Introduction
Materials and Methods
Sample preparation and preharvest treatments
Measurement of physicochemical properties
Determination of the respiration rate
Statistical analysis
Results
Room-temperature storage experiment
Cold storage experiment
Discussion
Room-temperature storage experiment
Cold storage experiment
Conclusion
Introduction
Mango (Mangifera indica L.), which belongs to the Anacardiaceae family (Mwaurah et al., 2020; Seehanam et al., 2022), is considered as a valuable and essential economic fruit that has gained commercial importance in more than 87 countries. According to recent metrics, the global mango production volume in 2021 exceeded 57 million metric tons, slightly higher than the 56.69 million metric tons recorded in 2020. Comparing the production values from the previous five and ten years, there were increases of 20.5% and 45.2%, respectively, in the mango production volume (FAO, 2023). Mangoes are primarily grown in tropical and subtropical regions in Asia, South and Central America, and Africa. Known as the “king of fruits” (Chung et al., 2021), mangoes are praised for their delicious flavor, nutritional value, vibrant color, and enticing aroma. They are rich in phytochemical components, making them a valuable addition to a healthy diet. Mango consumption provides an outstanding source of antioxidants (Eshetu et al., 2019), which can help reduce the risk of certain cancers, slow down the aging process, improve lung function, and assist in managing diabetes (Eshetu et al., 2019; Vilvert et al., 2022). These health benefits and desirable attributes contribute to the high demand for mangoes in both domestic and foreign markets.
As a climacteric fruit, mangoes have a limited shelf life and achieve respiration peaks on the third or fourth day after being harvested under room-temperature conditions (Eshetu et al., 2019; Sánchez Aldana et al., 2021). The biochemical and metabolic processes that occur during fruit maturation lead to various chemical changes, which ultimately result in the fruit reaching a desirable quality. These changes include an increase in the respiration rate and ethylene synthesis, softening owing to changes in polysaccharides, the destruction of chlorophyll and the synthesis of carotenoids, the conversion of carbohydrates or starch into sugars, and modifications of organic acids, lipids, phenolics, and volatile substances (Kundu et al., 2020).
Several approaches have been suggested to inhibit deterioration, prolong the shelf life, and maintain the quality of mango fruit (Ramayya et al., 2012; Khaliq et al., 2016; Mg et al., 2019). Edible coatings are among the innovative technologies and tactics utilized to maintain the physiochemical and organoleptic qualities of fruits while reducing losses. The post-harvest characteristics and shelf life of processed and non-processed fruit and vegetables are ameliorated by the post-harvest application of edible coating (Kumar et al., 2019; Mg et al., 2019). The concept of an edible coating stemmed from the requirement for higher quality produce to meet consumer needs (Kumar et al., 2020). An edible coating, notably the application of chitosan, is an affordable, simple, and effective approach for preventing moisture loss and lowering the rates of decomposition and respiration. It is a biocompatible and biodegradable high-molecular-weight cationic polysaccharide produced from chitin. Chitosan poses no toxicity risk, is safe to use biologically, and is also a naturally occurring biopolymer made from chitin that has been deacetylated and contains (1, 4)-linked 2-amino-deoxy-β-d-glucan (Sharma et al., 2016; Mg et al., 2019). Owing to its film-forming, biochemical, and antimicrobial capabilities, it has a wide range of applications in fruits and vegetables (Devlieghere et al., 2004). However, chitosan polymers are beneficial in combination and as a single treatment. Examples of studies in this area include those focusing on the application of Aloe vera gel and chitosan combinations for mango (Shah and Hashmi, 2020), edible coatings consisting of essential oils and Mexican lime (Citrus aurantiifolia Swingle) pectic extract on Haden mangoes (Sánchez Aldana et al., 2021), and a chitosan-rhamnolipid coating on ‘Agege Sweet’ oranges (Adetunji et al., 2018). Several studies have endeavored to maintain the quality and prolong the post-harvest shelf life of horticultural produce (Sharma et al., 2016).
Modified atmosphere packaging (MAP) is another preservation method used widely to enhance the shelf life of a broad range of fresh fruits and vegetables (Perumal et al., 2021; Byeon et al., 2022). This method can potentially increase the post-harvest life of fruit by increasing carbon dioxide concentrations and reducing oxygen around the fruit owing to its metabolic activity (Cukrov et al., 2019; Vilvert et al., 2022). The interior environment of the MAP system, which has reduced O2 and elevated CO2 levels (Medeiros et al., 2012), and the gas permeability of the container holding the fresh food cause certain beneficial interactions (Perumal et al., 2021). MAP also delays the physiological, biochemical, and organoleptic changes associated with fruit ripening, including ethylene production and increased respiration rates, color alterations, softening, increased sugar content, and reduced acidity levels (Vilvert et al., 2022). Typically, fruit is covered in hermetically sealed polyethylene bags to apply the MAP system. Polyethylene is a polymer with a few advantageous properties, including flexibility, strength, a light weight, stability, moisture resistance, chemical resistance, and simplicity of processing (Marsh and Bugusu, 2007). Several researchers have also reported the use of MAP for preserving fruit (Perumal et al., 2021), such as pomegranates (Candir et al., 2018), bananas (Mendoza et al., 2016), avocados (Sellamuthu et al., 2013), and mangoes (Phakdee and Chaiprasart, 2020; Vilvert et al., 2022).
In addition to the techniques described above, proper temperature management is essential for extending the shelf life of perishable commodities, such as mango, and this factor has the main environmental impact on fruit quality (Ambuko et al., 2018; Hesami et al., 2021). The deterioration rate of perishables is reported for every 10°C increase in temperature; the rate at which perishables deteriorate multiplies by two to three (Ambuko et al., 2018). Consequently, the shelf life of perishable products can be extended by lowering the moisture loss, respiration rate, and ethylene gas sensitivity by storing the fruit at the lowest safe temperature (10–12°C for chilling-sensitive tropical crops or 0°C for temperate crops) (Ambuko et al., 2018). Storing perishable commodities at temperatures above or below safe optimal levels primarily contributes to post-harvest losses as it accelerates the deterioration processes (Edmunds et al., 2008; Ambuko et al., 2018). Previous research has shown that combining MAP and cold storage results in a synergistic or cumulative effect that is superior to any approach applied independently (Phakdee and Chaiprasart, 2020). Therefore, this study examined the effectiveness of chitosan edible coatings and MAPs on the enhancement of the overall post-harvest quality of fully ripe ‘Irwin’ mango with forcing culture cultivation under two different storage conditions. The findings are anticipated to provide ideal strategies for the packaging and storage of ‘Irwin’ mangoes during long-distance transportation, which holds substantial practical importance.
Materials and Methods
Sample preparation and preharvest treatments
The ‘Irwin’ mangoes (Mangifera indica L. ‘Irwin’) used in this experiment were grown in a heated plastic greenhouse in Haman, Gyeongsangnam-do, South Korea. The high-molecular-weight chitosan used here (500,000 MW) was purchased from JS Logistic, Donkyo-ro, Mapo-gu, Seoul, Republic of Korea. For the first treatment, ‘Irwin’ mango fruits were fully dipped into chitosan at 100 µL·L-1 and 500 µL·L-1 in the form of a solution (chitosan 2% w/v + 0.2 M acetic acid + 0.02% surfactant). During this treatment process, the mangoes were treated with separate 100 µL·L-1 and 500 µL·L-1 treatments twice by fully dipping the whole fruit for two minutes, on June 1, 2022 and on June 8, 2022, started treating two weeks before harvesting. Then, both the pre-treated and non-treated ‘Irwin’ mangoes were harvested on June 15, 2022 at a fully ripe stage (0% green colored stage) without any external defects or disease occurrence. They were covered with expanded polyethylene (EPE) fruit foam nets and packed evenly in plastic cartons. Subsequently, they were immediately transported to the Laboratory of Fruit Science, Gyeongsang National University, South Korea, in a fully air-conditioned state for further analysis. Fig. 1 presents the experimental procedure and treatments. The harvested mangoes were sorted, and those uniform in size were divided into two batches, termed batch 1 and batch 2 for two experiments. They were initially weighed using a digital scale (Electric indicator scale-Bas 610, Chiba, Japan).
