Introduction
Materials and Methods
Plant Material and Sample Preparation
Determination of the Total Phenolic Content (TPC)
Determination of the Total Flavonoid Content (TFC)
Determination of the Radical Scavenging Activity
Cell Culture
Cell Viability Assay
Determination of Intracellular ROS
High-Performance Liquid Chromatography (HPLC) Analysis of Phenolic Acids and Flavonoids
Statistical Analysis
Results and Discussion
Seasonal Variations Influence the Total Phenolic and Flavonoid Contents of SQW.
Seasonal Variations Influence the Radical Scavenging Activities of SQW.
The Harvest Time of SQ Affects the Cellular ROS Inhibitory Ability of the SQW in PC12 Cells.
Seasonal Variations in the Phenolic Contents of SQW
Conclusion
Introduction
Sasa species, which are widespread throughout China, Korea, and Japan, are perennial dwarf bamboo plants. Bamboo grasses have been utilized in herbal medicine owing to their pharmacological effects, which include antidiabetic, anticancer, antioxidant, anti-melanogenic, hepatoprotective, and anti-inflammatory properties (Kang et al., 2012; Kim et al., 2015). Major active compounds in bamboo leaves include C-glycoside flavonoids such as vitexin, homovitexin, orientin, and isoorientin (Zhang et al., 2008). Phenolic acids including ferulic acid, caffeic acid, p-coumaric acid and chlorogenic acid have also been identified in bamboo leaves. These components have strong anti-aging, radical scavenging, antibacterial, and antiviral activities, which aid in the prevention of degenerative diseases (Ni et al., 2011). A hot water fraction and eight polysaccharides isolated from Sasa veitchii leaves significantly inhibited influenza A virus infection in the rat lung (Hayashi et al., 2014). In a recent study, Kurilensin A and B, two new compounds, and the well-known compounds tricin-4’-O-beta-d-glucopyranoside and tricin-5-O-b-D-glucosepyronoside, were separated from S. kurilensis leaves (Hasegawa et al., 2008). The DPPH radical scavenging capacity of both Kurilensin A and B was two-fold higher compared to ascorbic acid (Hasegawa et al., 2008).
Sasa quelpaertensis Nakai (SQ), also known as Jeju-Joritdae (Korean name), is an endemic species in Korea distributed on Jeju Island (Yoon et al., 2007). SQ leaves are commonly used as herbal teas. Hot water extracts have shown strong antioxidative and anti-inflammatory effects (Hwang et al., 2007). SQ leaves contain amino acids, polysaccharides, and polyphenols, such as tricin and p-coumaric acid. These phenolics are known for their anti-inflammatory, anticancer, and anti-obesity properties (Kim et al., 2015). A water extract of S. quelpaertensis Nakai leaves and p-coumaric acid can reduce oleic-acid-induced lipid accumulation in human hepatoma HepG2 cells by promoting AMPK phosphorylation (Kim et al., 2013). S. quelpaertensis Nakai leaves also exhibit anti-inflammatory effects by reducing the expression levels of inducible nitric oxide synthase (iNOS), cyclooxygenase-2 (COX-2), and tumor necrosis factor-α (TNF-α) (Kim et al., 2014).
Reactive oxygen species (ROS) such as hydroxyl radicals (∙OH), superoxide (O2∙−), and hydrogen peroxide (H2O2) are involved in major cellular responses, including signal transduction, gene transcription, and the immune response (Zuo et al., 2015). When ROS are generated in excess amounts, they cause oxidative stress, damaging DNA, RNA, proteins, and lipids (Cui et al., 2012). This damage can lead to cardiovascular, neurodegenerative, respiratory, and digestive diseases (Cui et al., 2012). Neuronal cells, which are attacked by oxidative stress, mostly die via apoptosis or necrosis. This is an important aspect of neurodegenerative diseases such as Alzheimer's disease, Parkinson’s disease, and Huntington's disease (Zhao and Zhao, 2013; Singh et al., 2019). Antioxidant defense systems in aerobic organisms can be classified as enzymatic or non-enzymatic antioxidants. Antioxidant enzymes including superoxide dismutase (SOD), glutathione peroxidase (GPx), glutathione reductase (GSR), and catalase (CAT) can stabilize or inactivate free radicals before they attack biological materials (Birben et al., 2012; Wu et al., 2013). Natural (non-enzymatic) antioxidants, such as vitamins E, A, and C as well as carotenoids, glutathione, minerals, and phenolic compounds, present in large amounts in vegetables, fruits, along with natural products have been used as therapeutic or preventive agents owing to their free radical scavenging abilities (Mirończuk-Chodakowska et al., 2018; Nimse and Pal, 2015).
