Introduction
Materials and Methods
Plant Materials and Growth Conditions
Air Anion Treatments
Plant Growth Characteristics
Photosynthetic Parameters
Mineral Content
Statistical Analysis
Results
Plant Growth Characteristics
Photosynthetic Parameters
Mineral Content
Discussion
Air Anion Concentration
Growth Characteristics
Photosynthetic Parameters
Mineral Content
Conclusions
Introduction
Air anions are molecules or atoms charged with an electron in the atmosphere (Jiang et al., 2018). These anions form in natural environments under the following conditions: (1) when the surrounding air is ionized in the presence of an electric field intensity above a critical point, such as during an electrical storm with lightning discharges (a lightning environment); (2) when water molecules split and collide with the ground under a waterfall, a large amount of negative electric charge is generated, which charges oxygen molecules or dust in the air to form air anions in the atmosphere; (3) when anions are generated by radiation derived from radioactive elements, such as uranium and radium; and (4) when anions are generated by the direct ionization of air molecules by ultraviolet radiation (Borra et al., 1997; Harrison and Carslaw, 2003; Iwama et al., 2004; Jiang et al., 2018). The effect of air anions on living organisms has mainly been studied in animals and includes relieving stress by reducing the level of serotonin, a neurohormone that regulates sleep and mood (Krueger and Reed, 1976; Bailey et al., 2018). A study of plant responses to air anions in the 1960s reported that air anions promoted photosynthesis and increased the transpiration rate of barley and oats (Krueger et al., 1962, 1964). In addition, recent studies on plants have shown a positive effect of air anions on indoor fine dust because they easily adhere to the leaves by electrical condensation and are then precipitated into the atmosphere as fine dust (Tanaka and Zhang, 1996; Grabarczyk, 2001).
In our previous research, we showed that air anion treatment increased the photosynthesis rate, transpiration rate, and stomatal conductance and led to the promotion of growth characteristics of kale and lettuce plants in a plant factory with artificial lighting (PFAL) (Song et al., 2014; Lee et al., 2015). These results demonstrate that air anions are effective in promoting plant growth in an enclosed environment and suggest the possibility of applying air anions in other enclosed environments, such as greenhouses with environmental control. Recently, the environmental conditions (temperature, humidity, CO2 concentration, light quantity, wind speed, etc.) in greenhouses have been monitored by information and communications technologies (ICT) in real time to actively create suitable environmental conditions for crop growth. In this respect, air anion treatment in greenhouses may create a synergistic effect on increasing plant production.
Spinach (Spinacia oleracea) belongs to the goosefoot family and is a perennial plant native to southwest Asia. Spinach is widely used as a salad with increasing market demand because it is rich in vitamins A, B, and C. It is classified as a superfood that contains calcium, potassium, and iron (Palaniswamy, 2003; Al-Qumboz and Abu-Naser, 2019). Spinach is a cool season crop that develops floral differentiation under high temperatures and long-day conditions; in Korea, it stops growing at high temperatures and humidity and, in severe cases, when there are pests and physiological disorders (Naiki and Kanoh, 1978; Lim et al., 1990; Woo et al., 1997). There is a need to improve cultivation techniques to increase spinach production and reduce the cultivation period in spring and fall. Therefore, the purpose of this study was to verify whether air anion treatment in a greenhouse can induce growth promotion of spinach.
Materials and Methods
Plant Materials and Growth Conditions
Spinach seeds (‘Mustang’, FarmHannong, Seoul, Korea) were sown in a tray (32 mL per cell) containing horticultural growing medium (Baroker, Seoul Bio, Eumseong, Korea) (two seeds per cell) and placed in a growth chamber (DS-12BPLH; Dasol Scientific, Hwaseong, Korea) under the following growing conditions: average temperature 23°C, relative humidity 60%, light intensity 130 µmol·m-2·s-1, and a 16-h photoperiod. For seed moisture management, 1 L of distilled water was sub-irrigated every 2 days. Fourteen days after sowing, the spinach seedlings were individually transplanted into square plastic pots (10.6 × 10.6 × 11.5 cm, L × W × H) and cultivated in a greenhouse. There were 48 spinach plants in the control or each treatment. Hoagland solution (Hoagland and Arnon, 1950) (pH 5.8, EC 1.0 dS·m-1) was supplied to four trays (30 × 20 × 11.5 cm, L × W × H) with four pots per treatment every day at a volume of 1 L per tray. In case there was a large amount of evaporation due to increasing temperatures, the nutrient solution was supplemented to a height of 1 cm from the bottom of the pot to prevent drought stress. To minimize the experimental error caused by the unequal distribution of the growth environment conditions, including air anion concentration, light intensity, and temperature, the location of the pots was regularly rotated every day. The temperature inside the greenhouse was controlled using an automation module, and the side window was opened at temperatures above 18°C and closed at temperatures below 15°C. This experiment was conducted twice in the spring (March 15-May 16) and fall (September 20-November 4) of 2019. The average temperature and relative humidity in the greenhouse were 20.0°C and 55.3%, respectively, in spring and 19.6°C and 65.3%, respectively, in fall. The average daily light integral (DLI) inside the greenhouse in spring and fall was 58.5 and 37.0 mol·m-2·d-1, respectively.