Room-temperature storage experiment
Among them, twenty non-treated mangoes of batch 1 were treated with the 500 µL·L-1 chitosan solution as a post-harvest treatment. In this case, the mango fruits were fully dipped in the chitosan solution for two minutes and then taken outside and air dried until the whole fruit surface was completely dried. The other two groups of twenty mangoes were packed with low-density polyethylene (LDPE) MAP materials with oxygen transmission rates (OTR) of 80,000 cc and 150,000 cc (Force Tech Co., Ltd., Manjangsan-ro, Gwangtan-myeon, Paju-si, Gyeonggi-do, Republic of Korea). During this treatment, each fruit was enclosed in individual MAP packaging (52 µm × 30 cm × 20 cm) and sealed completely against the outer environment after the initial weights were recorded. Batch 1 consisted of control treatment, 100 µL·L-1 and 500 µL·L-1 chitosan preharvest treatments, 500 µL·L-1 chitosan post-harvest treatment, and 80,000 cc and 150,000 cc MAP treatments (Fig. 1). The control and 100 µL·L-1 and 500 µL·L-1 chitosan preharvest treatments were replicated with seven biological replicates (fruits), while both the 500 µL·L-1 chitosan postharvest treatment and the 80,000 cc and 150,000 cc MAP treatments were replicated with five biological replicates (fruits). These treated fruits were kept in room-temperature storage at 20°C and were subjected to an analyses of their physiochemical characteristics and respiration rates 0, 2, 6, 9, and 13 days after harvest (DAH).
Cold storage experiment
Similarly, batch 2 consists of the control treatment and the 100 µL·L-1 and 500 µL·L-1 chitosan preharvest treatments. They include seven replicates for each treatment on each sampling date. These treated fruits were kept in cold storage at 7°C for 14 days and were transferred to the room-temperature storage condition at a temperature of 20°C to simulate commercial preservation conditions. These ‘Irwin’ mangoes were analyzed at intervals of 0, 2, and 5 days after storage (DAS) to ascertain their physicochemical characteristics and respiration rates (Fig. 1).
Measurement of physicochemical properties
The physicochemical properties, in this case the weight loss% (WL%), soluble solids content (SSC), firmness, titratable acidity (TA), and chromaticity [L*, a*, b*], were measured. The same physicochemical parameters were examined in two batches for the two corresponding experiments.
Measurement of WL%
The weight loss values of the treated and non-treated mango fruits were calculated at each sampling date according to the following equation, WL% = [(wi–wf) / wi] × 100, where wi is the initial weight of each sample and wf is the final weight of the sample at the given sampling date. The weight loss values are expressed as a percentage (%).
Measurement of the SSC
Here, the fruit was sliced into two longitudinal halves and SSC measurements were taken from the flesh portion in the center of a seedless slice. The square-shaped peeled mango flesh part was enfolded with a four-layer cheesecloth and squeezed, with the absorbance value then obtained using a hand refractometer (Pocket Refractometer, PAL-1, Atago Co. Ltd., Tokyo, Japan) calibrated in °Brix (Kumarihami et al., 2020). The °Brix (%) range of the refractometer was 0 to 53% with a 0.1% Brix resolution at room temperature, and it had a refractive index precision level of ± 0.2 (Jang et al., 2022).
Measurement of the TA
The TA was measured using a pH meter (BP3001, Trans Instruments, Jalan Kilang Barat, Singapore). Titration was carried out using 80 mL of distilled water diluted with 1 mL of fruit juice that had been extracted from pulp. NaOH (0.1 mol·L-1) was then added at a rate of 1 mL·min-1 to achieve a pH of 8.3 (Jang et al., 2022).
Measurement of firmness
The firmness of the ‘Irwin’ mango fruit was assessed using a rheometer (CR-100, Sun Scientific Inc., Tokyo, Japan) equipped with an 8 mm round flat probe by compressing the flesh to a depth of 3 mm with a crosshead speed of 2 mm·s-1 (Jang et al., 2022). Flesh firmness was measured on a previously cut longitudinal slice with the seed. The maximum force produced during penetration was represented as the firmness in Newton (N) (Kumarihami et al., 2020).
Measurement of chromaticity
The color values of mango samples were measured using a portable colorimeter (CR-400, Konica Minolta Inc., Osaka, Japan). The L*, a*, and b* chromaticity values were taken in both the front and back sides of the mangoes in both batches after white calibration was completed. These values were taken as a measurement of the fruit peel of mango fruits.
Determination of the respiration rate
Carbon dioxide production was determined by weighing each fruit, sealing them in 2.1 L plastic boxes for 2 h, and injecting 1 mL of headspace gas into a gas chromatograph (GC-2014, Shimadzu Co., Ltd., Kyoto, Japan) according to the method explained by Thammawong and Arakawa (2007). The respiration rate was measured using a SUS column (4 m × 2.2 mm) and a TCD detector with helium as the carrier gas under the following conditions: a column temperature of 35°C, an injector temperature of 250°C, and a detector temperature of 250°C. The respiration rate was then measured as an indicator of the CO2 concentration, expressed as mg CO2·kg-1·hr-1.
Statistical analysis
This experiment was set up using a completely randomized design (CRD). The statistical analyses were conducted via an analysis of variance (ANOVA) for repeated measurements, and time was considered as a covariate. Tukey’s multiple range test (p < 0.05) was used to determine differences among statistically significant variables. All data obtained were analyzed statistically using JMP Pro software (Version 16.1.0, SAS Institute, Cary, NC, USA). All results are denoted as the mean value ± standard error (SE).
Results
Room-temperature storage experiment
Changes in physicochemical properties
Changes in WL%
The weight loss% increased dramatically throughout the storage period of 13 days at room temperature (20°C), as shown in Fig. 2. Compared to the two MAP treatments, the other chitosan treatments and the control treatment showed a significantly greater increase in the weight loss throughout the storage period at 20°C. The chitosan post-harvest treated fruits showed the largest increase (11.2%) at the end of the storage period in all other treatments. There was no significant difference between the two MAP treatments, apart from those of the other treatments on every sampling date (Fig. 2).
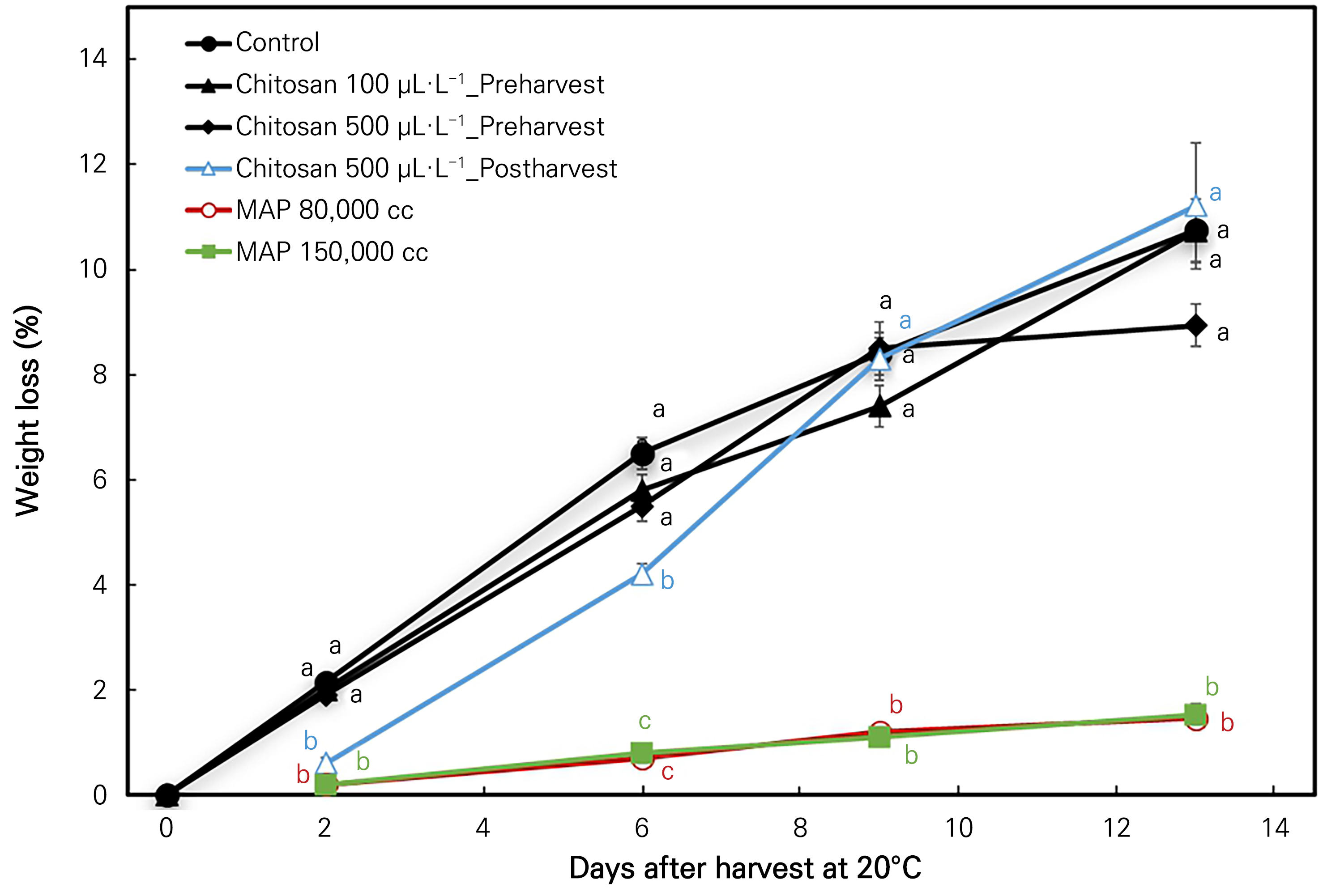
Fig. 2.