The timing of the harvest influences the chemical composition and pharmacological effects of different plants (Dong et al., 2003; Coklar and Akbulut, 2021; Ghimire et al., 2021; Lee et al., 2021b). To determine the optimal harvesting season, it is necessary to investigate when bioactive compounds are produced and accumulate in high amounts.
Although there have been many studies of the health and industrial benefits of S. quelpaertensis, few studies of the relationship between the harvest time and the biological properties of its leaves have been conducted. Such studies are needed to determine the optimal harvest period, where the quality of the plant material depends on the content of phenolic compounds and is important for industrial applications (Modareskia et al., 2022). This study aimed to analyze seasonal variations in total flavonoid and phenolic contents and the radical scavenging activity and cellular ROS inhibitory ability of S. quelpaertensis leaves.
Materials and Methods
Plant Material and Sample Preparation
SQ leaves were harvested in spring (May), summer (August), fall (November), and winter (February) in Jeju, Jeju Special Self-Governing Province, South Korea, in 2021. The plant materials were taxonomically identified by Yun Ji Lee and voucher specimens (MPS006530-1, MPS006530-2, MPS006530-3, and MPS006530-4) were placed at the Korean Medicinal Resources Herbarium (KMRH) in Eumseong, South Korea. Leaves (1 kg) were initially crushed and sieved using a wire mesh sieve (aperture size, 1.40 mm). Next, the ground powder was refluxed with distilled water three times at room temperature for a period of at least 24 h (solvent:sample =10:1, v/v). All extracts [Sasa quelpaertensis Nakai leaf water extract (SQW)] were solubilized in DMSO or MeOH (Sigma, St. Louis, MO, USA).
Determination of the Total Phenolic Content (TPC)
TPC was estimated according to a previously described method using Folin–Ciocalteu’s reagent (Blainski et al., 2013; Cho et al., 2020; Lee et al., 2021a; Lee et al., 2022b). Each sample reacted with 200 µL of 2% sodium carbonate and 10 µL of Folin–Ciocalteu’s reagent for 30 min at RT. The value was measured at 750 nm with a plate reader (BioTek, Winooski, VT, USA).
Determination of the Total Flavonoid Content (TFC)
TFC was analyzed by the AlCl3 method with some variations (Akbay et al., 2003; Eum et al., 2020; Orlando et al., 2022). Various concentrations of a standard solution (quercetin; 31.25, 62.5, 125, 250, and 500 µg/mL) and four extracts were mixed with deionized water. Then, 5% sodium nitrite was added and the resulting solution was incubated for 5 min at RT, followed by the addition of 10% aluminum chloride and incubation for 6 min. Next, 1M sodium hydroxide was put into each reaction mixture. Finally, the absorbance was estimated at 510 nm using a plate reader (Bio-Tek).
Determination of the Radical Scavenging Activity
Radical scavenging activities were measured by means of an ABTS assay (Cao et al., 2022; Lee et al., 2022a; Van den Berg et al., 1999). The ABTS radical cation solution was made by mixing potassium persulfate (2.6 mM) and ABTS (7.4 mM) in deionized water, which was then reacted for 24 h at RT. Then, each sample was reacted with ABTS+ solution for 30 min at RT. Values were estimated with a plate reader at 735 nm. ABTS+ radical scavenging activity was estimated according to the following equation:
where Abscontrol is the absorbance of the ABTS radicals in water and Abssample is the absorbance of the ABTS radical solution mixed with the sample.