Air Anion Treatments
After transplanting, the spinach plants were treated with different levels of air anions for 27 days. Four air anion generators (EGT1A2-2; EGtech, Incheon, Korea) were installed per unit cultivation area (42 × 42 cm) with heights from the upper part of the plant set at 67, 42, and 27 cm to generate three levels of air anions: low (LA) 3.4 × 105 ion/cm3, moderate (MA) 5.2 × 105 ion/cm3, and high (HA) 8.3 × 105 ion/cm3 (Fig. 1). For the control, the air anion generator was not installed above the plant, and the air anion concentration was 8.7 × 10 ion/cm3. The plants were exposed to the air anions for 12 hours a day during the daytime. The horizontal distance between the treatment sections was 180 cm to minimize interference by neighboring air anion treatments. The concentration of air anions was measured at nine points in the cultivation area using an air anion meter (COM-3600F, Com System, Akishima-City, Tokyo, Japan) for 5 min.
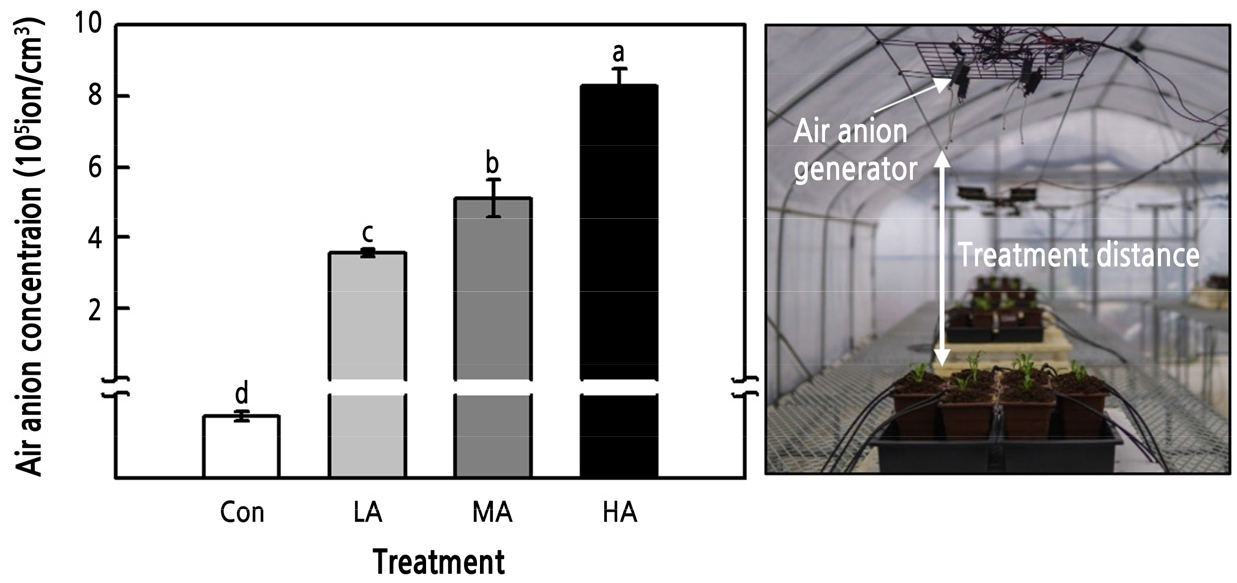
Fig. 1.
Air anion concentrations generated by air anion generators (A), and a picture of the experimental site in a greenhouse (B). Con, LA, MA, and HA indicate control, low, moderate, and high air anion treatments, respectively. The air anion concentration in the control treatment was 8.7 × 10 ion/cm3. Different letters indicate significant differences at p < 0.05 (n = 9).