Weight loss (%) changes in ‘Irwin’ mango during room-temperature storage at 20°C for 13 days in response to the preharvest and post-harvest chitosan treatments and modified atmosphere packaging (MAP). Vertical bars indicate the standard error and means, with different letters (a-c) indicating significant differences between the treatments at each sampling date according to Tukey’s test at p <0.05.
Changes in the SSC
The soluble solids content is a foremost physiochemical characteristic preferred mostly by growers when they make predictions pertaining to the fruit maturity of mangoes (Meena and Asrey, 2018). As shown in Fig. 3, the SSC values did not show any particular trend in their variation. The two MAP treatments showed a significant decrease in the SSC content by the end of the storage period. The control treatment showed the highest values of 16.1°Brix and 15.1°Brix on the 6th and 13th days, respectively. Similarly, the MAP 80,000 cc treatment showed a significant decrease throughout the storage period compared to the other treatments. Significant differences were noted between the treatments on each storage day, except for the 0th and 2nd days of the storage period (Fig. 3).
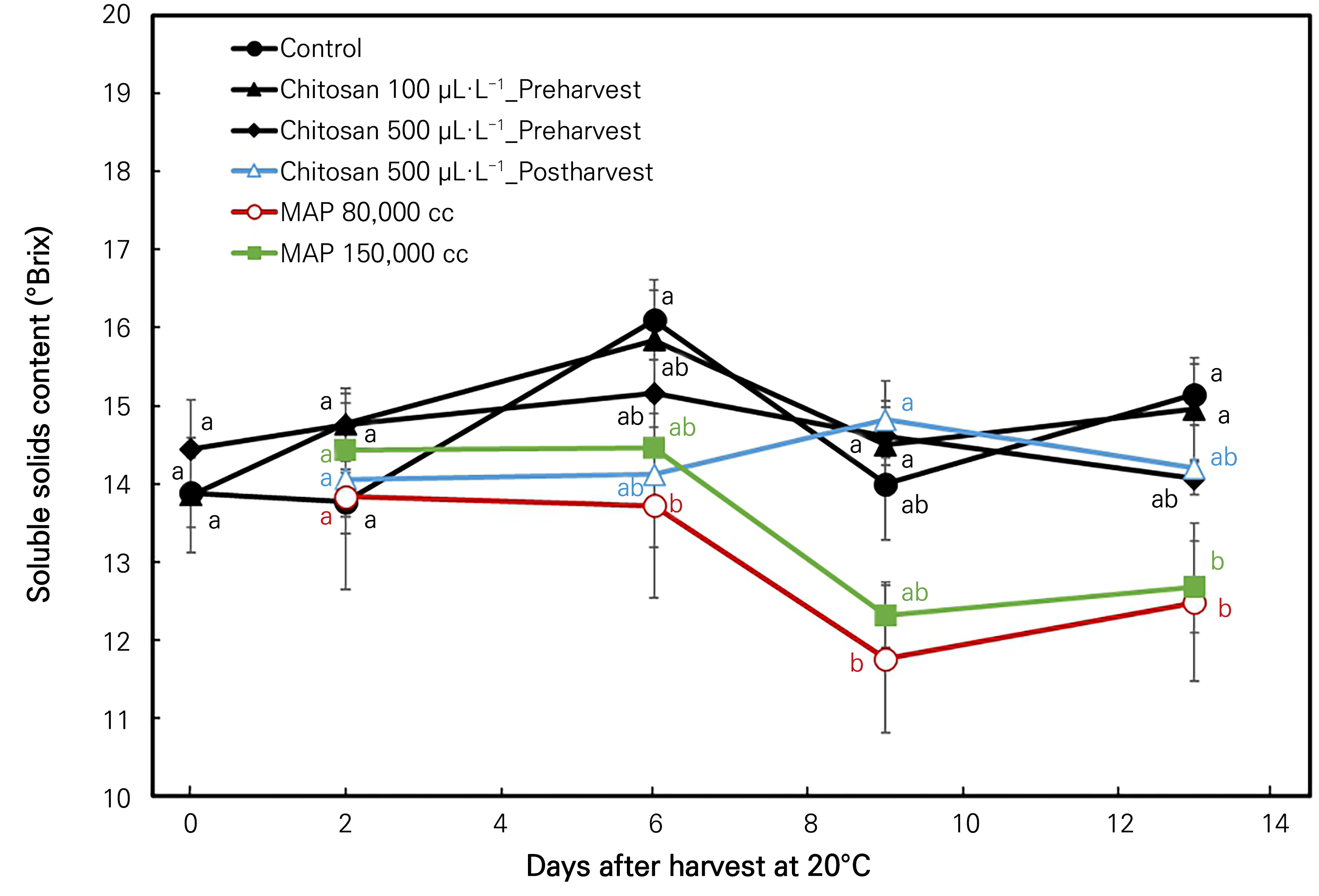
Fig. 3.
Soluble solids content (°Brix) changes in ‘Irwin’ mango during room-temperature storage at 20°C for 13 days in response to the preharvest and post-harvest chitosan treatments and MAP treatments. Vertical bars indicate the standard error and means with different letters (a-c) indicate significant differences between the treatments at each sampling date according to Tukey’s test at p < 0.05.
Changes in the TA
The TA variations also decreased throughout the storage period for each treatment (Fig. 4). However, the 500 µL·L-1 post-harvest chitosan treatment showed a deviation; it increased significantly on the 6th day of storage and then decreased. The highest retention was obtained from the post-harvest chitosan application. The treated fruits showed significant differences in every sampling date except for the first and the last day of storage. (Fig. 4).
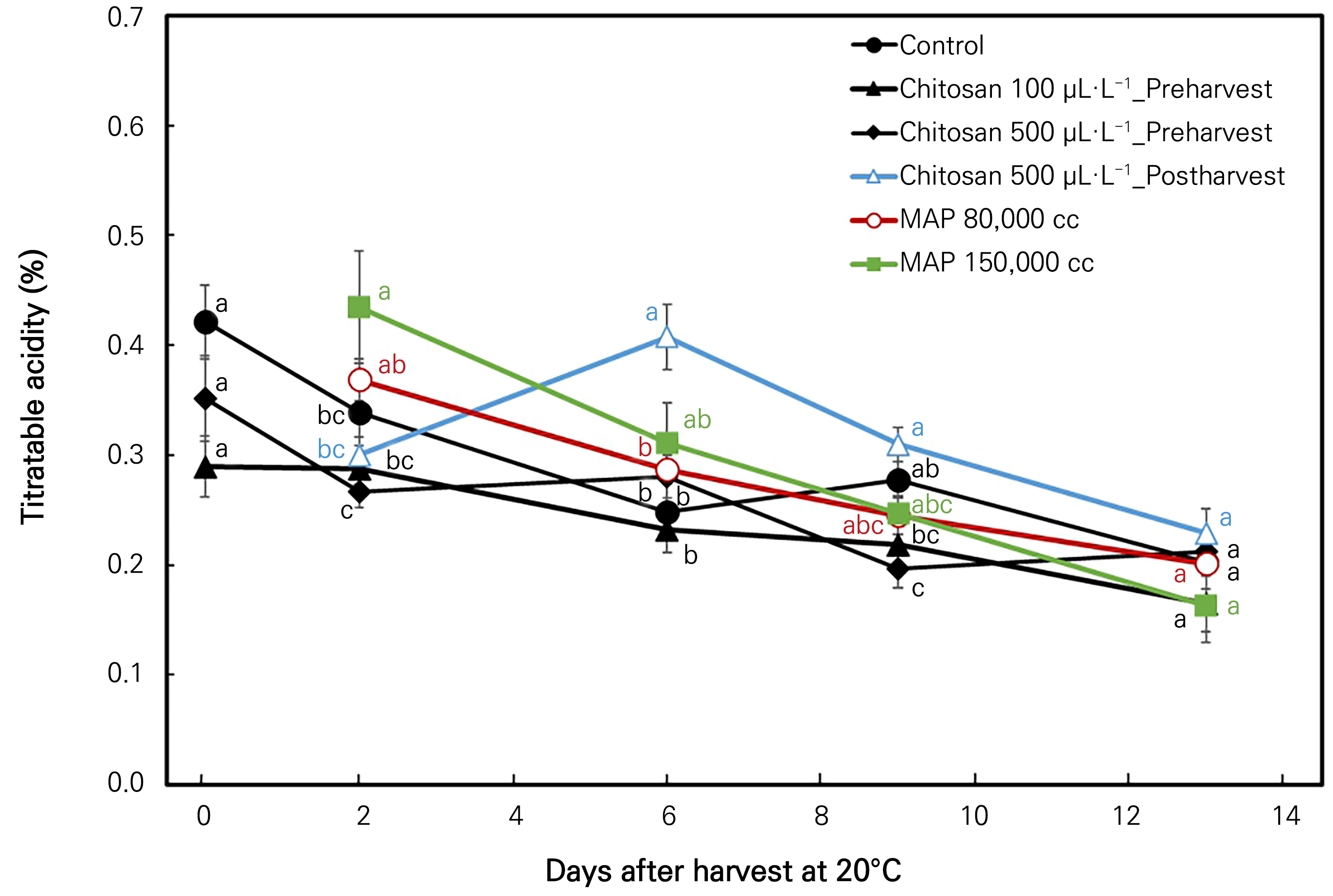
Fig. 4.