The extract reacted with the DPPH radical solution for 30 min at RT. The value of the mixture was gauged at 520 nm. The DPPH radical scavenging activity was estimated according to the following equation (Bitalebi et al., 2019):
Cell Culture
Pheochromocytoma cells (PC12) from rats were obtained from ATCC (Manassas, VA, USA) and cultured in DMEM (supplemented 10% FBS and 1% penicillin and streptomycin; Gibco, Grand Island, NY, USA) at 37°C in a humidified atmosphere containing 5% CO2. Adherent PC12 cells were passaged when they covered the surface of the culture vessel (70–80% confluent), with trypsin-EDTA solution used to detach the cells from the plate every 2-3 days.
Cell Viability Assay
The cytotoxicity of the SQW (February, May, August, and November) in PC12 cells was evaluated by means of a MTT [3-(4,5-dimethylthiazol-2-yl)-2,5-diphenyltetrazolium bromide] assay. PC12 cells were seeded at 1 × 105 cells/mL in a 96-well plate. After cultivation for 24 h, extracts (25–200 µg/mL) were added to the cells and incubated at 37°C for 24 h. Thereafter, MTT solution was added to each well and the plate was cultured for 1 h at 37°C. The media were eliminated and DMSO (100 µL) was added. After shaking, the value was measured at 540 nm with a plate reader.
Determination of Intracellular ROS
ROS levels in the PC12 cells were determined using a 2’,7’-dichlorohydrofluorescein diacetate (DCF-DA; Sigma-Aldrich) assay. PC12 cells were seeded in 96-well plates and cultured for 24 h. Next, the cells were treated with SQW (February, May, August, and November) at two different concentrations (25 and 50 µg/mL) and cultured at 37°C for 24 h. Then, the cells were exposed to 50 µM H2O2 for 30 min, followed by DCF-DA (20 µM) for 30 min.
High-Performance Liquid Chromatography (HPLC) Analysis of Phenolic Acids and Flavonoids
Standards consist of phenolic acids (vanillic acid, p-coumaric acid, gallic acid, ferulic acid, caffeic acid, homogentisic acid, protocatechuic acid, chlorogenic acid, veratric acid, ellagic acid, rosmarinic acid, and cinnamic acid) and flavonoids (isoorientin, tricin, kaempferol, luteolin, quercetin, apigenin, naringenin). Phenolic compounds in SQW were analyzed by HPLC using a UV-VIS detector (1200 series; Agilent Technologies, Santa Clara, CA, USA; column: XB-C18, 100 × 4.6 mm, 2.6 µm; Phenomenex, Torrance, CA, USA). The mobile phase was composed of water with 0.1% formic acid (solvent A) and acetonitrile with 0.1% formic acid (solvent B). The gradient program for the mobile phase was 0–3 min (7–7% B), 3–10 min (7–12% B), 10–42 min (12–22% B), 42–50 min (22–50% B), 50–60 min (50–100% B), 60–68 min (100–100% B), and 68–75 min (100–7% B). The detection wavelength, injection volume, and flow rate were set to 280 nm, 10 µL, and 1.0 mL/min, respectively. Standard solutions were analyzed at concentrations of 0.03125, 0.0625, 0.125, 0.25, and 0.5 mg/mL. A standard curve was generated to quantify the amounts of vanillic acid and isoorientin. In the linear regression equation (y = ax + b), x represents the concentration of vanillic acid and isoorientin (mg/mL), and y is the peak area.
Statistical Analysis
All data were analyzed using Excel 2016 (Microsoft Corp., Redmond, WA, USA) and the values are presented as the mean ± standard deviation (SD). To determine the significance levels set at p < 0.05, p < 0.01, and p < 0.001, Duncan’s test and one-way ANOVA were performed using R software (version 3.6.3; R Development Core Team, Vienna, Austria). A correlation analysis was conducted using GraphPad Prism software (GraphPad Software Inc., San Diego, CA, USA).
Results and Discussion
Seasonal Variations Influence the Total Phenolic and Flavonoid Contents of SQW.
