Introduction
Materials and Methods
Plant material and experiment conditions
Polyphosphate treatment
Growth characteristics
Statistical analysis
Results and Discussion
Introduction
A plant factory is a production facility with a thermally insulated and nearly airtight warehouse-like structure containing multiple vertically stacked culture shelves fitted with electric lamps (Kozai et al., 2020). Such factors are recognized as a new growth driver for agriculture, for adding value, and for being eco-friendly. Prominent developed countries, including the United States, Japan, and several European nations, have allocated significant funding to this industry sector for decades on account of the expected substantial economic and industrial consequences (Kim, 2010). Because plant factories typically require significant capital investments in artificial lighting and environmental control systems (Kalantari et al., 2018), various studies have been conducted in an effort to improve the profitability of plant factories (Streed et al., 2021). Choi et al. (2023) analyzed the annual energy demand according to climate and environment changes outside of plant factories. To manipulate the growth and functional materials of the plants, the light intensity and photoperiod were adjusted inside the plant factories (Kim and Park, 2023). Additionally, researchers have also attempted to develop nutrient solution compositions to enhance lettuce growth in plant factories (Kwack et al., 2015).
Lettuce is a widely cultivated leafy vegetable that achieves widespread acceptance within the commercial domain of plant factories (Ahmed et al., 2020). The growth rate observed in the initial phases of lettuce development transcends that observed in the subsequent stages, exhibiting a sigmoidal growth pattern (Li et al., 2022). Therefore, if the initial growth rate is increased, the production cycle of lettuce in plant factories can be shortened, and economic profitability can be increased. Lettuce is a cool-season vegetable (Sublett et al., 2018), and its growth is affected by internal and external factors such as environmental conditions and the nutrient supply (Rizkiana et al., 2021; Montesdeoca-Flores et al., 2024). Therefore, efficient cultivation methods are required in plant factories to optimize lettuce growth (Hiroki et al., 2013). There are several ways to shorten the growth period, such as controlling the environmental conditions in plant factories, including the light, temperature, and CO2, and the controlling electrical conductivity (EC), the pH, the nutrients, and the oxygen levels in the root zone (Ahmed et al., 2020; Teodor et al., 2021).
The nutrient solution is one of the most important factors determining crop yield and quality outcomes (Trejo-Téllez and Gómez-Merino, 2012). Studies have focused on large amounts of certain elements, such as N, P, K, and Ca, and adjustments have been attempted in the field (Römer and Schilling, 1986; Zhang et al., 2017; Henriques and Marcelis, 2020). Various studies have investigated the compositions of nutrient solutions used for lettuce cultivation (Heinen et al., 1991; Fallovo et al., 2009; Kwack et al., 2015; Li et al., 2023). Nitrogen fertilizers both accelerate and delay the bolting of lettuce (Boroujerdnia and Ansari, 2007). Higher N levels positively affect the growth, yield, and quality of lettuce (Thapa et al., 2022). The NH4+:NO3‑ ratio affects the plug seedlings of horticultural crops (Lim and Jeong, 2001; Park et al., 2021) and lettuce cultivation (Noh and Jeong, 2023). Lettuce naturally absorbs large amounts of K and has high levels of K in its leaves (Zhang et al., 2017). Increasing the K concentration in the nutrient solution greatly affects the growth and yield of lettuce, especially the yield quantity and the Ca and Mg contents of the plant tissues (Inthichack et al., 2012).