Plant Growth Characteristics
To determine changes in growth following air anion treatments, spinach plants were collected to measure growth characteristics after 27 days of treatment. The fresh weights of the shoots and roots were measured using electronic scales (SI-234; Denver Instruments, Denver, CO, USA). Then, the shoot and root dry weights were measured after drying them in a constant-temperature dryer at 70°C (VS-1202D3, Vision, Daejeon, Korea) for more than 72 h. The total leaf area was measured using a leaf area meter (Li-3100C; Li-COR, Lincoln, NE, USA).
Photosynthetic Parameters
The photosynthetic rate, transpiration rate, and stomatal conductance of the spinach were measured using a portable photosynthetic meter (Li-6400 XT; Li-COR, Lincoln, NE, USA) at 15 and 24 days of treatment. Measurements were made for 4 h from 8 a.m. to noon. During the measurement, the photosynthetic photon flux density (PPFD), CO2 levels, relative humidity, and leaf temperature within a leaf cuvette were maintained at 400 µmol·m-2·s-1, 400 µmol·mol-1, 70 ± 5%, and 23°C, respectively, which is similar to the growth conditions of the greenhouse. Measurements were made using the third main leaf of the spinach for each treatment, and data for 5 min were obtained from six replications per treatment.
Mineral Content
Mineral contents in spinach shoot were assessed by modifying the method reported by Lee et al. (2015). Shoots were dried at 70°C in a constant-temperature dryer and were then ground to a powder using a mixer (MFM-002H, HiBell, Hwaseong, Korea). Approximately 1.0 g of the powdered sample was placed in a Teflon beaker (CW.007.250, Cowie, UK) with 15 mL of 70% nitric acid (N1056, Samjeon Chemical, Pyeongtaek, Korea), covered with a Teflon watch glass (CW.013.100, Cowie, UK), and reacted overnight in a hood. Subsequently, the samples were placed on a heating block (Ecopre 2, ODlab, Gwangmyeong, Korea), and the temperature was gradually raised to 125°C and heated for 1 h and 30 min. After cooling for 10 min, 7.5 mL of 30% hydrogen peroxide (H0297, Samjeon Chemical, Pyeongtaek, Korea) was added, and the samples were heated again at 125°C for 1 h, and the same process was repeated once. Finally, once the sample was completely decomposed without any residue, the mixture was heated at 200°C for 1 h and 30 min and then sufficiently cooled. Then, 45 mL of 2% nitric acid was added to the completely decomposed sample, and the mixture was allowed to react for 3 h. The sample solution was weighed (SI-234; Denver Instruments, Denver, CO, USA) and filled to 80 g using tertiary distilled water, and then the solution was filtered through quantitative filter paper (TO5B-110, Advantec, Tokyo, Japan). The contents of P, K, Ca, Mg, S, Fe, Cu, Zn, Mn, and B were analyzed using inductively coupled plasma spectroscopy (OPTIMA 7300 DV, Perkin Elmer, Waltham, MA, USA).
Statistical Analysis
This experiment was designed using a randomized complete block design in three independent greenhouses. Nine plants per treatment were collected to determine the growth characteristics, while six plants were used to measure the photosynthetic parameters and mineral contents. The data were statistically analyzed using analysis of variance in the Statistical Analysis System (SAS version 9.4, SAS Institute, Cary, NC, USA). To determine significant differences of the mean value for each treatment, Tukey's honest significant difference (HSD) test was used.
Results
Plant Growth Characteristics
After 27 days of air anion treatment in spring, the fresh weights of the shoots and roots of spinach in the HA group were 26% and 33% higher than those in the control group, respectively (Fig. 2A and 2C). Meanwhile, there were no significant differences in the shoot and root dry weights among the treatment and control groups, although the results showed a similar trend regarding the shoot and root fresh weights (Fig. 2B ans 2D). During fall, shoot and root fresh and dry weights markedly decreased by 341% compared to those in spring, and the values in treatment groups were almost 50.2% higher than in the control group. In addition, the total leaf area of spinach in spring tended to increase as the concentration of air anions increased, and this value was significantly higher in the HA treatment than in the control by 33% (Fig. 3A). During fall, the total leaf area was significantly higher in all air anion treatment groups by at least 50% than in the control group. The effect of air anions on spinach growth was visually confirmed in the HA group (Fig. 3B).