S. quelpaertensis leaves change color and shape depending on the season. In spring, a curled sprout appears, while in the summer the leaves are stretched out. In autumn, the rim of the leaf has a yellowish band that broadens in winter (Ko et al., 2018). This study was performed to provide fundamental data to determine the most suitable harvesting time for S. quelpaertensis leaves to ensure high contents of bioactive compounds and high antioxidant activity. We hypothesized that the levels of active components and biological activities in S. quelpaertensis leaves would show seasonality.
The yields of SQWs in February (5.31%), May (9.41%), August (8.07%) and November (6.93%) were taken as the dry weight of the plant material. The TPC, TFC, and antioxidant activities of the four extracts prepared from plants harvested in February, May, August, and November are shown in Fig. 1A. The TPCs of the SQW were 222.73 ± 7.04 mg GAE/g extract (February), 298 ± 5.80 mg GAE/g extract (May), 228.88 ± 5.80 mg GAE/g extract (August), and 176.65 ± 7.41 mg GAE/g extract (November). The TFCs of SQW were determined to be 128.11 ± 30.82 mg QE/g extract (February), 240.81 ± 10.27 mg QE/g ex-tract (May), 86.60 ± 20.55 mg QE/g extract (August), and 74.73 ± 17.79 mg QE/g extract (November).
Seasonal Variations Influence the Radical Scavenging Activities of SQW.
The radical scavenging activities of SQW in the ABTS and DPPH assays showed seasonal variations among the extracts. The IC50 values of the ABTS assay were 2.02 ± 0.14 mg/mL (February), 1.62 ± 0.09 mg/mL (May), 1.85 ± 0.03 mg/mL (August), and 0.73 ± 0.06 mg/mL (November). The order of the IC50 values in the DPPH assay was 3.15 ± 0.06 mg/mL (February) > 2.93 ± 0.15 mg/mL (August) > 1.82 ± 0.08 mg/mL (May) > 1.47 ± 0.06 mg/mL (November), as shown in Fig. 1B. The IC50 values were the concentrations required to eliminate 50% of the free radicals. Therefore, a lower IC50 value indicates a higher antioxidant effect (Matuszewska et al., 2018). The SQW from May exhibited the highest TPC and TFC outcomes, while the SQW from November showed the highest antioxidant activity. The antioxidant property of the SQW from May was second highest.
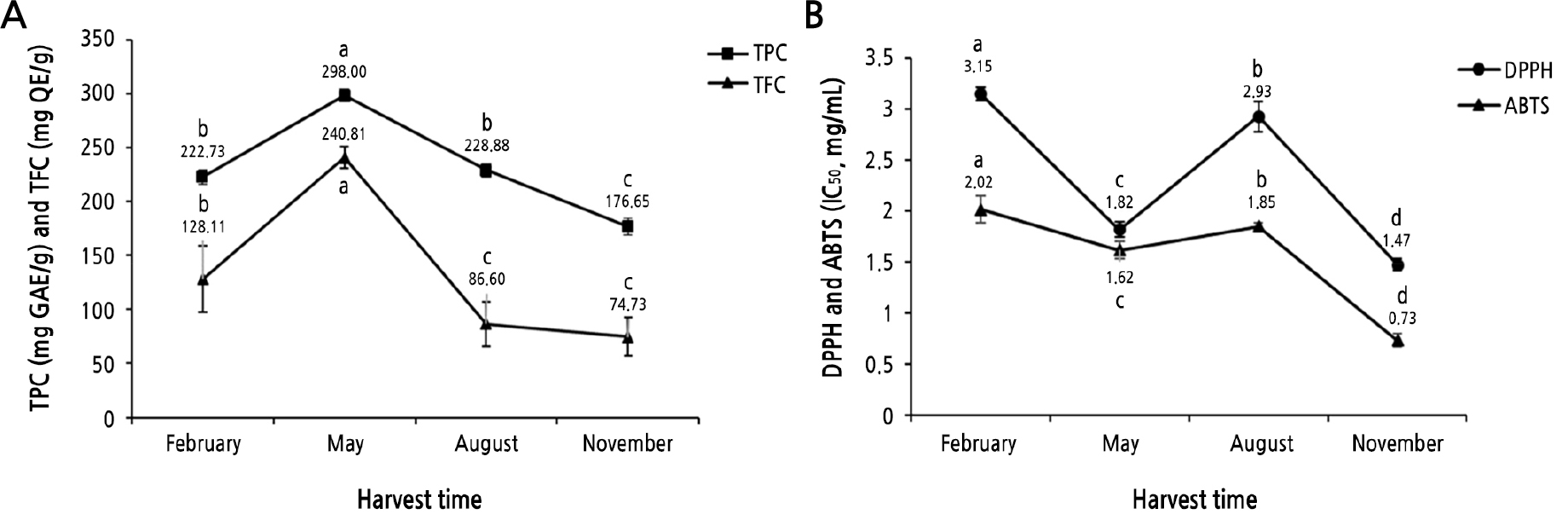
Fig. 1.