Recently, hydroponic cultivation technologies using polyphosphates have become widespread. Polyphosphates are linear polymers consisting of orthophosphate units linked by phosphoanhydride bonds (Lorenzo-Orts et al., 2019). Polyphosphates are important stores of phosphate and energy (Zhu et al., 2020). Using polyphosphates in nutrient solutions significantly affects root system development and plant growth and induces earlier flowering (Torres-Dorante et al., 2006; Sobczak et al., 2020). Plants mainly absorb phosphorus as orthophosphate. The general formula of inorganic polyphosphates is Me(n+2)PnO(3n+1), where Me is a monovalent metal cation. The anions are composed of chains in which each phosphorus atom is linked to its neighbors via two oxygen atoms (Harold, 1966). Polyphosphate fertilizers must be broken down into the orthophosphate form, and polyphosphate hydrolysis involves enzymes of soil microorganisms and enzymes secreted by plant roots (Kulakovskaya et al., 2012). Polyphosphate-based fertilizers are used worldwide; however, information pertaining to how these fertilizers influence plant P acquisition is limited (Torres-Dorante et al., 2006). Most of the experiments thus far have focused on soil-based cultivation, with the results indicating the superiority of polyphosphates over monophosphates (Al-Dulaimi et al., 2021; Chtouki et al., 2021; Bae et al., 2023). In an experiment with soil-cultivated lettuce, the percentage of P in the plants at 36 days from sowing accounted for 60% of the variation in the plant dry weight (Costigan, 1984). Fertigation in hydroponic cultivation with a medium containing 30% phosphorus in the form of polyphosphates increased calcium uptake by pepper plants grown under LED lighting (Sobczak et al., 2020).
Information on effective hydroponic nutrients remains limited, but it is essential to increase productivity and maximize profitability (Frasetya et al., 2019). Our hypothesis is that the use of polyphosphates can enhance the growth and yield of lettuce cultivated in plant factories. Therefore, the purpose of this study was to evaluate whether there was a significant difference in the growth rate of lettuce seedlings between experimental groups using nutrient solutions with monophosphates only and nutrient solutions with monophosphates and different concentrations of polyphosphates and to improve profitability through faster growth during the early stages of lettuce development.
Materials and Methods
Plant material and experiment conditions
Seeds of Lactuca sativa L. ‘Caipira’ (Enza Zaden Co., Enkhuizen, the Netherlands) were sown in 128-cell plug trays (54 × 28 × 4.8 cm; Bumnong Co. Ltd., Jeongeup, Korea) filled with a commercial growing medium (Tosilee, ShinanGro Co. Ltd., Hapcheon, Korea) on December 27, 2021. After sowing, germination and seedling nurseries were carried out in a closed-type plant production system (CPPS, C1200H3, FC Poibe Co. Ltd., Seoul, Korea) fitted with RGB LEDs (red:green:blue = 7:1:2, ES LEDs Co. Ltd., Seoul, Korea) at a temperature of 25 ± 1°C, light intensity of 200 µmol·m-2·s-1 in terms of the photosynthetic photon flux density (PPFD), and a 12 h/12 h photoperiod (light/dark) for 26 days.
Polyphosphate treatment
Here, phosphorus was applied with various combinations of KH2PO4 (potassium monophosphate) and K5P3O10 (potassium polyphosphate). These were 32.4 + 15 (M2P1), 16.6 + 29.9 (M1P2), and 0.1 + 44.9 (M0P3) mg·L-1, while 48 mg·L-1 of phosphorus with KH2PO4 was used as a control (Table 1). By adjusting the amounts of KNO3 (potassium nitrate) and KH2PO4 (potassium monophosphate), the total amounts of phosphorus and other nutrients in the nutrient solution were kept the same, and only the ratios of monophosphate to polyphosphate were prepared differently. The nutrient solution prescribed was a commercial solution prescription (Greenbowl, Daegu, Korea) (Table 2).
Table 1.
Composition of the nutrient solutions used in the experiment
Treatment | Macro elements (me·L-1) | Micro elements (mg·L-1) | |||||||||||||
P | PPz | K | Ca | NH4 | NO3 | S | Mg | Fe | Mn | Zn | Cu | B | Mo | ||
Control | 4.6 | - | 7.3 | 5.3 | 0.5 | 9.2 | 5.4 | 3.1 | 1.68 | 0.82 | 0.46 | 0.05 | 0.49 | 0.06 | |
M2P1 | 3.1 | 1.5 | |||||||||||||
M1P2 | 1.6 | 2.9 | |||||||||||||
M0P3 | 0.1 | 4.3 |
Table 2.
