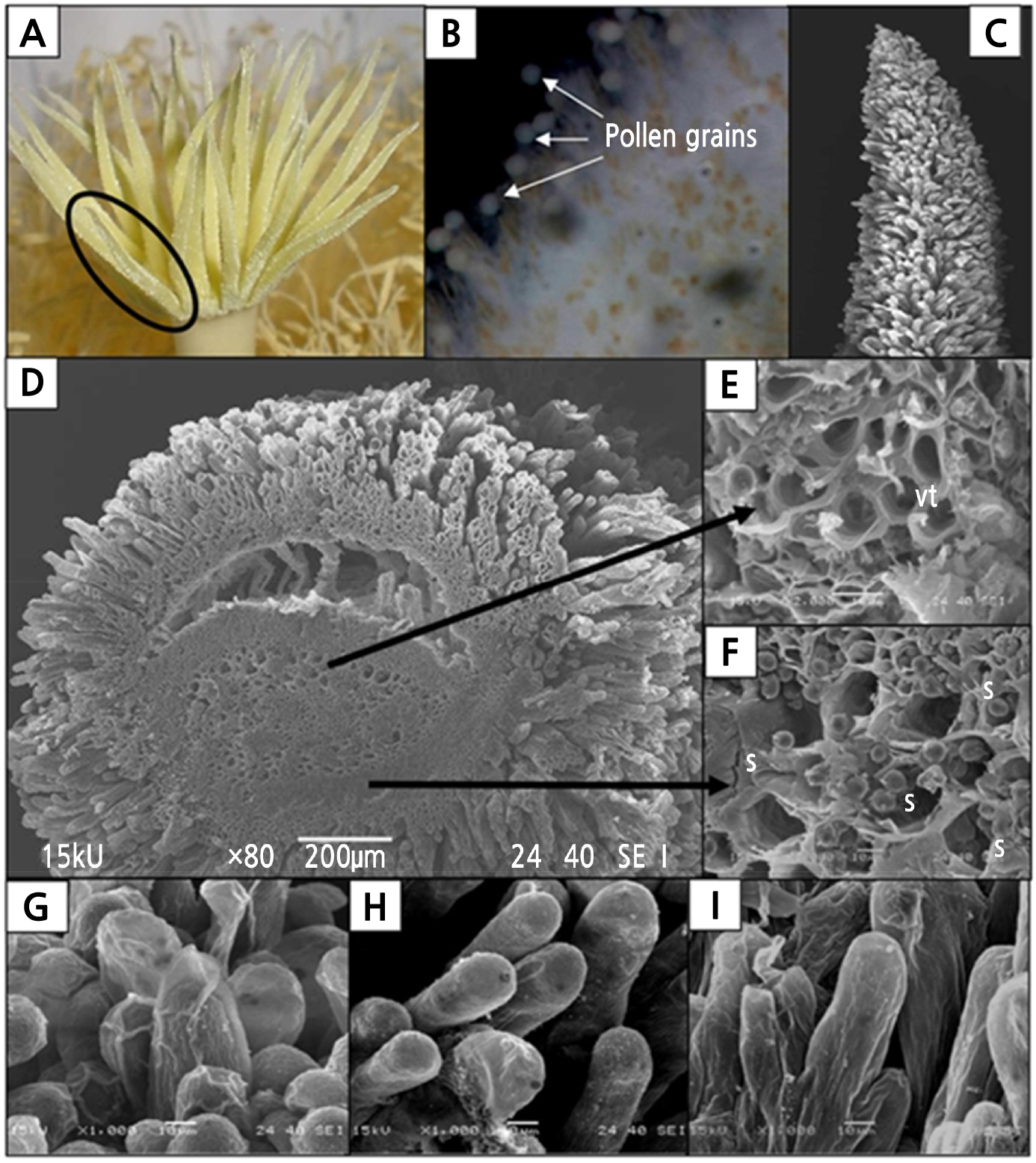
Fig. 5.
The stigmas of Hylocereus polyrhizus. (A) Multiple stigma lobes at the top of the style, which formed a cup-like feature during anthesis at 2200 h. (B) Pollen grains attached on the papillae surface during anthesis as viewed using a stereomicroscope. (C) The stigma lobe is fully covered with papillae structures at anthesis (200 µm, 80x). (D) Transverse view of a stigma lobe (200 µm, 80x). (E) Vascular tissues (vt) were found in the centre of the stigma lobe (10 µm, 1000x). (F) Starch granules (s) were found occupying parenchymatous cells (10 µm, 2000x). (G-H) Papillae on the stigma surface. (G) At 1900 h, some of the papillae were sheathed with a thin layer of pellicle structure (10 µm, 1000x). (H) At 2200 h, the pellicle sheath was absent and most of the papillae structures were turgid and erect, indicating readiness to receive pollen grains (10 µm, 1000x). (I) At 0900 h (the following morning), most of the papillae tissue still remained turgid (10 µm, 1000x).
A transverse view of the stigma lobe (Fig. 5D) revealed the presence of vascular bundles (Fig. 5E) and starch granules in the cells (Fig. 5F). At pre-anthesis (1900 h), the papillae were covered by a thin layer of pellicle (Fig. 5G) that had become less prominent by 2200 h during anthesis (Fig. 5H). The papillae tissue became more turgid, indicating its readiness to receive pollen grains at 2200 h. At 0900 h the following morning or 1 DAP, most of the papillae tissue still remained turgid (Fig. 5I).