Variations of TPC, TFC, and the radical scavenging activities of SQW depending on the harvest time (February, May, August, and November). (A) TPC and TFC of the tested samples expressed as the gallic acid equivalent (GAE) and quercetin equivalent (QE), respectively, on a dry mass basis. (B) Antioxidative activities (DPPH and ABTS radical scavenging assay) of the tested samples expressed as IC50 values. Data are shown as the mean ± SD (n = 3). Lowercase letters represent significant differences at p < 0.05.
The Harvest Time of SQ Affects the Cellular ROS Inhibitory Ability of the SQW in PC12 Cells.
As shown in Fig. 2, SQW from the different seasons (February, May, August, and November) was not cytotoxic to PC12 cells up to a level of 50 µg/mL (Fig. 2). Thus, the following experiments were conducted using SQW concentrations of up to 50 µg/mL. We focused on changes in the cellular ROS inhibitory ability of the SQW depending on the harvest season. When cells were stimulated with 50 µM H2O2, a 1.37-fold increase in the production of ROS compared to the untreated control was observed. All extracts (February, May, August, and November) significantly inhibited ROS production in H2O2-stimulated PC12 neuronal cells in a concentration-dependent manner (Fig. 3A). SQW (February, May, August, and November) at 50 µg/mL decreased the intracellular ROS level 0.67-, 0.56-, 0.80-, and 0.78-fold, respectively. SQW in May decreased ROS production in PC12 neuronal cells most efficiently. These seasonal changes in the ROS scavenging activity were consistent with the variations in TPC and TFC, indicating that the cellular ROS inhibitory ability of the May SQW was influenced by diverse phenolic compounds.
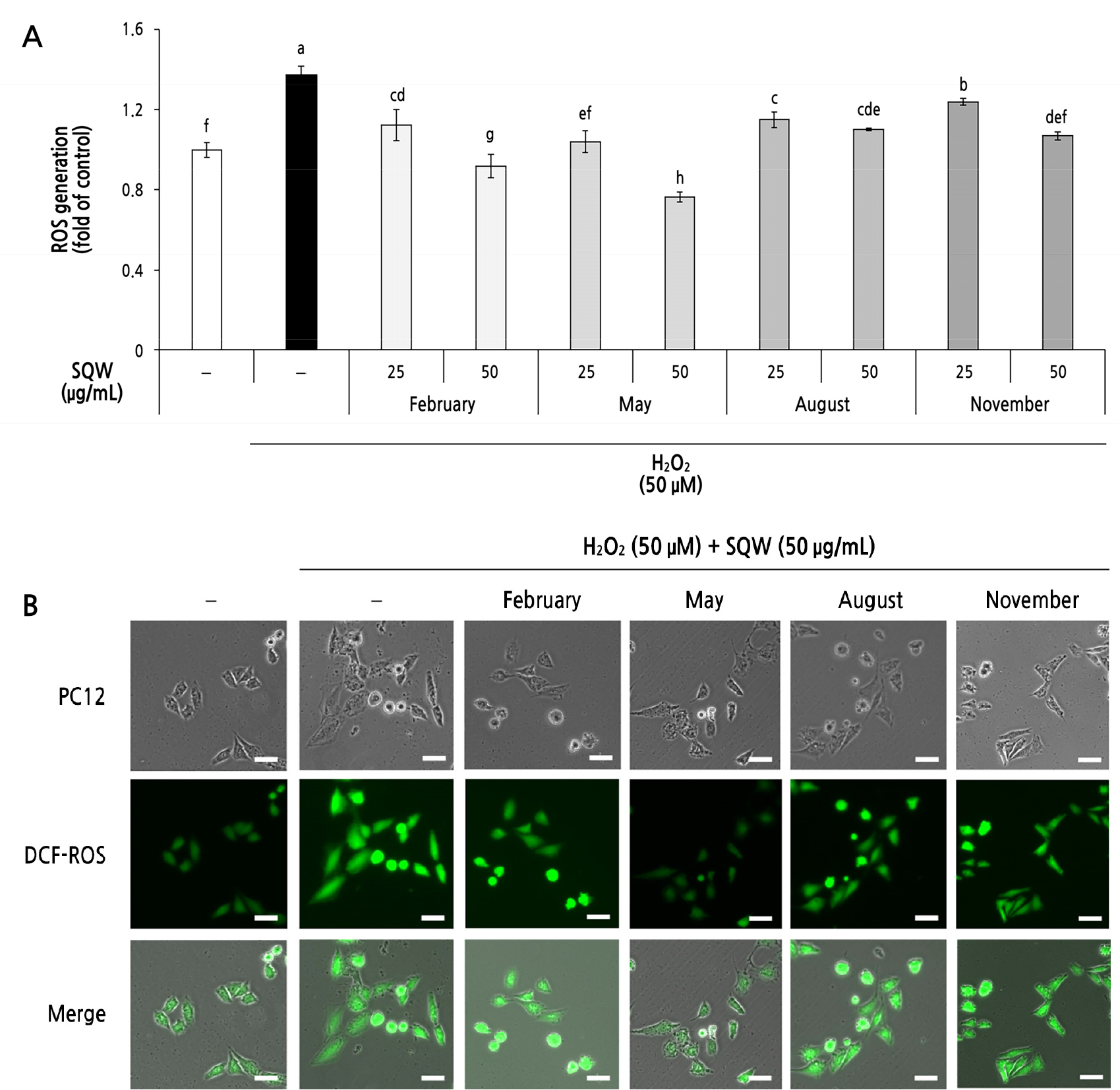
Fig. 3.