Titratable acidity (%) changes in ‘Irwin’ mango during room-temperature storage at 20°C for 13 days in response to preharvest and post-harvest chitosan treatments and MAP treatments. Vertical bars indicate the standard error and means with different letters (a-c) indicate significant differences between the treatments at each sampling date according to Tukey’s test at p < 0.05.
Changes in the SSC/TA ratio
In our study, it is interesting to note that the SSC/TA ratio increased dramatically over 13 days of storage in all treatments of fully ripened ‘Irwin’ mango (Fig. 5). Nevertheless, a significant reduction of this ratio was noted on the 6th, 9th, and 13th day of storage in the 500 µL·L-1 chitosan postharvest treatment, the control treatment, and the 500 µL·L-1 chitosan preharvest treatment, respectively.
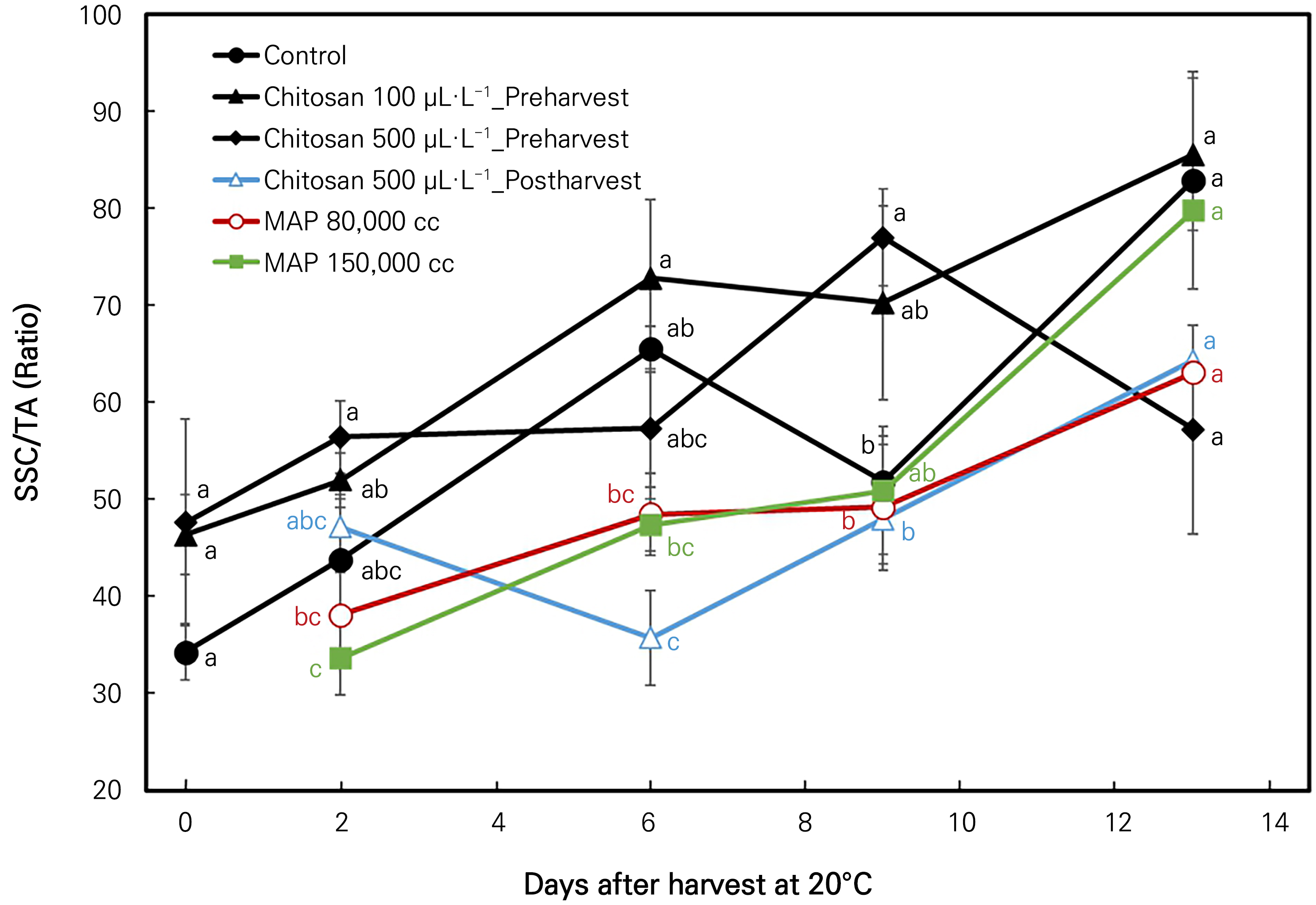
Fig. 5.
SSC/TA (ratio) changes in ‘Irwin’ mango during room-temperature storage at 20°C for 13 days in response to preharvest and post-harvest chitosan treatments and modified atmospheric packaging (MAP). Vertical bars indicate the standard error, and means with different letters (a-c) indicate significant differences between the treatments at each sampling date according to Tukey’s test at p <0.05.
Changes in fruit firmness
The variation in the fruit firmness of the treated and non-treated ‘Irwin’ mangoes with the storage time at room temperature is presented in Fig. 6. Here, the fruit firmness showed less variation throughout the storage period. There are no significant differences among the treatments on each sampling date.
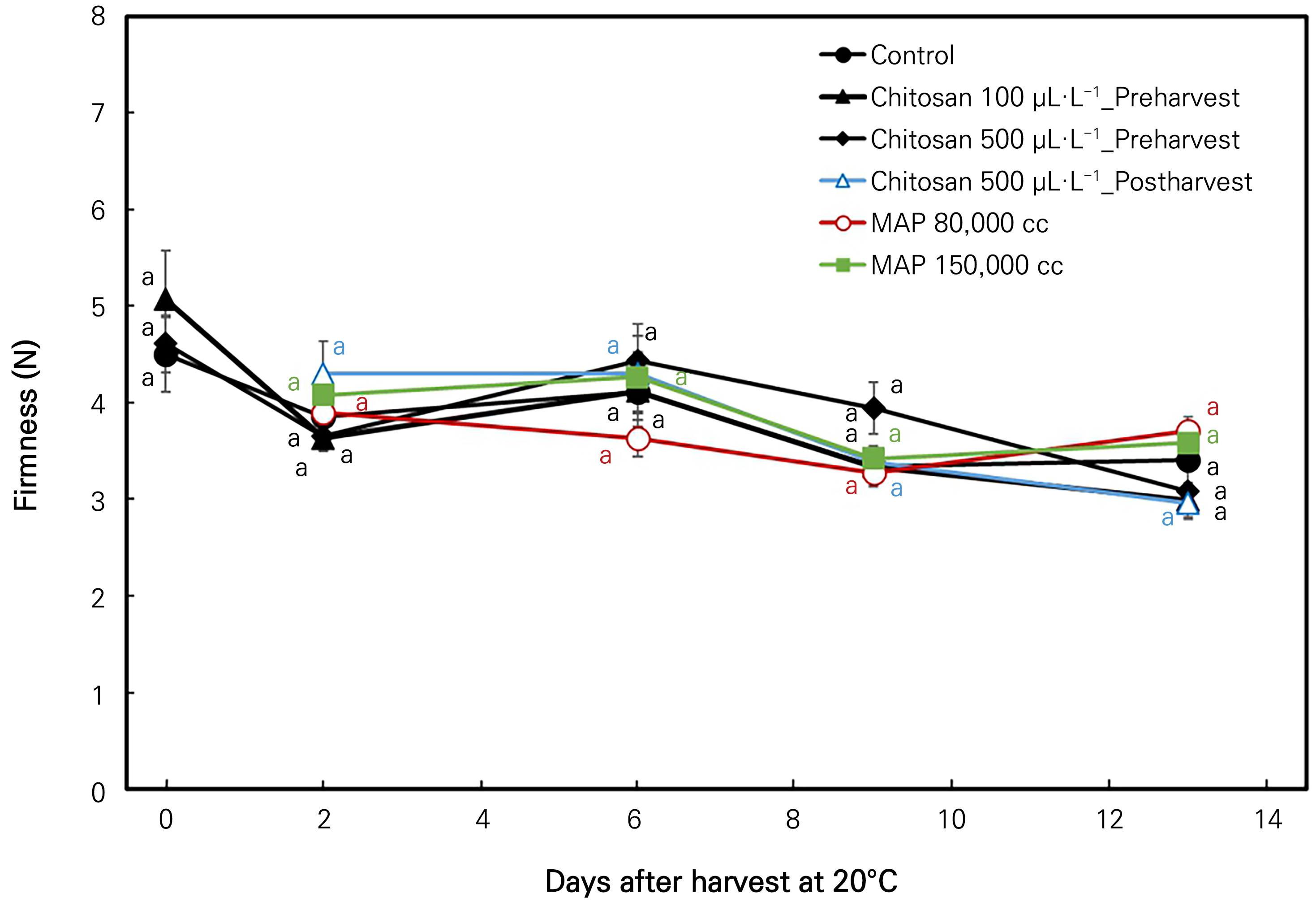
Fig. 6.