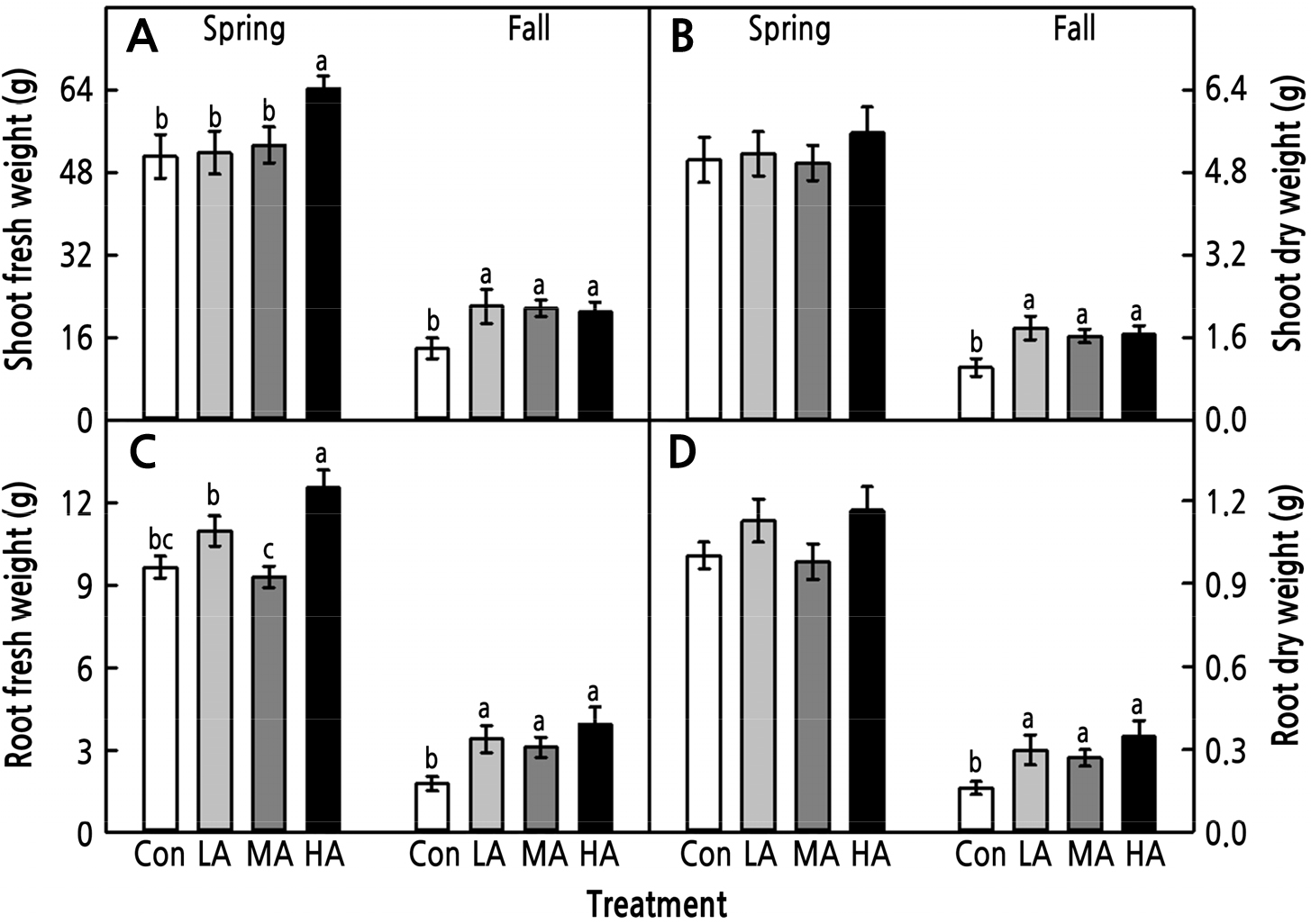
Fig. 2.
Shoot fresh weight (A), dry weight (B), root fresh weight (C), and dry weight (D) of spinach plants grown under air anion treatments for 27 days in the spring and fall seasons of 2019. Con, LA, MA, and HA indicate control, low, moderate, and high air anion treatments, respectively. Different letters indicate significant differences at p < 0.05 (n = 9).