(A) SQW (25 and 50 μg/mL) prepared from plants harvested at four different times (February, May, August and November) reduce H2O2-induced ROS production in PC12. The negative control (–) indicates an untreated group. SQW, Sasa quelpaertensis water extract. Values represent the means ± SDs of triplicate measurements. Data indicated by the same letters are not significantly different (p < 0.05). (B) Cellular ROS levels were detected by fluorescence microscopy with DCF-DA. Scale bar, 5 μm.
We monitored changes of ROS in PC12 cells in response to a treatment with SQW (50 µg/mL) at the different harvest times (Fig. 3B). All SQW samples inhibited ROS production following a H2O2 treatment; the SQW from May was the most efficient. This result is consistent with the results of the quantitative analysis of the ROS levels (Fig. 3A).
Seasonal Variations in the Phenolic Contents of SQW
Phenolic compounds derived from secondary metabolism contain hydroxyl groups (Babbar et al., 2014). These can be classified into groups according to whether they contain stilbenes, lignans, flavonoids, or phenolic acids, and depending on their chemical structure (Cione et al., 2020). We analyzed 20 phenolic compounds in SQW and detected vanillic acid (a phenolic acid) and isoorientin (a flavonoid) in this study.
We conducted a HPLC analysis to determine how major compounds within the SQW vary by harvest period. The chromatographic data for individual phenolic compounds in the four different SQWs are shown in Figs. 4 and 5. The vanillic acid content was 2.10 ± 0.03 mg/g in May, 1.22 ± 0.14 mg/g in August, and 0.80 ± 0.00 mg/g in November. The isoorientin content was 1.19 ± 0.02 in February, 1.56 ± 0.12 in May, 1.19 ± 0.01 in August, and 0.85 ± 0.01 in November. The SQW in May had higher levels of vanillic acid and isoorientin compared to the other extracts (p < 0.05). The SQW from May showed the highest cellular ROS inhibitory ability in PC12 cells as well as the highest vanillic acid and isoorientin contents (2.10 ± 0.03 and 1.56 ± 0.12 mg/g, respectively).