Firmness (N) changes in ‘Irwin’ mango during room-temperature storage at 20°C for 13 days in response to preharvest and postharvest chitosan treatments and MAP treatments. Vertical bars indicate the standard error and means with different letters (a-c) indicate significant differences between the treatments at each sampling date according to Tukey’s test at p < 0.05.
Changes in L*, a*, and b* color values
Considering the L* color values (color lightness) (Wijethunga et al., 2023) of the ‘Irwin’ mango fruits in storage at 20°C (Table 1), both the MAP treatments and the chitosan 100 µL·L-1 preharvest treatment did not show significant differences between the DAH periods on both sides, while the control and chitosan 500 µL·L-1 preharvest treatments showed significant differences in both sides of the fruit. However, the 500 µL·L-1 chitosan post-harvest treatment showed significant differences in the L* color values only on the back side (Table 1).
Table 1.
Effects of different chitosan treatments and MAP treatments on the color of the front and back sides of ‘Irwin’ mangoes during storage at room temperature (20°C)
Treatment (A) | DAH (B) | Color | |||||
L* | a* | b* | |||||
Front | Back | Front | Back | Front | Back | ||
Control | 0 | 41.2 ab | 41.7 ab | 24.0 a | 26.7 a | 23.7 a | 23.7 abz |
2 | 48.3 a | 47.8 a | 16.2 b | 16.1 b | 13.3 a | 11.9 b | |
6 | 37.9 b | 43.2 ab | 26.2 a | 25.1 a | 20.6 a | 27.7 a | |
9 | 37.1 b | 36.4 b | 28.2 a | 25.8 a | 20.1 a | 19.4 ab | |
13 | 39.7 ab | 40.4 ab | 22.4 ab | 21.3 ab | 23.9 a | 24.9 a | |
Chitosan 100 µL·L-1 -Preharvest | 0 | 41.6 a | 38.1 a | 22.1 ab | 25.0 a | 24.5 ab | 19.7 a |
2 | 47.2 a | 47.4 a | 14.2 b | 17.3 a | 11.5 b | 11.4 a | |
6 | 39.1 a | 40.4 a | 25.9 a | 26.8 a | 22.5 ab | 24.4 a | |
9 | 40.5 a | 40.5 a | 25.6 a | 25.6 a | 24.3 ab | 24.3 a | |
13 | 41.4 a | 38.7 a | 24.1 a | 24.2 a | 25.5 a | 22.5 a | |
Chitosan 500 µL·L-1 -Preharvest | 0 | 42.2 ab | 42.3 a | 24.3 a | 26.1 a | 26.0 a | 26.1 a |
2 | 49.0 a | 45.9 a | 15.2 b | 17.2 b | 13.8 a | 11.0 c | |
6 | 39.6 ab | 41.5 ab | 24.9 a | 25.5 a | 22.4 a | 25.1 ab | |
9 | 40.7 ab | 34.3 b | 27.6 a | 26.6 a | 24.3 a | 15.8 bc | |
13 | 34.8 b | 41.0 ab | 23.7 a | 22.8 ab | 17.8 a | 26.3 a | |
Chitosan 500 µL·L-1 -Postharvest | 2 | 45.4 a | 50.6 a | 17.9 a | 15.9 a | 11.3 b | 18.0 a |
6 | 43.3 a | 43.1 ab | 25.2 a | 20.5 a | 28.6 a | 26.6 a | |
9 | 37.1 a | 37.8 b | 25.8 a | 24.0 a | 20.4 ab | 18.9 a | |
13 | 35.6 a | 42.8 ab | 21.3 a | 14.4 a | 18.4 ab | 26.0 a | |
MAP 80,000 cc | 2 | 47.4 a | 45.3 a | 14.4 b | 14.8 b | 16.1 a | 12.1 b |
6 | 42.2 a | 40.5 a | 27.4 a | 27.1 ab | 25.7 a | 23.9 ab | |
9 | 39.7 a | 44.6 a | 27.3 a | 21.2 a | 22.4 a | 30.7 a | |
13 | 40.0 a | 40.5 a | 19.9 b | 24.2 ab | 23.5 a | 22.6 ab | |
MAP 150,000 cc | 2 | 45.6 a | 50.6 a | 15.6 b | 14.4 c | 12.6 b | 11.9 b |
6 | 37.7 a | 43.1 a | 30.4 a | 27.6 a | 20.1 ab | 23.7 ab | |
9 | 42.3 a | 37.8 a | 28.6 a | 28.2 a | 27.7 a | 30.2 a | |
13 | 39.9 a | 42.8 a | 26.1 a | 23.8 b | 24.3 a | 26.1 a | |
F-testy | A | NS | NS | NS | NS | NS | NS |
B | * | * | * | * | * | * | |
A × B | NS | NS | NS | NS | NS | NS |
The a* values (the red/greenness) (Wijethunga et al., 2023) on both sides of the fruits (Table 1) did not change significantly only in the 500 µL·L-1 chitosan post-harvest treated fruits and on the back side of the 100 µL·L-1 chitosan preharvest chitosan treated fruits throughout the storage period. Here, the values were increased slightly in the chitosan 100 µL·L-1 preharvest treatment, and a comparative increment was found in both MAP treatments. Apart from these outcomes, the a* color values of both sides of the 500 µL·L-1 chitosan preharvest treated mangoes showed a slight decrement during the storage period at room temperature.
With regard to the b* color values (the yellow/ blueness) (Wijethunga et al., 2023) (Table 1), both sides of the 150,000 cc MAP-treated mangoes showed significant differences during the storage period. Moreover, the chitosan 100 µL·L-1 preharvest treatment and the 500 µL·L-1 post-harvest treatment showed significant differences between the DAH values when stored at 20°C at the front side, while the control, the 500 µL·L-1 chitosan preharvest treatment, and the 80,000 cc MAP treatment showed a significant difference in their values on the back sides (Table 1).
Changes in the respiration rate
The respiration rate changes of mangoes under room-temperature storage are depicted in Fig. 7. The CO2 concentration did not change considerably in the 100 µL·L-1 chitosan pre-harvest treatment, while the control and the chitosan 500 µL·L-1 preharvest treatment showed decreases during the first six days of storage. All three treatments varied similarly until the last day of storage. A significant difference among the treatments was observed only at the beginning of the storage period (Fig. 7).