At 2 DAP, the flowers became flaccid and wilted (Fig. 6A). The stigma started to shrink, and a few stigma lobes became brown (Fig. 6B). At 3 DAP, both the sepaloid tepal and the upper part of the petaloid tepals became more wilted (Fig. 6C) with more stigma lobes shrinking and browning (Fig. 6D) compared with previous days. At 4 DAP, the flower was completely wilted, and fungi began to colonize the outer part of the flower (Fig. 6E). At this stage, white mycelium was growing on the stigma surface, while the browning of the stigma lobes was more intense, especially on the style closest to the stigma (Fig. 6F). The pericarpel that contained the young fruit continued to develop as the days passed.
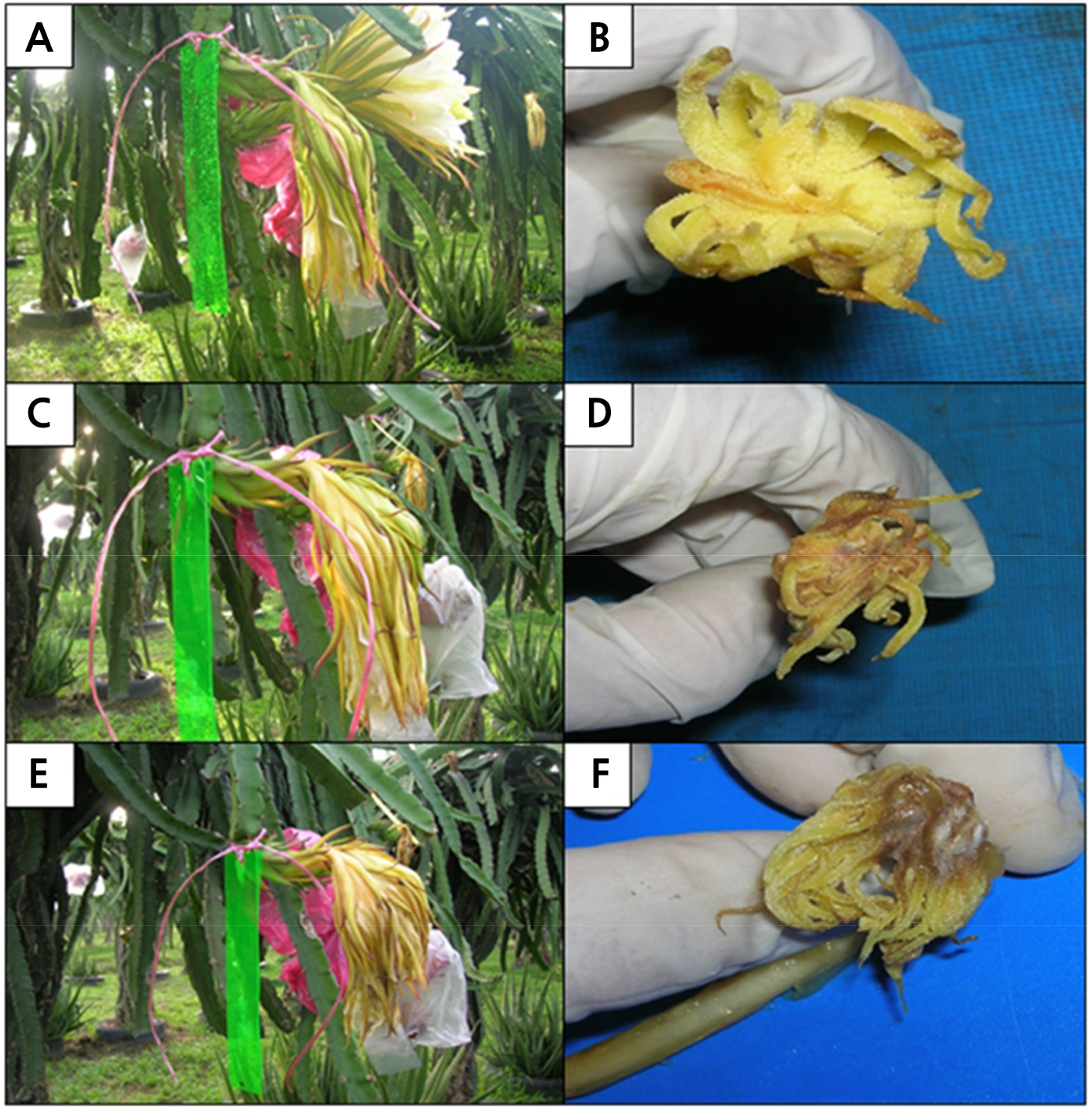
Fig. 6.
The blooming sequence of Hylocereus polyrhizus flowers at (A) 2, (C) 3, and (E) 4 days after pollination (DAP) with corresponding respective stigmas (B, D and F). (A) At 2 DAP, the flowers became flaccid and wilted, while (B) some of the stigma lobes had started to shrink and turned brown. (C) At 3 DAP, the flowers wilted, while (D) stigmas continued to shrink and more browning occurred. (E) At 4 DAP, the flower had completely wilted, and fungi mycelia were found growing on the outer part of the flower and (F) stigma surface. The browning of stigma lobes was more intense especially at styles closest to the stigma.
The duration of the anthesis indicated that the effective pollination period of H. polyrhizus did not exceed 12 h as the stigma collapsed as the anthesis progressed. Observation of the flowers at 1900, 2200, 0000, and 0900 (following morning) revealed a lack of exudates on the stigma surface. Hence, the stigma of H. polyrhizus was considered to be dry-type.