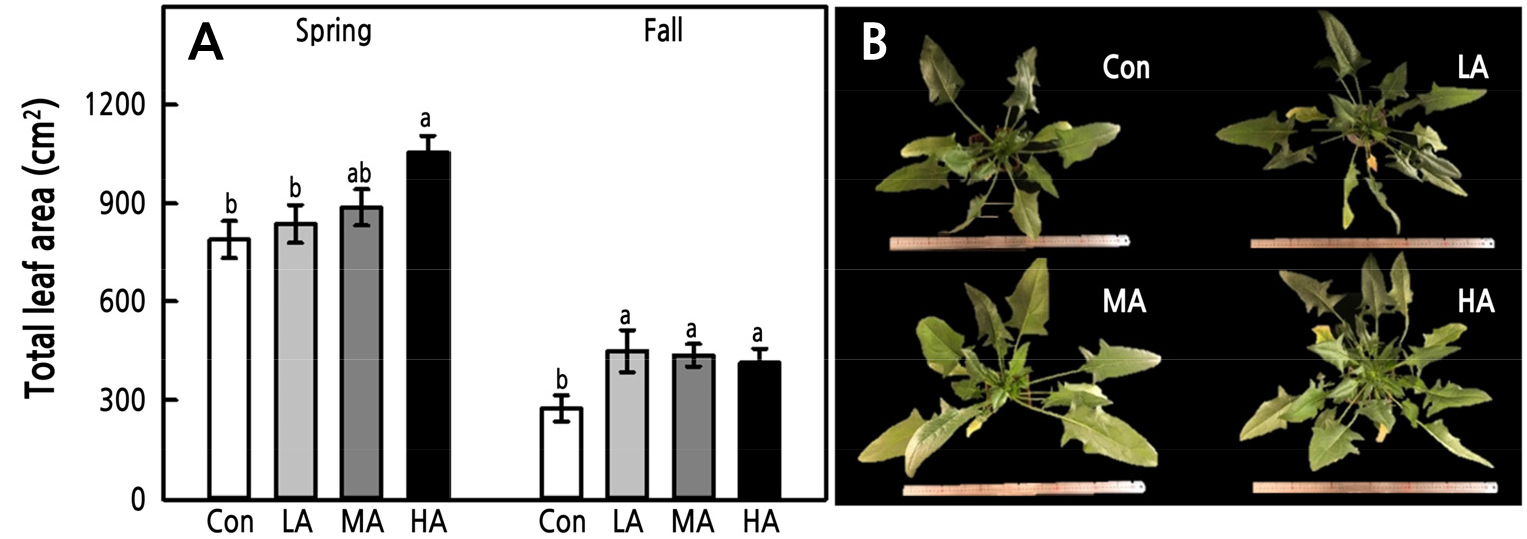
Fig. 3.
Total leaf area of spinach plants grown under air anion treatments for 27 days in spring and fall (A), and spinach plants grown under air anion treatments for 27 days in the spring of 2019 (B). Con, LA, MA, and HA indicate control, low, moderate, and high air anion treatments, respectively. Different letters indicate significant differences at p < 0.05 (n = 9).
Photosynthetic Parameters
At 15 and 24 days of air anion treatment during spring and fall, the photosynthetic rate, transpiration rate, and stomatal conductance were measured (Fig. 4). During spring, the photosynthesis rate was not significantly different among all treatments on both days 15 and 24, whereas the transpiration rate in all treatments groups at day 15 was at least 1.6 times higher than that in the control group; at day 24, this value in the LA and HA groups was significantly higher by 1.5 and 1.7 times, respectively. In particular, it was the highest in the HA group at day 24. The stomatal conductance at 15 and 24 days was significantly higher in all air anion treatment groups than in the control group by at least 1.4 and 1.3 times, respectively.