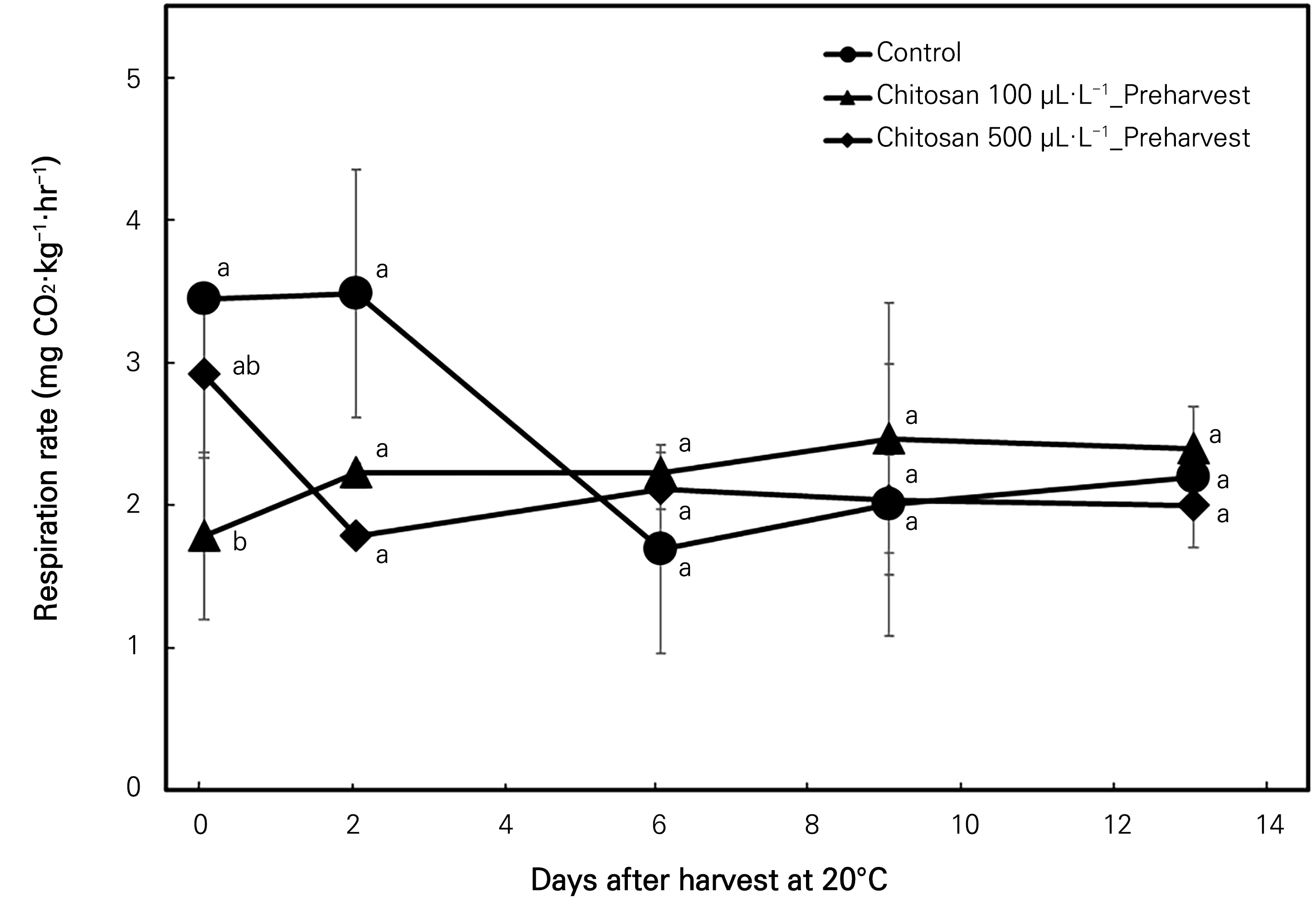
Fig. 7.
Effects of the preharvest chitosan application on the respiration rate (mg CO2·kg-1·hr-1) of ‘Irwin’ mango during room-temperature storage at 20°C for 13 days. Vertical bars indicate the standard error and means with different letters (a-c) indicate significant differences between the treatments at each sampling date according to Tukey’s test at p < 0.05.
Cold storage experiment
Changes in physicochemical properties
Changes in WL%
Subsequently, according to our second experiment, the weight of ‘Irwin’ mangoes kept in the cold storage at 7°C decreased steadily after being kept in room-temperature storage (Table 2). A considerable decrease was noted in the 500 µL·L-1 chitosan preharvest treatment, at 8.5% by the end of the storage period. A significant difference was found from each treatment among the DAS periods. Immediately after the cold storage period, the highest and lowest WL% values were obtained in the chitosan 500 µL·L-1 preharvest treatment and the control treatment at 2.5% and 1.8%, respectively. Moreover, the highest rate of WL% reduction was found in the control treatment compared to the preharvest chitosan treatments.
Changes in the SSC
As mentioned in Table 2, the SSC values showed no significant difference throughout the storage period for each treatment after the fruits were kept in cold storage at 7°C. On the other hand, the highest SSC values were obtained in the chitosan 500 µL·L-1 preharvest treatment, at 15.3°Brix. Thus, good flavor quality retention can be obtained if using the 500 µL·L-1 preharvest chitosan treatment compared to the other treatments.
Changes in the TA
The TA contents of mangoes remained relatively unchanged during room-temperature storage after cold storage at 7°C in all treatments (Table 2). In this case, a significant difference could not be identified in any of the treated mangoes during the storage period.
Changes in fruit firmness
The firmness changes of ‘Irwin’ mangoes during the days after cold storage condition are shown in Table 2. A significant difference between DAS values could not be identified in either chitosan-treated during the days after cold storage. The greatest firmness reduction was obtained in the control-treated fruits compared to the other two chitosan treatments. Nevertheless, the lowest value for firmness of DAS 14+0 was acquired from the 500 µL·L-1 chitosan preharvest treated fruits (3 N).
Table 2.
Effects of different chitosan preharvest treatments on the weight loss (WL), soluble solids content (SSC), titratable acidity (TA), firmness, and respiration rate of the ‘Irwin’ mango fruit in the days after cold storage at 7°C
Treatments (A) |
DAS (B) |
WL (%) |
SSC (ºBrix) |
TA (%) |
Firmness (N) |
Respiration rate (mg CO2·kg-1·hr-1) |
Control | 14+0 | 1.8 c | 13.0 a | 0.3 a | 4.3 a | 3.4 az |
14+2 | 3.9 b | 13.0 a | 0.3 a | 3.9 ab | 3.1 a | |
14+5 | 6.8 a | 12.8 a | 0.3 a | 0.3 b | 3.9 a | |
Chitosan 100 µL·L-1 -Preharvest | 14+0 | 2.4 c | 14.9 a | 0.3 a | 3.3 a | 2.9 a |
14+2 | 4.9 b | 14.2 a | 0.2 a | 3.5 a | 2.9 a | |
14+5 | 6.9 a | 13.1 a | 0.3 a | 0.3 a | 3.7 a | |
Chitosan 500 µL·L-1 -Preharvest | 14+0 | 2.5 c | 15.3 a | 0.3 a | 3.0 a | 2.6 a |
14+2 | 4.3 b | 14.5 a | 0.3 a | 2.9 a | 2.9 a | |
14+5 | 8.5 a | 14.2 a | 0.3 a | 0.3 a | 3.9 a | |
F-testy | A | * | * | NS | * | NS |
B | * | NS | NS | NS | * | |
A × B | * | NS | NS | NS | NS |
Changes in L*, a*, and b* color values
According to Table 3, a significant difference was not observed between the DAS values for each treatment for the front sides and back sides of the fruits. Nevertheless, comparing the L* color values of the front sides of all treated mangoes, the lowest values were obtained from the chitosan 500 µL·L-1 preharvest treated ‘Irwin’ mangoes in the days after cold storage, though this was not true with the back sides of the fruits.
In accordance with the a* values of the front and back sides of the mangoes kept under room-temperature storage after the cold storage period (Table 3), no significant differences in the DAS values were observed in all treatments. Nevertheless, the values on the back side of the 100 µL·L-1 chitosan pretreated fruits showed significant differences in DAS, and the a* color values fell dramatically with the storage period at 20°C.
Overall, there were no significant differences in the b* color values among the different storage days after cold storage for both sides of the treated and untreated fruits (Table 3). Considering the treatments, lower b* values were reported for the front sides of the 100 µL·L-1 and 500 µL·L-1 chitosan-pretreated fruits compared to those of the control treatment. However, for the back sides, somewhat similar values were obtained in the control, 100 µL·L-1 chitosan pretreated and 500 µL·L-1 pretreated mangoes.
Table 3.