Pollen Morphology and Pollination Biology
Pollen grains of H. polyrhizus were released at anthesis as monads in mass quantity. The pollen grains are large, oblate spheroidal with a polar axis measuring 78.19 µm (Table 4) and an equatorial diameter of 80.65 µm (Fig. 7A). The polar:equatorial ratio of the grain is 0.97. Pollen grains viewed by SEM appeared trizonocolpate (Fig. 7B). The exine surface was covered with many sharply pointed radially directed rods called echinates (Fig. 7C and 7D). Three apertures of colpi arranged equidistantly around the axis of a pollen grain (Fig. 7E), which served as germination furrows for the emerging pollen tube during fertilization.
Table 4.
Pollen size and polar: equatorial ratio of Hylocereus polyrhizus flower
Pollen size (mm ± SD) | Polar: equatorial ratio | |
Polar axis | Equatorial diameter | |
78.19 ± 4.78 | 80.65 ± 3.84 | 0.97 |
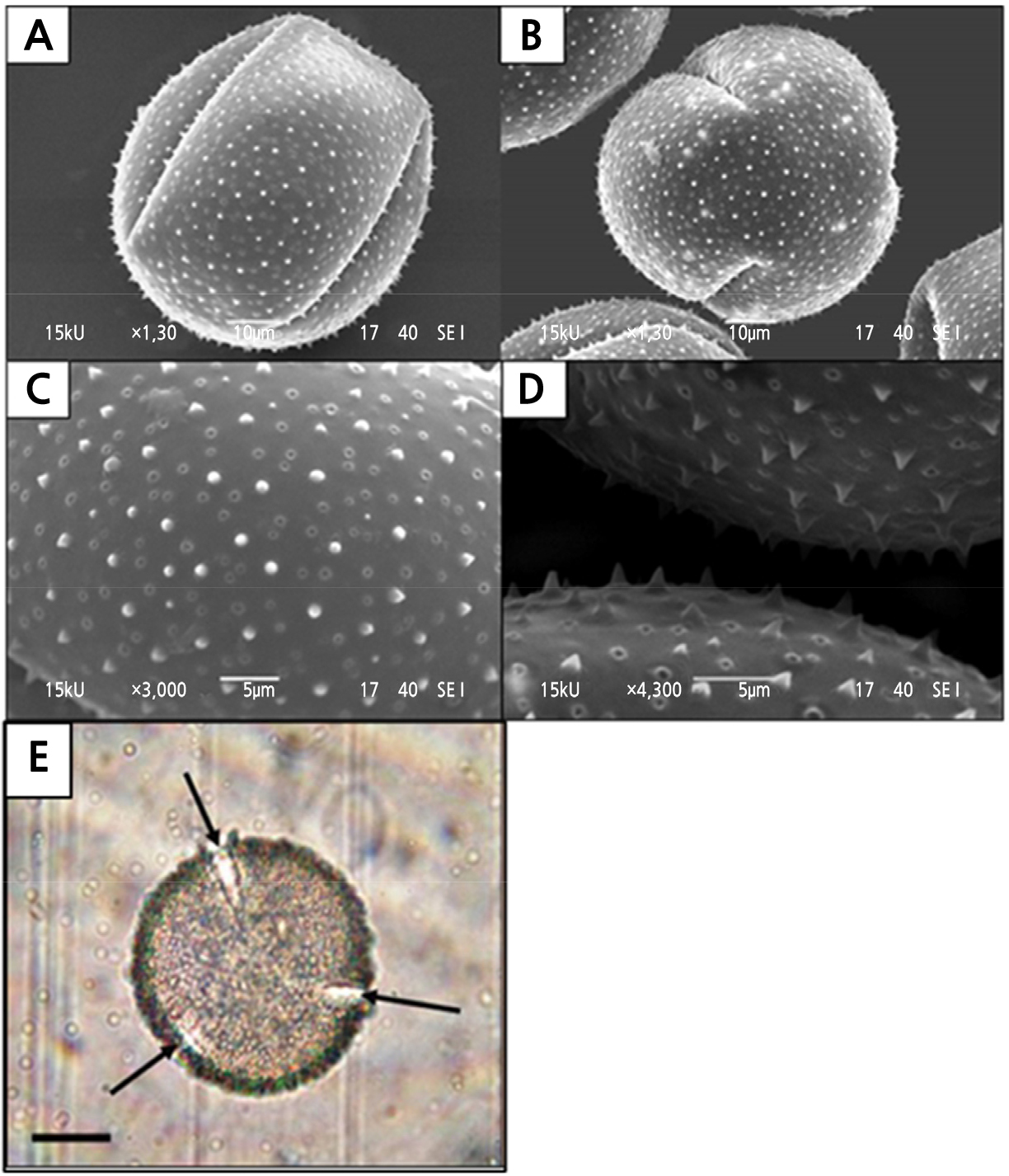
Fig. 7.
Features of the Hylocereus polyrhizus pollen grains viewed under the scanning electron (SEM) and light microscopes (LM). (A) SEM micrograph of equatorial view of pollen (10 µm, 1300x). (B) A pollen grain showing the trizonocolpate feature (10 µm, 1300x). (C) Echinate ornamentation on the exine surface (5 µm, 3000x). (D) Side view of the echinates on the exine surface (5 µm, 4300x). (E) Arrows indicated the three colpi that were arranged equidistantly around the axis (20 µm, 40x) as viewed under an LM.