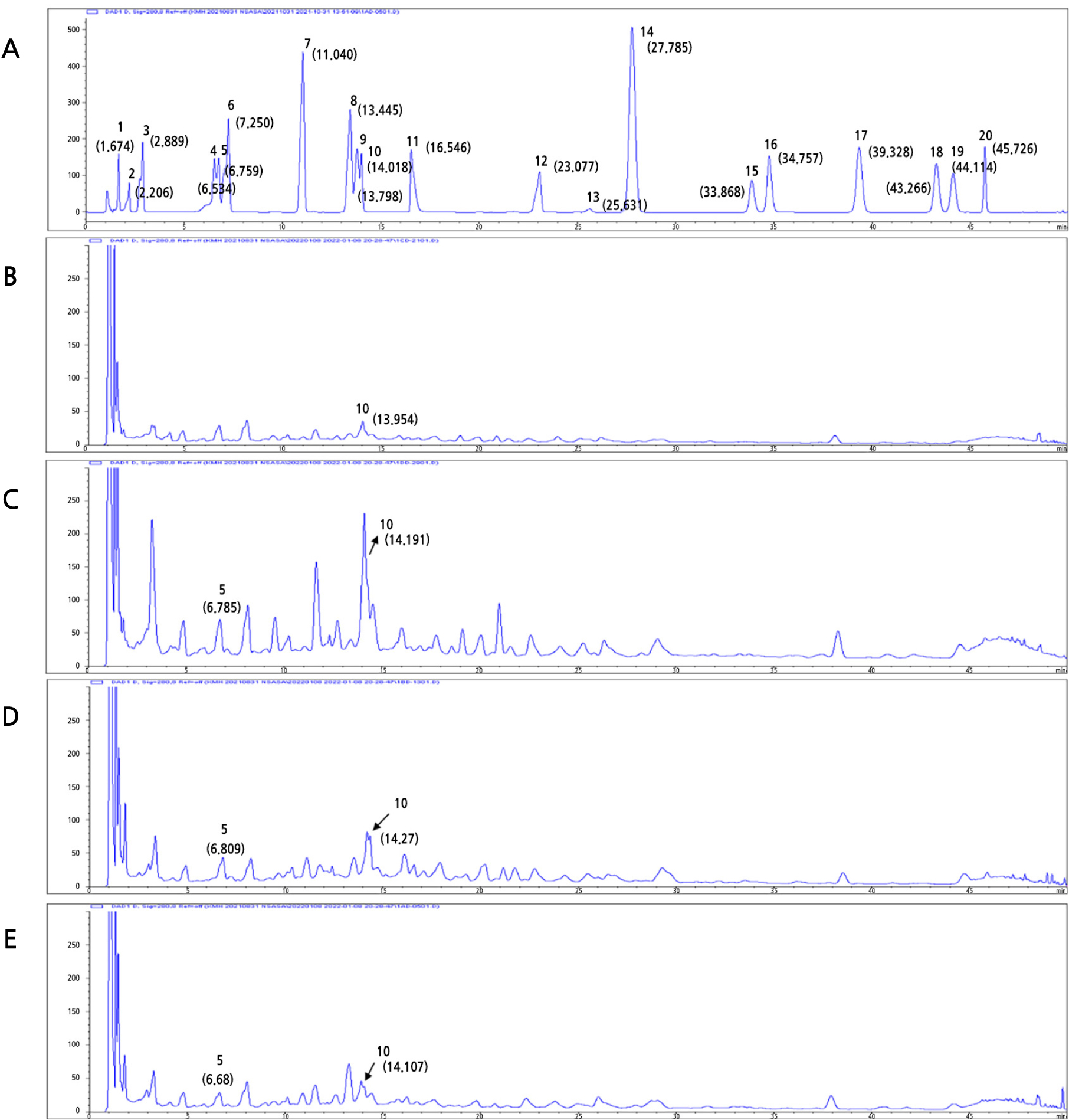
Fig. 4.