Nutrient solution prescriptions used in the experiment
Growth characteristics
Plant height, leaf length, leaf width, the number of leaves, the leaf area, and the fresh and dry weights of shoots and roots were measured at three-day intervals starting at eleven days after sowing (DAS). The plant height from the soil line to the top of the plant was measured. Leaf area was measured using a leaf area meter (LI-3000; LI-COR Inc., Lincoln, NE, USA). Fresh weight was measured using an electronic balance (EW220-3 NM; Kern and Sohn GmbH., Balingen, Germany), and dry weight was measured after drying the same sample in an oven (Venticell-220, MMM Medcenter Einrichtungen GmbH., Planegg, Germany) at 70°C for 72 h. Root morphology parameters, in this case the total root length, average root diameter, root surface area, and root volume, were measured using an image analysis system (WinRhizo Pro 2007a, Regent Instruments, Sainte-Foy, QC, Canada) coupled with a professional scanner (Expression 1000XL; Epson America Inc., Long Beach, CA, USA). The relative growth rate (RGR), crop growth rate (CGR), and leaf area rate (LAR) were calculated using the following equations (Solanki and Saxena, 2022):
RGR (g·g-1·d-1) = (logew2–logew1) / (t2–t1)
CGR (g·m-2·d-1) = 1/Ga·(w2–w1) / (t2–t1)
LAR (cm2·g-1) = leaf area/dry weight of shoot
w1, w2: dry weight of plant measured on Jan. 3 and Jan. 21, respectively.
t1, t2: days between Jan. 3 and Jan. 21, respectively.
Ga: cultivated ground area
Statistical analysis
The experiment was laid out in a completely randomized design with three replicates per treatment, each consisting of 128 plants per plug tray. All assays were performed in triplicate, and the results were averaged. Statistical analyses were performed using Statistical Analysis System software (SAS 9.1; SAS Institute Inc., Cary, NC, USA). The results were subjected to an analysis of variance (ANOVA) with the procedure Proc GLM, and means were separated by the least significant difference (LSD) test or with Tukey’s multiple-range test. Graphs were created using SigmaPlot software (SigmaPlot 12.0; Systat Software Inc., San Jose, CA, USA).
Results and Discussion
Leaf growth was affected by different polyphosphate concentrations in the nutrient solution (Fig. 1). The leaf length, width, and area of lettuce in M0P3 were highest at 7.28, 5.23, and 76.28, respectively. The plants subjected to the M2P1 treatment, which had a low polyphosphate concentration, exhibited reduced lengths compared to the control plants. However, the plants treated with M1P2 and M0P3 showed greater heights relative to the controls. Leaf width showed a similar pattern to leaf length during the experimental period but differed in the last two investigations; the M1P2 and M0P3 treatments showed better results than the control plants at 5.23 and 5.02. Regarding the leaf area and fresh weight, M0P3 showed improved results compared to the control plants; M1P2 was similar to the control plants, and M2P1 was lower. Dry weight showed no significant differences between the treatments. There were no significant differences in the number of leaves among the experimental groups. Phosphorus has been suggested to play a key role in morphogenetic and leaf expansion processes related via cytokinin physiology (Fredeen et al., 1989). Information regarding the influence of polyphosphates on plant phosphorus acquisition is limited. In a pot experiment conducted under controlled conditions with corn (Zea mays L.), the efficiency of polyphosphate compounds was explained by improved root growth, which thereby improved the acquisition of phosphorus by the plant (Torres-Dorante et al., 2006).

Fig. 1.
Changes in the leaf length (A), leaf width (B), number of leaves (C), leaf area (D), fresh weight of the shoots (E), and dry weight of shoots (F) of ‘Caipira’ lettuce as affected by different polyphosphate concentrations in the nutrient solution (n = 12). Different letters indicate statistically significant differences according to LSD testing at p < 0.05. Table 1 presents details on the nutrient solution treatments.