After 2 h of pollination (i.e. 0 DAP), most of the pollen grains were hydrated and appeared spherical when in contact with the receptive stigma lobe as observed under by SEM (Fig. 8A). The emergence of a pollen tube was observed from one of the colpi closest to the stigma surface, indicating the germination of pollen grains (Fig. 8B). At 1 DAP, most of the pollen grains had been germinated with the pollen tube (Fig. 8C). However, a small number of pollen grains had dehydrated and collapsed, suggesting infertile pollen grains or incompatibility. The germinated pollen grains penetrated the papillae toward the transmitting tract in the style (Fig. 8D). By 2 DAP, both pollen grains and papillae structure were dehydrated and collapsed (Fig. 8E). Fungi mycelia could be found on the collapsed pollen grains and papillae by 2 DAP (Fig. 8F). The release of ethylene from the senescent organs and the high relative humidity in the open field could have promoted the establishment of fungi growth.
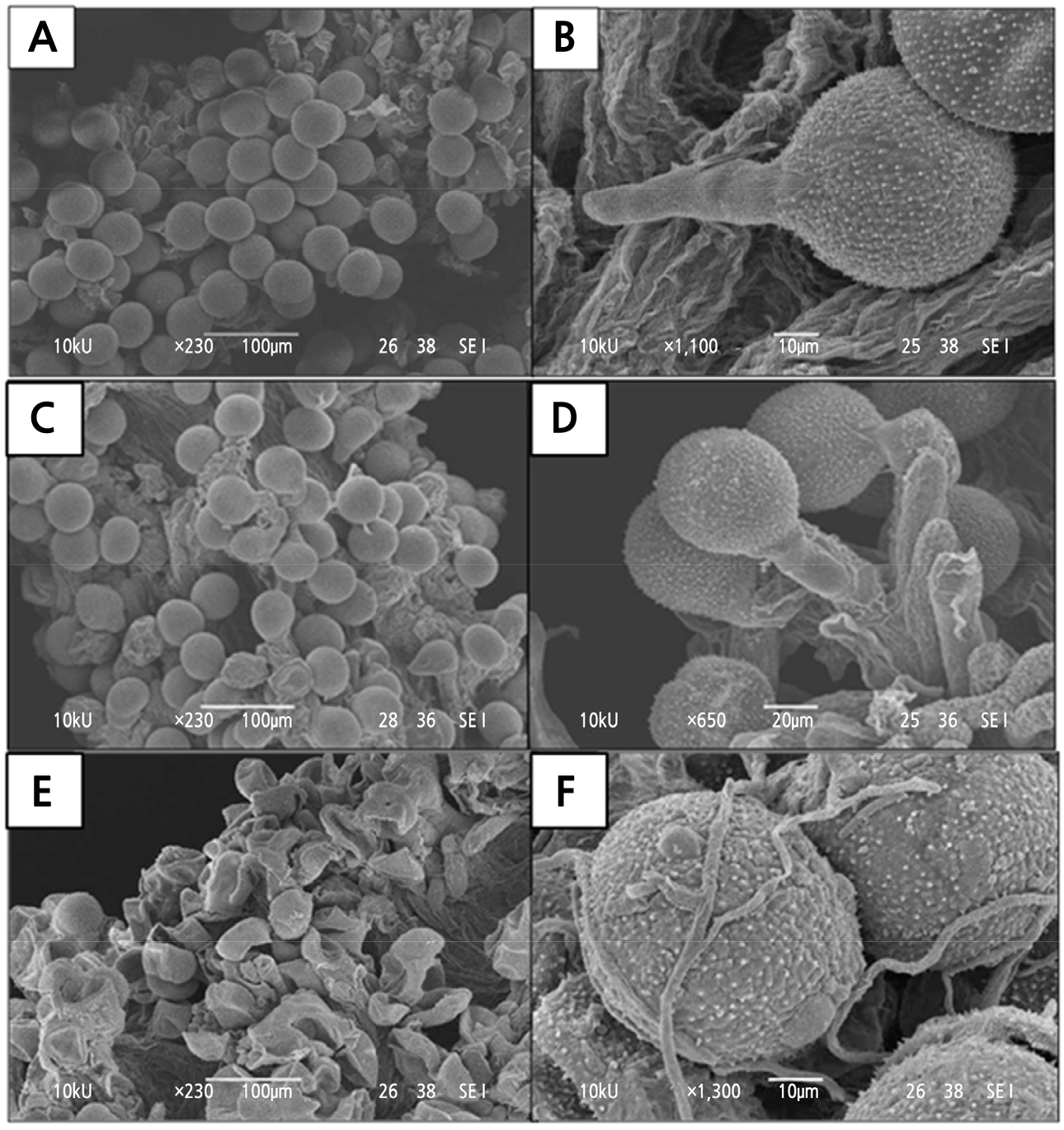
Fig. 8.
Pollen-stigma interactions viewed under the scanning electron microscope at various days after pollination (DAP): (A-B) 0 DAP, (C-D) 1 DAP, and (E-F) 2 DAP. (A) Two hours after pollination, hydrated pollen grains were spherical (100 µm, 230x). (B) A germinating pollen grain (10 µm, 1100x). (C) At 1 DAP, most of the pollen grains had germinated. However, there were some dehydrated and collapsed pollen grains (arrows) (100 µm, 230x). (D) Germinating pollen grain growing down the papillae tissue can be seen at 1 DAP (20 µm, 650x). (E) At 2 DAP, most of the pollen grains and stigmatic tissue collapsed, while mycelium can be found growing on pollen grains and papillae (arrows) (100 µm, 230x). (F) Under high magnification, mycelium can be found growing among the pollen grains (10 µm, 1300x).