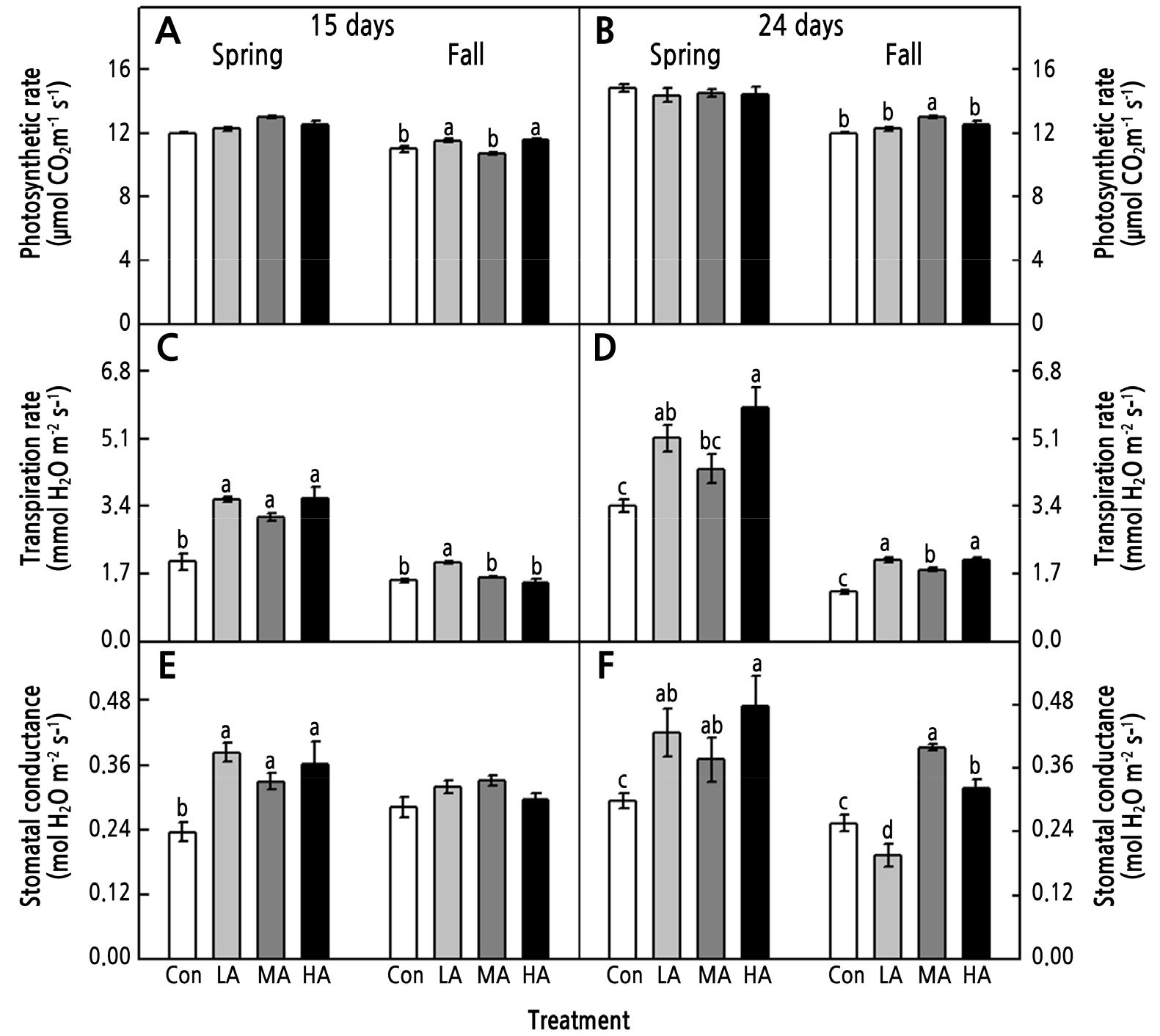
Fig. 4.
Photosynthetic rate (A and B), transpiration rate (C and D), and stomatal conductance (E and F) of spinach plants grown under air anion treatments for 15 days (A, C, and E) and 24 days (B, D, and F) in the spring and fall seasons of 2019. Con, LA, MA, and HA indicate control, low, moderate, and high air anion treatments, respectively. Different letters indicate significant differences at p < 0.05 (n = 6).
In fall, the photosynthetic rate at day 15 was 5% higher in the LA and HA groups than in the control group, whereas the photosynthesis rate in the MA group on day 24 was 8% higher than that in other treatment and control groups. The transpiration rate at day 15 was significantly higher (29%) in the LA group than that in the control group, and at 24 days, this rate in all air anion treatment groups was approximately 45% higher than that in the control group. Stomatal conductance was not significantly different in all treatment groups at day 15 but was higher than the control group in the MA and HA groups at day 24 by 56% and 25%, respectively.
Mineral Content
The mineral contents of spinach shoots in spring and fall are shown in Table 1. The macro- and micro-elements in spinach tended to increase as the level of air anions increased in spring. The highest mineral content was noted in the HA group, but there were no statistically significant differences in any element contents among the treatments. During fall, the mineral content in the LA group was at least 130% higher than in the control group; in particular, Fe content was 198% higher, which was the highest rate of increase.
Table 1.