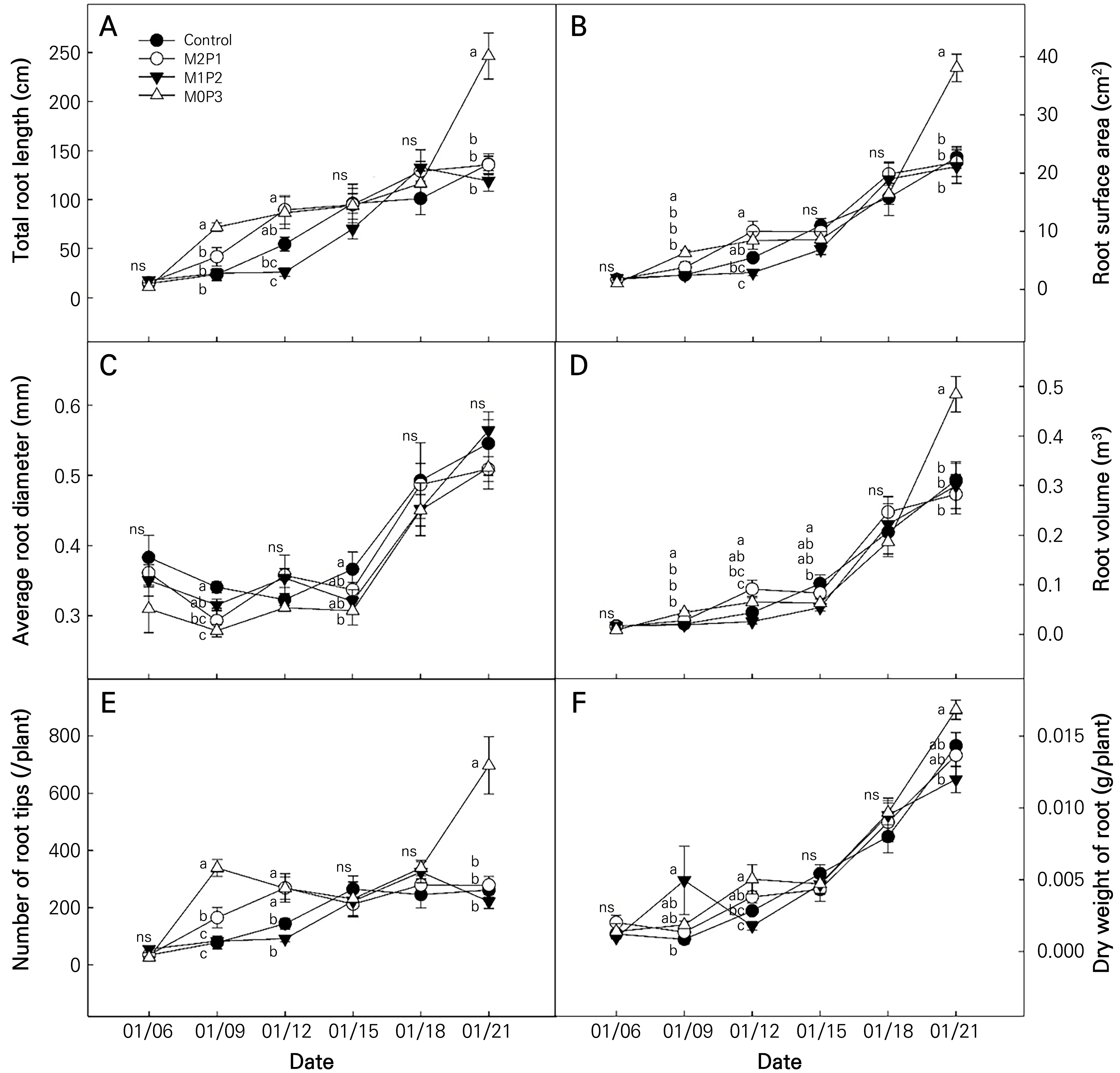
Fig. 2.
Changes in the total root length (A), root surface area (B), average root diameter (C), root volume (D), number of root tips (E), and dry weight of roots (F) of ‘Caipira’ lettuce as affected by different polyphosphate concentrations in nutrient solution (n = 12). Different letters indicate statistically significant differences according to LSD testing at p < 0.05. Table 1 presents details on the nutrient solution treatments.