When observed by fluorescence microscopy, 2 h after pollination, the pollen tubes appeared to be elongated underneath the papillae tissues (Fig. 9A). No traces of pollen tubes were detected in the middle part of the style (10 cm below the stigma) or the ovary (Fig. 9B). In the ovary, the ovules yielded a faint fluorescent light, especially in the funiculus thread region (Fig. 9C). After 2 DAP, most of the germinating pollen tubes were seen growing underneath the papillae tissue toward the style (Fig. 9D). The callose plugs of the pollen tubes were detected in the transmitting tract, which reached the middle part of the style (Fig. 9E). A few pollen tubes had reached the style end, but none were found to have entered the ovary cavity at this stage (Fig. 9F). At 4 DAP, pollen tubes were found germinating at the stigma surface and still growing underneath the papillae towards the style (Fig. 9G). Compatible pollen developed tubes in a dense cluster and grew rapidly down the middle style (Fig. 9H) and entered the ovary cavity (Fig. 9I). Compared to 2 DAP, the fluorescence of the callose plugs was less bright at 4 DAP. At this stage, numerous ovules had been fertilized by pollen tubes. With the help of the fluorescing callose plugs of the growing pollen tubes, transmitting tissue was found in the centre of the style, sandwiched between the canal and the parenchyma tissue, forming a ring when viewed in transverse section (Fig. 9J).
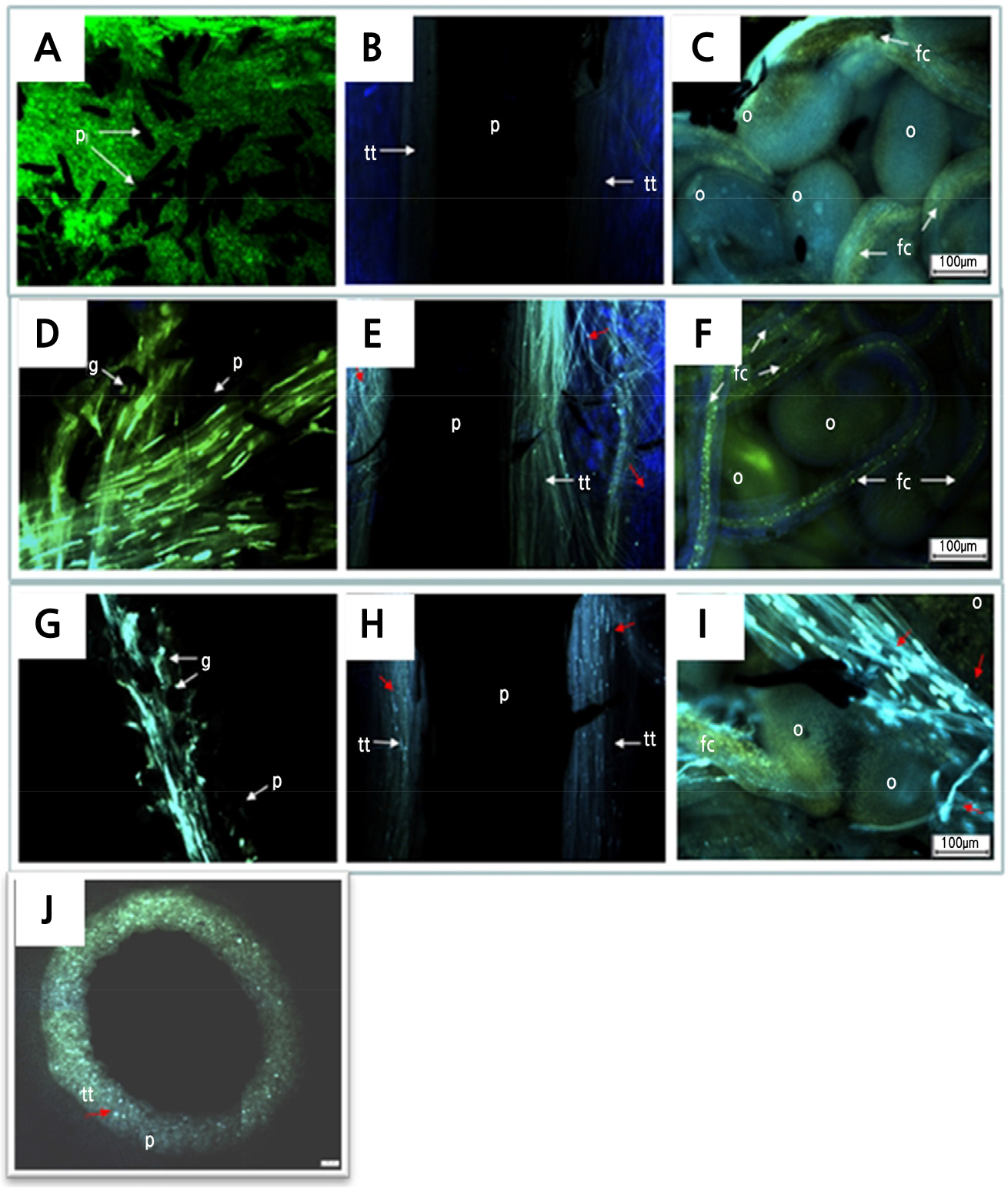
Fig. 9.