Changes in the L*, a*, and b* color values for the front and back sides of ‘Irwin’ mangoes during the days after cold storage at 7°C in response to the different chitosan preharvest treatments
Treatments (A) |
DAS (B) | Color | |||||
L* | a* | b* | |||||
Front | Back | Front | Back | Front | Back | ||
Control | 14+0 | 42.1 a | 38.7 a | 24.5 a | 30.3 a | 25.7 a | 21.2 az |
14+2 | 41.1 a | 41.0 a | 21.6 a | 23.8 a | 23.6 a | 24.0 a | |
14+5 | 38.8 a | 38.6 a | 28.8 a | 26.2 a | 20.7 a | 20.1 a | |
Chitosan 100 µL L-1 -Preharvest | 14+0 | 38.5 a | 39.7 a | 27.1 a | 30.0 a | 20.0 a | 22.2 a |
14+2 | 41.4 a | 42.0 a | 29.3 a | 26.1 ab | 22.1 a | 25.4 a | |
14+5 | 43.4 a | 41.4 a | 21.3 a | 18.2 b | 26.3 a | 23.5 a | |
Chitosan 500 µL·L-1 -Preharvest | 14+0 | 36.8 a | 38.9 a | 28.1 a | 27.3 a | 19.5 a | 21.7 a |
14+2 | 37.3 a | 38.7 a | 26.2 a | 27.0 a | 20.6 a | 23.5 a | |
14+5 | 38.9 a | 37.3 a | 21.3 a | 26.9 a | 20.3 a | 19.0 a | |
F-testy | A | NS | NS | NS | NS | NS | NS |
B | NS | NS | NS | NS | NS | NS | |
A × B | NS | NS | NS | NS | NS | NS |
Changes in the respiration rate
The respiration rates were not significant in all treated mangoes during the storage period after the fruits were kept in cold storage at 7°C (Table 2). The highest and lowest respiration rates were found in the control and 500 µL·L-1 chitosan preharvest treatments on the first day (DAS 14+0) after they were kept in cold storage, at 3.4 and 2.6 mg CO2·kg-1·hr-1, respectively.
Discussion
The ‘Irwin’ mango is one of the most popular and economically important mango cultivars in South Korea. Its short post-harvest shelf life may be caused by the climacteric nature of the fruit, and improper post-harvest management hinders long-distance mango distribution and has led to considerable monetary losses due to rapid post-harvest losses (Parvin et al., 2023). The surrounding environmental conditions affect how the physicochemical properties change and affect the post-harvest shelf life of this fruit. On the other hand, these mango fruits are frequently distinguished by high perishability and a short post-harvest shelf life due to the tremendous metabolic activity following harvest. Chitosan edible coating and MAP applications are some of the most useful strategies for enhancing the postharvest fruit quality after the harvesting of mangoes. Therefore, identifying fruit characteristics after the fruit are exposed to different conditions is essential for improving the quality after harvest because they vary depending on the environmental conditions and the various treatments used.
Room-temperature storage experiment
Changes in physicochemical properties
Changes in WL%
Weight loss is a natural characteristic of horticultural crops during storage attributable to respiration, transpiration, and other biological changes that occur. An elevated rate of weight loss is linked to the undesirable appearance of fruit, which tends to wilt, with consumer acceptability then falling as well (Vilvert et al., 2022). Weight loss in mangoes is primarily affected by the composite treatments used and the storage duration and is also caused by moisture loss during storage (Minas et al., 2014; Wei et al., 2019). According to Vilvert et al. (2022), the minimum weight loss was found in ‘Palmer’ mangoes treated with polyethylene-based bags, followed by chitosan-based treatments and the control treatment. These observations are generally similar to the present findings for the fruit kept only under room-temperature storage at 20°C.
Polyethylene is a widely used polymer for foods, including fruits and vegetables, owing to its transparency, relatively low toxicity, flexibility, low cost, density, and high processability (Raj et al., 2005). On the other hand, due to its non-polarity and lack of biodegradability, it performs poorly as a barrier for gases (Mohamed et al., 2017; Vilvert et al., 2022). Owing to their superior barrier qualities against oxygen and carbon dioxide, many biopolymers, particularly polysaccharides such as chitosan, have been investigated for use as barrier materials (Vilvert et al., 2022). The present study used perforated polyethylene (PE) bags with tiny holes as MAPs. Hence, the WL% reduction of ‘Irwin’ mangoes here, after a treatment with both MAPs, was caused by the non-polarity nature of PE, which makes it a hydrophobic polymer, in contrast the polar characteristic of chitosan (Vilvert et al., 2022).
Changes in the SSC
The SSC is a crucial measure when assessing the nutritional value and storage quality of fruits that contain sugars, organic acids, and minerals (Wei et al., 2021b). The MAP-treated ‘Irwin’ mango fruits kept only under room-temperature storage show a comparative reduction of their SSC values compared to the other treatments, indicating the significant effects of these treatments on suppressing SSC during storage. According to previous studies (Wei et al., 2021a; Vilvert et al., 2022), the significant increments of the SSC contents in both MAP and coating treatments were comparatively suppressed by the MAP treatments during the storage period, and inconsistent with the present results.
Starch is almost entirely hydrolyzed into simple sugars, predominantly glucose, fructose, and sucrose, throughout the mango ripening process, increasing soluble solids content while imparting the fruit a sweet flavor (Vilvert et al., 2022). Controlling and maintaining the upward trend of the SSC is the goal of fruit storage (Wei et al., 2021b). However, the present findings indicate that this hydrolyzation process in ‘Irwin’ mango fruits was remarkably delayed in both MAPs under room-temperature storage conditions due to the low oxygen concentration that developed around the fruits, which reduced the process of the starch breaking down into glucose by inhibiting the amylase and maltase activities (Singh et al., 2003; Vilvert et al., 2022).
Changes in the TA
The primary metabolic substrates used in the post-harvest process are sugars and organic acids, and organic acids are preserved by varying factors, limiting the corresponding metabolic activities (Nia et al., 2021). The TA contains various organic acids, serving as substrates for the respiratory metabolism of fruits (Ibarra-Garza et al., 2015). TA usually decreases during storage, and the maximum decline denotes fruit senescence (Nia et al., 2021).
As previously mentioned, the chitosan edible coatings form a thin outer covering on the fruit surface, which effectively reduces gas exchange, the fruit respiration rate, and ethylene production (Castro et al., 2006; Abbasi et al., 2009; Hong et al., 2012). Therefore, the treatment, particularly post-harvest chitosan-coated mangoes under room-temperature storage, inhibits the metabolic process and delays the decrease in the TA. The findings of Vilvert et al. (2022) support these observations of the TA to some extent because the MAP-treated mangoes showed a significant retention of their TA contents, but the values in the current results are lower than those of chitosan post-harvest coating treatments.
Changes in the SSC/TA ratio
Fruit flavor is influenced primarily by the balance between the sugar and organic acid contents (Vilvert et al., 2022) and is accessed through the SSC/TA ratio. All MAP treatments here significantly reduced the increasing rates of variation in the SSC/TA ratio compared to the control treatment, meaning that the flavor quality of ‘Irwin’ mangoes packed with MAPs was retained at a high quality level. In addition, the highest flavor quality was obtained from the 100 µL·L-1 chitosan preharvest treatment given the highest SSC/TA ratio. A rapid decrease in this ratio for mango fruits during storage is correlated with quick fruit senescence (Abbasi et al., 2009). Hence, among all treatments, fruits that underwent the 500 µL·L-1 chitosan preharvest treatment reached senescence on the last day of the experiment. These results are in line with those obtained by Mohamed et al. (2017), Nia et al. (2021), and Vilvert et al. (2022).
Changes in fruit firmness
Firmness is a crucial criterion for assessing storability and a qualitative factor influencing consumer preferences and shelf life (Wei et al., 2021b). Firmness levels in the comparison among all treated and non-treated samples showed no significant differences during the room-temperature storage period. Hence, every treatment affecting homogeneity maintained fruit firmness under room-temperature storage. However, Wei et al. (2021b) reported contrasting findings, while Saikaew et al. (2023), and Srinivasa et al. (2002) reported a similar variation of fruit firmness changes in both chitosan-and MAP-treated mangoes.
Losses in the degree of the softening progress with the storage period due to mango ripening were observed due to the increases in the enzymatic activities of cell wall hydrolyzing enzymes, such as pectinase, pectin methyl esterase (PME), polygalacturonase, and pectate lyase (Mohamed et al., 2017; Byeon et al., 2022). The major polysaccharide found in the cell wall and mesocosm layer is pectin (Li et al., 2019b). Consequently, the protopectin is degraded by these enzymes during the post-ripening stage of mangoes, which facilitates the softening of the fruit and decreases firmness (Ibarra-Garza et al., 2015). Studies have shown that altering the environment within the bags suppresses the expression of some genes that code for the enzymes that break down the middle lamella and fruit cell walls (Cukrov et al., 2019). In this case, this modified atmosphere develops due to CO2 and O2 gas diffusion from the MAPs; the chitosan coatings maintained the normal metabolic activity, which delayed the textural changes and reduced the ripening process of the ‘Irwin’ mangoes.