(A) High-performance liquid chromatograms of the phenolic compounds in SQW. The peaks represent the following phenolics: 1, gallic acid; 2, homogentisic acid; 3, protocatechuic acid; 4, chlorogenic acid; 5, vanillic acid; 6, caffeic acid; 7, p-coumaric acid; 8, ferulic acid; 9, veratric acid; 10, isoorientin; 11, ellagic acid; 12, naringin; 13, rosmarinic acid; 14, cinnamic acid; 15, quercetin; 16, luteolin; 17, naringenin; 18, apigenin; 19, kaempferol; 20, tricin. (B)-(E). SQW from February, May, August, and November.
The SQW from May had the highest TPC, TFC, vanillic acid, isoorientin contents as well as the highest cellular ROS inhibitory ability, whereas the SQW from November showed a greater antioxidant capacity than that from May. A possible explanation for these discrepant results is that leaves have large amounts of antioxidants, not detected in this study, in November. Further study of these compounds will be an interesting topic.
We analyzed the correlations among TPC, TFC, vanillic acid, the isoorientin contents, the anti-oxidant activities, and intracellular ROS production (Table 1). The vanillic acid and isoorientin contents of SQW demonstrated positive correlations with TPC (0.699 and 0.975, respectively) as well as TFC (r = 0.598 and 0.868, respectively). Also, TPC and TFC showed strong correlations with ROS production in PC12 cells (r = ‒0.800 and ‒0.877, respectively). The isoorientin content showed a negative correlation with intracellular ROS generation (r = ‒0.765), indicating that high levels of isoorientin, which is a major component of S. quelpaertensis, increase ROS inhibition. Regarding the correlation analysis data (Table 1), we can conclude that a high amount of isoorientin in SQW from May is a critical factor affecting the cellular ROS inhibitory ability. Isoorientin (6-C-glycosylflavone), which is present in corn (Zea mays) silks and pollens, as well as Pueraria tuberosa and Patrinia villosa, exhibits antiviral, antitumor, antioxidant, and anti-inflammatory activities (Peng et al., 2005; Yuan et al., 2016; Anilkumar et al., 2017; Li et al., 2020).
Table 1.
Correlations among TPC and TFC, vanillic acid and isoorientin contents, antioxidant activity, and ROS generation in PC12
Factors | TPCz | TFCy | Vanillic acid | Isoorientin | ABTS+x | DPPHx | ROSw |
TPCz | 1.000 | ||||||
TFCy | 0.879*** | 1.000 | |||||
Vanillic acid | 0.699* | 0.598* | 1.000 | ||||
Isoorientin | 0.975*** | 0.868*** | 0.613* | 1.000 | |||
ABTS+x | 0.508 | 0.304 | ‒0.110 | 0.594 | 1.000 | ||
DPPHx | 0.034 | ‒0.194 | ‒0.497 | 0.142 | 0.855*** | 1.000 | |
ROSw | ‒0.800** | ‒0.877*** | ‒0.399 | ‒0.765** | ‒0.269 | 0.153 | 1.000 |
In this study, S. quelpaertensis leaves harvested at different times showed differences in the chemical constitution of the phenolic compounds and the antioxidant properties. Leaves collected in spring, which have high amounts of phenolic compounds such as isoorientin, exhibited the best cellular ROS inhibitory ability in PC12 neuronal cells. Thus, spring is the optimal time to harvest S. quelpaertensis leaves, in the wild or in the field, for industrial use.
Conclusion
Seasonal variations in the contents of TP, TF, and isoorientin in SQW are related to changes in cellular ROS scavenging activities in neuronal cells. Our results indicate that spring is the best season to collect S. quelpaertensis leaves for industrial application owing to the high amounts of phenolic compounds such as isoorientin. S. quelpaertensis leaves harvested in the spring, with their high levels of neuroprotective bioactive compounds of vanillic acid and isoorientin, may help prevent neurodegenerative diseases.