Pollen tube growth of Hylocereus polyrhizus at various days after pollination (DAP) examined under a fluorescence microscope. (A-C) 0 DAP. (A) Pollen grains with germinating tubes after 2 h of pollination (100 µm, 4x). (B) Pollen tube growth was absent in transmitting tissue in the middle part of the style (10 cm below stigma) (100 µm, 4x). (C) Pollen tubes were not detected in the ovary (100 µm, 4x). (D-F) 2 DAP. (D) Pollen tubes growing underneath papillae towards the style (100 µm, 4x). (E) Abundant pollen tubes were growing down the style (100 µm, 4x). (F) No pollen tube was detected in the ovary (100 µm, 4x). (G-I) 4 DAP. (G) Pollen tubes were found to be growing underneath papillae towards the style (100 µm, 4x). (H) Pollen tubes were found growing abundantly down the style at 4 DAP. Compared to 2 DAP, the fluorescence properties of callose plugs were less bright (100 µm, 4x). (I) At 4 DAP, pollen tubes were detected in the ovary and some were found growing towards ovules (100 µm, 4x). (J) Transverse section at the middle part of the style at 4 DAP shows a ring that formed from fluorescing callose plugs of growing pollen tubes. Fig. abbreviations: p, papillae; g, pollen grain; tt, transmitting tissue; fc, funiculus; o, ovule. Red arrows indicated pollen tube with callose plugs.
The transverse section of a light microscope (LM) micrograph shows the ground tissue of H. polyrhizus style was made up mostly of parenchymatous cells (Fig. 10A) and interspersed with vascular bundles (Fig. 10B). At 0 DAP, mucilage tracts (Fig. 10A) and starch granules (Fig. 10C) were also seen in the adjacent cells of transmitting tissue, possibly as a food resource for the growing pollen tubes within the style. The transmitting tissues were made up of small and compact cells with a thin wall that formed a distinct layer within the style (Fig. 10D). Phenolic compounds were also found within papillae structures (Fig. 10E).
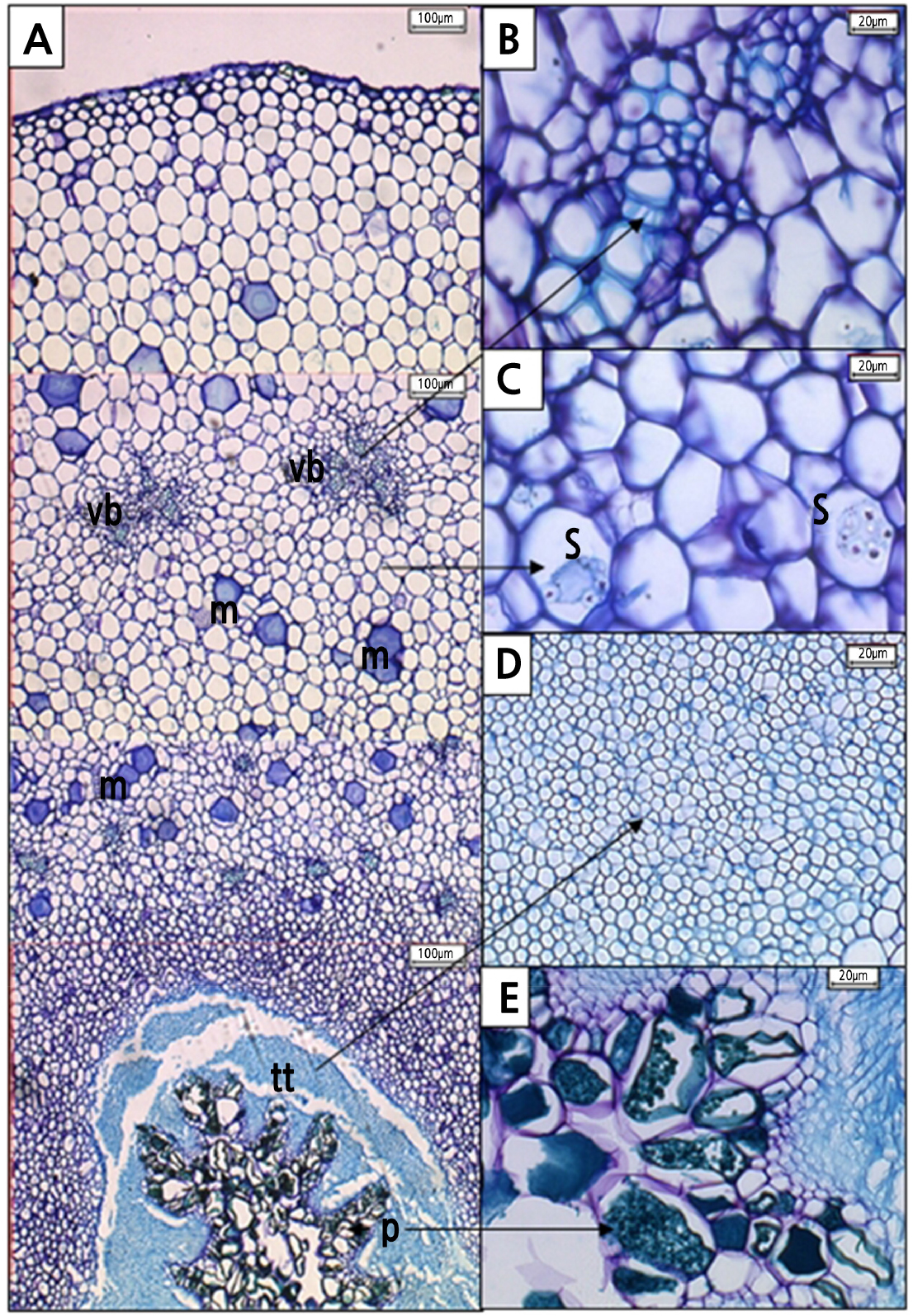
Fig. 10.