Changes in the L*, a*, and b* color values
Fruit color is a very important trait in mangoes related to consumer perceptions when they purchase fruits (Perumal et al., 2021). Moreover, mango pigments contribute to the nutritional content of the fruits and are authentic as harvest indices given their connection to the fruit maturity stage (Nordey et al., 2014). Generally, in the ‘Irwin’ mango cultivar, the green color of immature mangoes becomes reddish-yellow as they mature, later turning a remarkable dark red color at the fully mature ready-to-eat stage (Wijethunga et al., 2023). In addition, the green color of immature mangoes tended to decrease, becoming reddish-yellow with chlorophyll degradation and the increased accumulation of anthocyanin and carotenoids during the fruit maturation and ripening stage (Khoo et al., 2011; Ma et al., 2018; Li et al., 2019a).
The identical red color of ‘Irwin’ mangoes can be caused by anthocyanin in the fruit skin. The color changes in fully ripened ‘Irwin’ mangoes can be delayed or retained by adopting low oxygen conditions, implemented by aforementioned treatments. This occurs due to the decrease in degrading enzymes such as chlorophyllase, as evidenced by the non-significant color values throughout storage periods under the room-temperature storage condition. Li et al. (2019a) reported that treatments with higher CO2 levels delayed the increase in the a* color values and the decrease in the b* values during the strawberry fruit preservation process, whereas the current changes in the color values in the chitosan coatings and MAP treatments under room-temperature storage conditions are consistent with previous findings.
Cold storage experiment
Changes in physicochemical properties
Changes in WL%
For the ‘Irwin’ mangoes kept in room-temperature storage after being kept in cold storage, the highest rate of WL% reduction was found in the control treatment as opposed to the preharvest chitosan treatments. Encouraging results were obtained by Mohamed et al. (2017) for mango cv. ‘Zibda’. Additionally, previous studies of peaches (Gayed et al., 2017) and table grapes (Nia et al., 2021) are in good agreement with the present findings. The chitosan coating creates a layer of a semi-transparent, smooth pericarp surface that serves as a protective barrier to reduce respiration and transpiration rates via the fruit surface (Zhu et al., 2008; Mohamed et al., 2017). In the current study, the low-temperature storage environment could more effectively hinder metabolic activities by reducing the dissipation of moisture and nutrients. In addition, the chitosan edible coating treatment applied to mango fruits effectively serves as a physical barrier against moisture loss (Wei et al., 2021b). These factors are likely the main reasons for the suppressed weight loss reduction during cold storage and afterward.
Changes in the SSC
As reported elsewhere (Gayed et al., 2017; Mohamed et al., 2017; Kumarihami et al., 2020; Nia et al., 2021), the SSC contents of all treatments decreased dramatically throughout the storage period. Among them, the chitosan preharvest treatments overcame the increase in the SSC contents. Therefore, the current results obtained from the room-temperature storage condition after the fruit were kept in cold storage stand in contrast to these findings.
Nevertheless, as observed after cold storage at 7°C, each treated fruits retained SSC throughout the storage period which is evident by the non-significant values. The effects of the coating treatments with regard to sustaining the accumulation of SSC was most likely due to the semipermeable membrane applied around the fruit, which adjusted the interior atmosphere by lowering oxygen and increasing the carbon dioxide to slow down the respiration and the metabolic activities (Singh et al., 2007; Yu et al., 2012; Mohamed et al., 2017).
Changes in the TA
In this study, the observations of the period after cold-temperature storage in all treatments had a similar effect on their TA changes. This could be attributed to the fact that the films produced by the 100 µL·L-1 and 500 µL·L-1 chitosan preharvest treatments were more homogeneous and had superior properties in terms of preventing oxygen penetration, thus inhibiting the respiration process and lowering the nutrient consumption of mangoes. Considering the cold-temperature storage at 7°C, these results contrast with those of Chitarra et al. (2001), Cosme Silva et al. (2017) and Mohamed et al. (2017) for mangoes; Kumarihami et al. (2020) for Kiwi fruits; Nia et al. (2021) for table grapes; and El-Shazly et al. (2013) and Gayed et al. (2017) for peaches.
Changes in fruit firmness
Considering the firmness values of fruits obtained in the days after cold storage, although a comparative firmness loss was reported after the 500 µL·L-1 chitosan preharvest treatment, a significant reduction was obtained in the control samples during the room-temperature storage period. Some earlier findings were in harmony with those reported by Cosme Silva et al. (2017), Mohamed et al. (2017), and Kumar et al. (2020), as the firmness values of the chitosan-treated fruits decreased dramatically with the storage period and were retained compared to the control treatments. Firmness loss in mangoes is mainly caused by the activation of the aforementioned cell-wall-degrading enzymes upon fruit ripening (Mohamed et al., 2017; Vilvert et al., 2022). Furthermore, it is affected by the diffusion of water via the stomata (Kumarihami et al., 2020). However, the barrier properties imparted by the preharvest chitosan treatments effectively reduced the respiration rate and water loss through cell wall by modifying the atmosphere surrounding the fruit surface, thereby significantly inhibiting the firmness reduction during the fruit ripening process compared to non-treated fruits (Reddy et al., 2000; Kumarihami et al., 2020).
Changes in L*, a*, and b* color values
The chitosan edible coating forms a partial barrier around the fruit, altering its internal environment by reducing oxygen and increasing carbon dioxide levels (Medeiros et al., 2012). The elevated carbon dioxide hampers fruit ripening by hindering the production of ethylene (Ali et al., 2011). This delay in ripening and aging slows down the color transformation step by lowering carotenoid production and preserving the chlorophyll content by inhibiting the chlorophyll-degrading enzymatic activity (Mohamed et al., 2017).
However, the ‘Irwin’ mangoes kept under cold storage condition at 7°C did not show any significant difference in their color values after they were exposed to the simulated room-temperature condition. However, these non-significant color changes during storage may have stemmed from the use of fully ripe ‘Irwin’ mangoes with same appearance for all treatments. Furthermore, the reason behind the non-significant variation among the control and pre-harvest treated fruits could be the homogeneity of all treatments in terms of the significant suppression of the respiration rate and chlorophyllase activity. However, findings reported by Mohamed et al. (2017) contrast with the present findings during the days after cold storage. In addition, the control-treated mangoes kept in cold storage exhibited black patches on the skin after the fifth day of room-temperature storage, and all treated mangoes were completely spoiled afterward.
Changes in the respiration rate
The results obtained from both conditions demonstrate the selective permeability to respiratory gases (O2 and CO2) by the chitosan treatment. The reduced respiration rates in a modified atmosphere caused by the effective inhibition of respiratory gas exchange produced by the semipermeable chitosan layer over the ‘Irwin’ mangoes indicates that the treatments increased the quality of the mangoes and hindered fruit metabolism (Wei et al., 2021b). Nonetheless, in this study, the effects of the pre-harvest chitosan coating treatment on the respiration rate during both the post-harvest room-temperature and cold-temperature storage periods were not significant, indicating a residual effect of pre-harvest chitosan on the post-harvest life of ‘Irwin’ mangoes.
Conclusion
Although numerous studies have investigated the efficiency of chitosan and MAP treatments, there is scant research on the evaluation of the effectiveness of both chitosan preharvest application and MAPs for preserving fruit quality and controlling post-harvest decay of fully ripe ‘Irwin’ mangoes under different storage conditions. Owing to the climacteric nature of mango fruit, rapid quality deterioration under adverse conditions shortens the post-harvest shelf life. The present study investigated the effects of both chitosan coating and MAP films under room-temperature storage. On the other hand, the influence of pre-harvest chitosan application under cold storage conditions was also evaluated. Here, our objective was to determine the best strategy for enhancing the overall fruit quality of fully ripe ‘Irwin’ mangoes in accordance with specific storage conditions. Evidently, MAPs at room temperature positively affected quality retention and weight maintenance compared to the chitosan treatments. Under the other condition, the physicochemical characteristics were retained during cold storage, and the WL% was changed moderately after the fruits were exposed to room-temperature condition. The changes in the SSC, TA, and respiration rates were not significant, and the firmness decreased significantly only in the control treatment in the days after cold storage. Considering the chromaticity, an apparent variation in every color value between the front and back sides of the fruit was not observed. Overall, MAPs in room-temperature storage and preharvest chitosan treatments in the days after cold storage were the most effective strategies for improving the post-harvest quality and prolonging the shelf life of ‘Irwin’ mango fruits. Hence, this study highlights the potential to hinder post-harvest losses during long-distance transportation and under different onsite storage conditions, eventually enhancing consumer preference and profits for ‘Irwin’ mangoes on a commercial scale.