Introduction
Materials and Methods
Plant Materials
Measurement of Absolute Fruit Growth
Soluble Sugars and Starch Analysis
Determination of Activities of Sucrose Metabolism-Related Enzymes
Statistical Analysis
Results and Discussion
Morphological Changes in Three Asian Pear Cultivars During Fruit Development and Ripening
Concentrations of Soluble Sugars and Starch in Three Asian Pear Cultivars During Fruit Development and Ripening
Activities of Key Enzymes Related to Sugar Metabolism in Three Asian Pear Cultivars During Fruit Development and Ripening
Heatmap Analysis of Relative Abundance of Sugar Metabolites and Related Enzyme Activities According to Fruit Maturity in Three Asian Pear Cultivars
PCA and Pearson Correlation Analysis of Parameters of Fruit Growth, Metabolites, and Enzyme Activity
Conclusions
Introduction
Pears are divided into two major groups: European and Asian pears. In contrast to European pears, Asian pears are harvested after ripening on the tree; thus, the fruit is eaten immediately after harvest. The Asian pear (Pyrus pyrifolia Nakai) is one of the most abundantly produced fruits in Korea and is popular because of its sweetness and juiciness. Asian pears are classified as early-, mid-, and late-maturing according to harvest time and present different texture attributes, storage ability, and physiochemical properties, such as firmness, acidity, and total soluble solids. For example, the late-maturing cultivars characteristically have large fruit that are slow to soften and have high storability, whereas the fruit of early-maturing cultivars are small and firm and have low acidity (Byun et al., 2003; Oanh et al., 2012). In addition, the harvest time depends on changes in fruit skin color; i.e., green to yellowish green or brownish green to brown. However, our current understanding of the mechanisms controlling fruit production and quality in Asian pears of different harvest maturity remains quite limited.
Sucrose is the main form of carbohydrate transported through phloem from the source (photosynthesizing leaves) to the sink (non-photosynthetic tissues; i.e., fruit, flower, seed) (Ruan et al., 2010). Sucrose is hydrolyzed into glucose and fructose by cell wall invertase (CWINV), neutral invertase (NINV), and vacuolar invertase (VINV) to be used as nutrients, an energy source, and signaling molecules for fruit set, growth, and ripening. Sugar composition and concentration are closely associated with qualities such as fruit taste and flavor, which depend on genotype/cultivar variation (Colaric et al., 2005).
Although some researchers have reported accumulation of carbohydrates and changes in the activities of related enzymes during fruit development and ripening, the role of sugars as signaling molecules regulating fruit maturity and quality in Asian pear has not been fully understood yet. Therefore, our goal was to investigate the regulatory mechanism controlling fruit maturity focused on sugar metabolism during fruit development and ripening in three Asian pear cultivars (‘Niitaka’, ‘Wonhwang’, and ‘Whangkeumbae’) previously identified for their contrasting harvest maturity periods.
Materials and Methods
Plant Materials
We used differently maturing Asian pear (Pyrus pyrifolia Nakai) cultivars grown in orchards in South Korea: ‘Whangkeumbae’ (early maturity) and ‘Wonhwang’ (early maturity) in Naju province (34°58'19.5"N, 126°46'01.9"E) and ‘Niitaka’ (late maturity) also in Naju province (34°58'34.1"N, 126°42'12.7"E). We used Information and Communication Technology (ICT)-based equipment, including a sensor to monitor soil or air temperature and moisture. The cultivars studied differ in skin color at harvest maturity: yellowish-green fruit skin color (‘Whangkeumbae’) and yellowish-brown skin color (‘Wonhwang’ and ‘Niitaka’). The full bloom date was April 8th for ‘Niitaka’, April 10th for ‘Whangkeumbae’, and April 11th for ‘Wonhwang’ in 2018. Pear fruit were harvested at 15-day intervals during the period from 57 to 177 days after full bloom (DAFB), based on ‘Niitaka’. On each sampling date, four replicates of fruit samples, with at least five fruit in each replicate from three trees, were harvested. The weights of the harvested fruit were immediately measured. The fruit were then cut into small pieces (after the core and peel were removed) and frozen in liquid nitrogen and stored in a deep freezer (–80°C) until use. The fruit fresh weight was previously published by Lee et al. (2021).