Transverse section of Hylocereus polyrhizus styles at 0 DAP as seen under a light microscope. Interspersed among parenchymatous cells of style were (A) mucilage (m) tracts (100 µm, 4x), (B) vascular bundle (vb) (20 µm, 40x), and (C) starch granules (s) (10 µm, 60x). (D) In the style canal, transmitting tracts (tt) (20 µm, 40x) coexist with (E) phenolic compounds (pc) that present in papillae (p) (20 µm, 40x).
As DAP progressed to the fourth day, parenchyma cells within the style became flaccid and less turgid (Fig. 11A). The collapsed vascular bundle (Fig. 11B), dried mucilage tracts (Fig. 11C), and flaccid transmitting tissue (Fig. 11D) were observed. At this stage, the phenolic compounds within the papillae structure were not found anymore (Fig. 11E). Starch granules were rarely found in the parenchyma cells. Most starch granules were hydrolysed and used as a food resource for growing pollen tubes as DAP advanced.
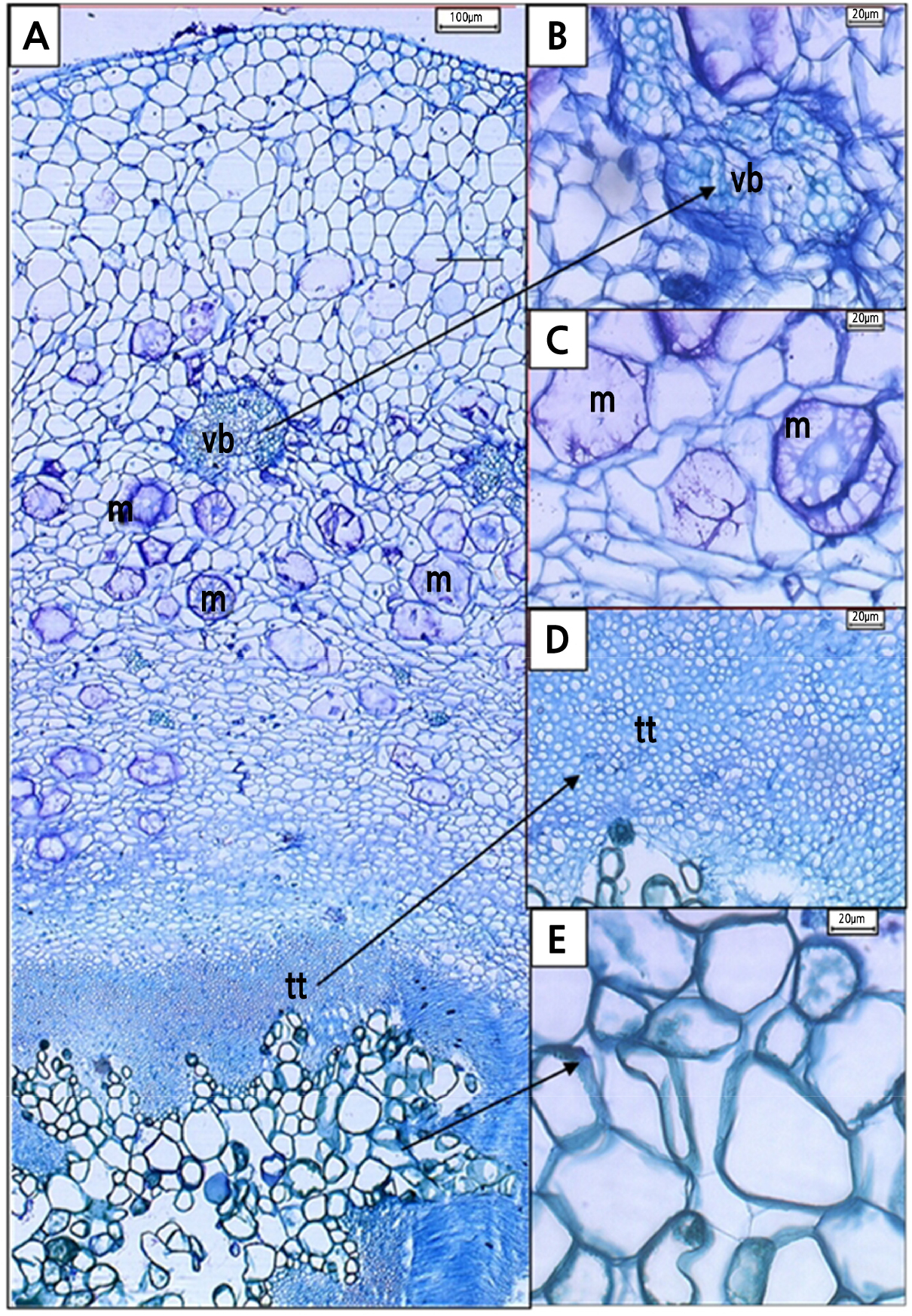
Fig. 11.
Transverse section of Hylocereus polyrhizus styles at 4 DAP as seen under a light microscope. (A) Parenchymatous cells became flaccid and less turgid (100 µm, 4x), while (B) vascular bundles (vb) collapsed (20 µm, 40x) with dried up (C) mucilage (m) and an absence of starch granules (20 µm, 40x). (D) Although the transmitting tracts (tt) were still in good shape (20 µm, 40x), (E) phenolic compounds were not detected in papillae (20 µm, 40x).