Changes in the roots were affected by the different polyphosphate concentrations in the nutrient solution (Figs. 2 and 3). Root development differed significantly among the treatments at an early stage. In particular, at 14 DAS (Jan. 09), the total root length and number of root tips were greatest in M0P3 at 71 and 338 cm, respectively; however, the average root diameter was thinnest in the M0P3 treatment at 0.28 mm. The root surface area, volume, and dry weight of the roots were also greatest in M0P3. This root development indicates that a new root system was developed in M0P3 compared to the other treatments. Well-developed root development, especially when promoted at an earlier stage, induces better nutrient absorption (Hodge et al., 2009). In addition, the low average root diameter and numerous root tips indicate that plants have fine and branched root systems (Jeong et al., 2021). In the pot experiments, root growth and phosphorus uptake preceded shoot growth. The high phosphorus uptake rate in the early stages of the life cycle reflects the high phosphorus requirements of plants (Römer and Schilling, 1986).
The growth of lettuce seedlings increased with an increase in the polyphosphate concentrations (Table 3 and Fig. 4). In M2P1, which had the lowest concentration of polyphosphate, growth decreased compared to that of the control using a normal phosphate. However, the growth of M1P2 was similar to that of the control. In M0P3, which contained the highest polyphosphate concentration, the plant height, leaf length, fresh and dry weights of the shoots and roots, and the leaf area increased significantly. However, no significant differences in SPAD or the number of leaves were found.
Table 3.
Growth characteristics of ‘Caipira’ lettuce seedlings affected by different polyphosphate concentrations in the nutrient solution (n = 12)
Treatmentz |
Plant height (cm) |
Leaf length (cm) |
Leaf width (cm) |
Leaf area (cm2/plant) |
Number of leaves (/plant) | SPAD | Fresh weight (g) | Dry weight (g) | |||
shoot | root | shoot | root | ||||||||
Control | 6.14 by | 6.04 b | 5.00 a | 55.06 b | 6.08 a | 25.11 a | 2.68 b | 0.22 b | 0.15 ab | 0.01 b | |
M2P1 | 5.13 c | 4.90 c | 4.09 b | 41.01 c | 6.50 a | 26.74 a | 2.05 b | 0.20 b | 0.12 b | 0.01 b | |
M1P2 | 6.53 b | 6.48 ab | 5.01 a | 57.33 b | 6.00 a | 22.10 a | 2.68 b | 0.22 b | 0.13 b | 0.01 b | |
M0P3 | 7.62 a | 7.27 a | 5.22 a | 76.28 a | 6.58 a | 23.30 a | 3.41 a | 0.40 a | 0.17 a | 0.02 a |
The RGR of M2P1 was lower than that of the control, and there was no significant difference in the LAR outcome. In terms of CGR, M2P1 and M1P2 were lower than the control, and a significant difference was observed for M0P3 (Table 4). Polyphosphorus metabolism in plants is readily identifiable but is complexly related to enzymatic activities and polymer synthesis (Seufferheld and Curzi, 2010). Phosphorus is involved in many plant metabolic functions and regulates many processes; adequate phosphorus supplementation in plant roots promotes cell division and increases plant growth (Razaq et al., 2017). In other experiments, a polyphosphate application not only significantly increased phosphorus uptake but also micronutrient availability (e.g., Fe, Mn, and Zn), accounting for the additional agronomic benefits of polyphosphates (Khourchi et al., 2022).
Table 4.
Growth parameters of ‘Caipira’ lettuce seedlings affected by different polyphosphate concentrations in the nutrient solution (n = 12)
Treatmentz | RGRy (g-1·g-1·d-1) | CGRx (g-1·m-2·d-1) | LARw (cm-2·g-1) |
Control | 0.146 av | 0.0007 ab | 5,232 a |
M2P1 | 0.101 b | 0.0005 b | 5,006 a |
M1P2 | 0.143 a | 0.0005 b | 7,574 a |
M0P3 | 0.163 a | 0.0010 a | 4,847 a |
In conclusion, the growth of the roots and leaves of lettuce seedlings treated with 45 mg·L-1 of phosphorus and polyphosphate produced the best results. After the final stage of the experiment, the lettuce was planted and grew rapidly. If the planting stage can be improved as much as possible by inducing good initial growth, as in the experiment here, the profitability of a plant factory can be improved because the harvesting period can be shortened. Therefore, using polyphosphates in nutrient solution prescriptions may be beneficial.