Mineral contents of spinach shoots subjected to air anion treatments for 27 days in the spring and fall seasons of 2019
Season (2019) | Treatmentz | Macronutrient (mg/g plant DW) | Micronutrient (µg/g plant DW) | |||||||||
P | K | Ca | Mg | S | Fe | Cu | Zn | Mn | B | |||
Spring | Con | 23.4 NSy | 425.9 NS | 44.9 NS | 43.6 NS | 18.1 NS | 423.2 NS | 30.2 NS | 252.2 NS | 815.6 NS | 232.5 NS | |
LA | 24.1 NS | 426.4 NS | 47.8 NS | 43.2 NS | 18.6 NS | 444.9 NS | 29.8 NS | 260.1 NS | 953.0 NS | 248.6 NS | ||
MA | 22.9 NS | 448.6 NS | 44.8 NS | 45.4 NS | 20.0 NS | 462.2 NS | 31.5 NS | 274.5 NS | 774.1 NS | 223.9 NS | ||
HA | 29.9 NS | 544. NS | 54.1 NS | 55.0 NS | 24.1 NS | 524.1 NS | 35.6 NS | 293.7 NS | 994.0 NS | 267.0 NS | ||
Fall | Con | 6.0 b | 99.9 b | 5.7 b | 14.8 b | 3.2 b | 57.7 b | 3.3 b | 47.9 b | 81.2 b | 23.8 b | |
LA | 14.7 a | 229.8 a | 13.4 a | 35.6 a | 8.2 a | 172.1 a | 9.3 a | 138.1 a | 213.2 a | 64.2 a | ||
MA | 11.6 ab | 194.5 ab | 11.9 ab | 30.1 ab | 6.8 ab | 151.8 a | 7.4 ab | 102.9 ab | 170.1 ab | 49.6 ab | ||
HA | 11.7 ab | 186.0 ab | 11.8 ab | 27.7 ab | 6.5 ab | 133.9 ab | 7.6 ab | 102.4 ab | 163.2 ab | 49.5 ab | ||
Season (A) | *** | *** | *** | *** | * | *** | *** | *** | *** | *** | ||
Treatment (B) | NS | NS | NS | NS | NS | NS | NS | NS | NS | NS | ||
(A) × (B) | NS | NS | NS | NS | NS | NS | NS | NS | NS | NS |
Discussion
Air Anion Concentration
Song et al. (2014) reported that the concentration of air anions is inversely proportional to the square of the distance between the anion generator and the plant. Thus, the concentration of air anions can be easily increased by reducing the distance, but the deviation in concentration is large depending on the location of the plant. When setting the same level of air anion concentrations, as in our experiment, a single air anion generator was installed almost near the plant cultivation area. In this experiment, four air anion generators were installed separately. As a result, the anion concentration deviation in this experiment could be reduced by 5.3 times than that of Song et al. (2014) (data not shown). Therefore, in our experiment, a more uniform and accurate air anion treatment was possible than in the previous study (Fig. 1). However, to use this treatment for plant cultivation in a large-scale facility, it is necessary to develop a device that can more effectively treat the area with uniform air anions.
Growth Characteristics
The effect of air anions on plant growth was previously reported. According to previous studies (Song et al., 2014; Won et al., 2018), lettuce grown under anions of 1.9 × 105 ion/cm3 and 7.1 × 105 ion/cm3 in PFAL increased the fresh and dry weights of shoots and the leaf area by at least 32% compared to the control. Lee et al. (2015) also reported that the fresh and dry weights of kale shoots grown in PFAL with air anions of 5.4 × 105 ion/cm3 increased significantly by at least 40% compared to the control because of the increase in leaf area and the number of leaves. Similar results were confirmed in this experiment using air anions in a greenhouse. In the HA treatment, the fresh weight and leaf area of the shoots and roots increased by at least 26% compared to the control in spring, and in fall the shoot and root weights increased in the air anion treatments by at least 50% compared to the control group (Fig. 2).
According to Borra et al. (1997), an electrostatic force is generated by an electric bottleneck caused by high voltage applied to the pointed part of the brush of the air anion generator. At this time, electrons (e-) emitted from the air anion generator are absorbed by gas molecules (oxygen or carbon dioxide) in the atmosphere to form air anions. The generation of air anions by electrostatic forces under natural conditions is an example of a lightning environment, as described in the Introduction. Studies of environments where lightning is produced have confirmed large amounts of air anions in the atmosphere, which results in the formation of electric fields (Standler and Winn, 1979). Therefore, in our experiment, it was judged that air anion treatment using the electron emission method on the space above the plant induced the formation of a higher electric field than in natural conditions. The positive effect of the electric field was confirmed by the increase in corn and kidney bean production (Murr et al., 1965, 1966). An external electric field around plants may be involved in the synthesis and distribution of auxin in the plants (Pohl and Todd, 1981; Smith and Fuller, 1961), which plays an important role in plant growth by inducing cell expansion and division (William et al., 2006).