Discussion
The nocturnal flower of H. polyrhizus is characterized by the typical white-coloured sepaloid tepals type that facilitates the visibility of the flower to night pollinators by providing maximum light reflection. The flowers also emit a strong musky scent during anthesis at night that entices night pollinators such as bats and hawkmoths that are the natural pollinators of H. polyrhizus (Weiss et al., 1994). Bees were also spotted visiting the flowers during diurnal blooming, but most of the pollen grains had already dehisced (unpublished data). The extension of floral anthesis to the following day in H. polyrhizus indicates mixed pollination syndromes that increase the probability of visits by new guilds of pollinators (Walter, 2010). A similar flowering trait is also reported in the columnar cactus species of Echinopsis terscheckii to ensure sexual reproduction when nocturnal pollinators vary spatially and temporally (Ortega-Baes et al., 2011).
Self-incompatibility of H. polyrhizus is associated with approach herkogamy as demonstrated by the spatial difference and positioning of the stigma above the anthers. The numerous stigma lobes radiating from the terminal rim of the tubular style provide a suitable landing spot, while the vector’s body transports pollen grains from previously visited flowers, ensuring pollen deposition onto the stigma upon contact. This pre-pollination mechanism is a plant trait common in Cactaceae to prevent selfing, and it promotes outcrossing by favouring xenogamous pollen (Almeida et al., 2013; Valverde et al., 2015). Weiss et al. (1994) previously demonstrated the self-unfruitfulness in H. polyrhizus grown in a controlled environment and confirmed that cross-pollination with other clones resulted in an absolute (100%) with significantly larger fruit size.
The production of many-seeded fruits in flowering cacti such as H. polyrhizus and the tall Chilean columnar cactus (Echinopsis chiloensis) is the result of deposition of a large amount of pollen grains on the stigma (Pimienta-Barrios and del Castillo, 2002; Walter, 2010). In H. polyrhizus, the probability of substantial pollen deposition is enhanced by the large stigma area created by the numerous stigma lobes. As pollen grains land on the stigma, subsequent adhesion is facilitated by the highly ornamented, echinate sculpture on the pollen exine of H. polyrhizus.
In the present study, the lack of exudates observed on the stigma surface covered in papillae tissue identifies the stigma of H. polyrhizus as a dry-type. Unlike wet stigma, which is covered with surface cells that lyse to secrete sticky exudates containing protein, lipids, polysaccharides, and pigments, dry stigma has intact surface cells that typically protrude as papillae (Edlund et al., 2004). The thin pellicle layer covering the papillae consists of hydrated, proteinaceous, extra-cuticular material that poses a significant obstacle to pollen tube penetration (Mandujano et al., 2010; Allen et al., 2011). Both the pollen and pellicle properties play crucial roles in pollen adhesion and hydration, especially in dry-type stigmas that serve as the point of pollen capture (Prychid et al., 2011; Swanson et al., 2016). Interaction between dry stigma and pollen grains is highly regulated especially in self-incompatible species like Arabidopsis thaliana and Brassica oleracea (Edlund et al., 2004; Zheng et al., 2018).
Pollen germination in H. polyrhizus occurred approximately 2 h after pollination. Upon germination on the stigma surface, the pollen tube elongated underneath the papillae tissues, marking the beginning of a successful journey. Upon penetrating the papillae tissues, the pollen tube headed downwards through the transmitting tissues and subsequently grew along with the style. This growth was facilitated by an extracellular matrix composed of starch granules, as observed in the adjacent cells of the transmitting tissues; by mucilage tracts on the parenchymatous cells of the style; and by phenolic compounds on the papillae (Williams, 2012; Pereira et al., 2014).
Pollen tube growth is biphasic. In the first autotrophic phase, pollen tube growth on the stigma depended on the reserves contained in pollen grains because dry-type stigmas contained no resources (Cruden, 2009). As the energy reserves in the pollen grain were expended, pollen tube growth became heterotrophic, using resources from the style reserves. The presence of callose plugs in the transmitting tract in the middle style at 2 DAP indicated the transition from autotrophic to heterotrophic tube growth (Stephenson et al., 2003).
The long style of H. polyrhizus (27 cm) belongs to the solid type as most of the pollen tubes grew to the ovary within the transmitting tissue. Development of compatible pollen tubes in a dense cluster suggests that the style of H. polyrhizus is capable of supporting the growth of a large number of pollen tubes. Even though the flower started to wilt at 2 DAP due to fungal invasion on the stigma and upper style region, most of the growing pollen tubes had successfully reached the middle style region (10 cm below the stigma) by that time. The pollen tubes reached the ovary cavity at 4 DAP and fertilized the ovules leading to eventual fruit set. The parenchyma cells of the style became flaccid, the vascular bundles collapsed, and mucilage tracts dried up, indicating exhaustion of the resources utilized during pollen tube growth.
In summary, floral traits of H. polyrhizus are associated with chiropterophily pollination with bats as the main pollinators that seek pollen grains and nectar as food sources. However, natural pollination by bats at night and by bees the next morning was not feasible because this would not guarantee high fruit production. A pre-pollination mechanism of herkogamy exhibited by the spatial positioning of the stigma above the anthers in H. polyrhizus further substantiates the significance of xenogamy. It also means that growers must carry out manual pollination at night to ensure efficient and successful fertilization; however, this manual labour is tedious and the cost may be a major concern to orchard owners. Therefore, assessing the pollination efficiency of H. polyrhizus under natural settings in open field experiments seems imperative to determine the rate of fruit set, especially for the commercial production of H. polyrhizus. The findings of the present study implicated the dry-type stigma as a post-pollination mechanism associated with self-incompatibility of the plant; however, further investigation is warranted. This study provided insights into the pollination process in H. polyrhizus that will be useful for obtaining maximum fruit production under field conditions.