Plants responded differently to the concentration of air anions depending on the season. In spring, the higher concentration (HA) of air anions significantly increased plant growth than other concentrations, whereas in the fall, no significant difference in the shoot and root fresh and dry weights was observed in all air anion treatments, which might be explained by the interaction of air anions, growth stage, and other environmental factors in the greenhouse. The shoot fresh weight of spinach in spring was more than 3.4 times higher than in fall owing to the difference in day length. When lettuce was cultivated under different DLI conditions between 1 and 14, the higher the DLI value was, the greater the dry weight was (by at least 20%) (Gent, 2014). In fact, the DLI in spring was 58% higher than in fall during the experimental period. The difference in environmental conditions could have altered the effects of air anion levels on plant growth. For example, when atmospheric humidity is high, it decreases the concentration of air anions in the atmosphere because the air anions are charged with water molecules and are eliminated (Horrak et al., 1998; Wu et al., 2006). In our experiment, the average relative humidity during the day in fall was 13.6% higher than in spring; therefore, our results demonstrated a correlation that was opposite to that between air anions and relative humidity described by Wu et al. (2006). Therefore, further investigations of the interaction between air anions and environmental conditions, such as relative humidity, are required.
Photosynthetic Parameters
The photosynthetic rate was significantly higher in the LA and HA groups on day 15 in fall than in the control group, and on day 24, the value in the MA group was significantly higher than that in the control group, which supported the effect of air anions on growth promotion (Fig. 4A and 4B). In contrast, the photosynthetic rate in spring was not significantly different among treatments, but both the stomatal conductance and transpiration rate in the air anion treatment groups were at least 1.3 times higher than in the control group (Fig. 4). The photosynthesis rate, transpiration rate, and stomatal conductance were measured per unit area of spinach leaves. Therefore, although the measured photosynthetic rate did not significantly change, the increase in leaf area in the air anion treatment groups during both seasons would indicate a positive effect on spinach growth by increasing the photosynthetic rate of the whole plant (Fig. 3).
The increase in stomatal conductance can enhance intercellular CO2 concentration, which can promote photosynthesis (Campbell et al., 1988; Huxman and Monson, 2003; Zhu et al, 2020). Our results are consistent with previous studies that showed an increase in the photosynthetic rate, transpiration rate, and stomatal conductance of lettuce, kale, and barley with air anion treatment (Kotaka et al., 1968; Sidaway and Asprey, 1968; Song et al., 2014; Lee et al., 2015). The electric field formed by air anions in this study migrated cations in leaves toward the negative electrode (Robinson, 1985), and the accumulation of K in both roots and leaves was occurred by electrical stimulation (Black et al., 1971; Lee and Oh, 2021), which may be closely related to stomatal opening and photosynthesis (Taiz and Zeiger, 2006).
Mineral Content
The mineral content of the spinach shoots after the LA treatment was at least 130% higher than that of the control in the fall (Table 1). The mineral uptake rate varies with cultivation season because of seasonal changes in environmental conditions, including light conditions such as light intensity and daylength (Horrak et al., 1998; Wu et al., 2006; Gent, 2014). Although there were differences in the effect of air anions on improving mineral content by season, it was generally positive. Lee et al. (2015) reported that macro-elements, such as P, K, Ca, Mg, and S, and micro-elements, such as Fe, Mn, and Zn, increased under air anion treatment, which was consistent with our results. The uptake of macro-elements (N, P, K, Ca, and Mg) increased in corn and tomatoes when treated with an electric field generated by an AC power supply connected to an aluminum plate (Murr, 1965, 1966; Black et al., 1971).
Air anion treatment improved the root growth and transpiration rate of spinach (Figs. 2 and 4). These increases demonstrated that the water-ionized minerals were more absorbed through the roots per unit of time. Low-mobility minerals, such as Ca, S, Fe, B, and Cu, also increased, so it is possible that they could be easily moved along the vascular tissue. Based on the results of our study, it is expected that air anion treatment may protect plants from physiological disorders, such as tip burn due to Ca deficiency, although further studies need to be conducted to explore the mechanism of mineral absorption by electric field.
Conclusions
In this study, we confirmed the applicability of air anions in a greenhouse. The increased level of air anions improved the photosynthetic parameters and subsequently promoted spinach growth in the two growing seasons. In addition, air anions also improved the absorption rate of the nutrient solution, which enhanced mineral contents in spinach plants. However, to develop these results into more practical and reproducible technologies, further studies are required for conducting molecular physiological analysis of plants treated with air anions. In addition, it is necessary to develop air anion generators that can process more uniform concentrations of air anions in a greenhouse.