Measurement of Absolute Fruit Growth
Absolute fruit growth rate (AGR) was estimated according to the following equation (Zibordi et al., 2009): AGR = (Wt1 – Wt0)/(t1 – t0), where Wt1 and Wt0 are the estimated fruit weight (g) at a given time (t1) and at the previous sampling (t0).
Soluble Sugars and Starch Analysis
Soluble sugars such as glucose, fructose, and sucrose were determined according to the method of Wilson et al. (1981). Starch content was measured using the method of Baxter et al. (2003) with some modifications. After soluble sugar extraction with distilled water, the pellet was dried, suspended with 1 mL distilled water, and heated at 100°C for 10 min. The pH of the solution was adjusted to 5.1 by adding 400 µL 200 mM acetate buffer. To digest starch, 100 µL of reaction mixture containing 0.2 U amyloglucosidase and 40 U α-amylase was added to the solution and then incubated under gentle agitation at 50°C for 24 h. After centrifugation at 12,000 × g for 10 min, glucose content in the supernatant was determined as described above. Total soluble sugar content was calculated as the sum of glucose, sucrose, and fructose content.
Determination of Activities of Sucrose Metabolism-Related Enzymes
SPS activity was assayed according to the procedure described by Baxter et al. (2003). SPS activity was expressed as µg sucrose min-1·mg-1·protein (U·mg-1·protein). After SPS enzyme extraction, the supernatant was used for analysis of NINV and VINV activity. The pellet was re-extracted with acetate buffer (pH 4.8) containing 1 M NaCl and then the supernatant was used for analysis of CWINV activity. The activity of NINV, VINV, and CWINV was assessed according to Qin et al. (2016) and French et al. (2014), with some modification. For VINV and CWINV activity assays, 150 µL of the supernatant was mixed with 50 µL 500 mM sodium acetate buffer (pH 4.8) and 50 µL 20 mM sucrose, followed by incubation at 37°C for 0 or 30 min. After incubation, 250 µL DNS solution (composed of 0.2 M NaOH, 23 mM 3,5-dinitrosalicylate, and 0.53 M sodium potassium tartrate) was added to reaction mixture and boiled for 10 min. Reducing sugars released from sucrose were quantified at 540 nm. NINV activity was determined in 50 mM HEPES buffer (pH 7.5) instead of sodium acetate buffer (pH 4.8). Enzyme activities were expressed as µg reducing sugar min-1· mg-1·protein (U·mg-1·protein).
Statistical Analysis
The experiment was performed in a completely randomized design with four replicates for three cultivars at different fruit development stages. Duncan’s multiple range test was used to compare the means of separate replicates in each cultivar. All statistical analyses were performed using SAS 9.4 (SAS Institute Inc., Cary, NC, USA). Differences at p < 0.05 were considered significant. Heatmap, principal component analysis (PCA), and Pearson correlation analyses were conducted using MetaboAnalyst 3.0 (https://www.metaboanalyst.ca).
Results and Discussion
Morphological Changes in Three Asian Pear Cultivars During Fruit Development and Ripening
To characterize the developmental stages of fruit from three cultivars of Asian pear, ‘Niitaka’, ‘Wonhwang’, and ‘Whangkeumbae’, growth data were collected from 57 to 147 DAFB in ‘Wonhwang’ and ‘Whangkeumbae’ or 177 DAFB in ‘Niitaka’ by measuring fruit fresh weight at 15-day intervals (Fig. 1). Representative fruit from each developmental stage are shown in Fig. 1A. All the cultivars exhibited a single sigmoid growth pattern for fruit development (Fig. 1B), as shown by ‘Hwasan’ Asian pear (Seo et al., 2020). The early-maturing cultivars, ‘Wonhwang’ and ‘Whangkeumbae’, showed a period of rapid fruit growth from 72 to 117 DAFB, consistent with an increase in AGR. In the late-maturing cultivar, ‘Niitaka’, fresh weight and absolute fruit growth rate (AGR) gradually increased up to 147 DAFB (Fig. 1C). The fruit fresh weight was the highest in ‘Niitaka’, followed by ‘Wonhwang’, and the lowest was in ‘Whangkeumbae’ on the last harvest day (Fig. 1B and 1C).
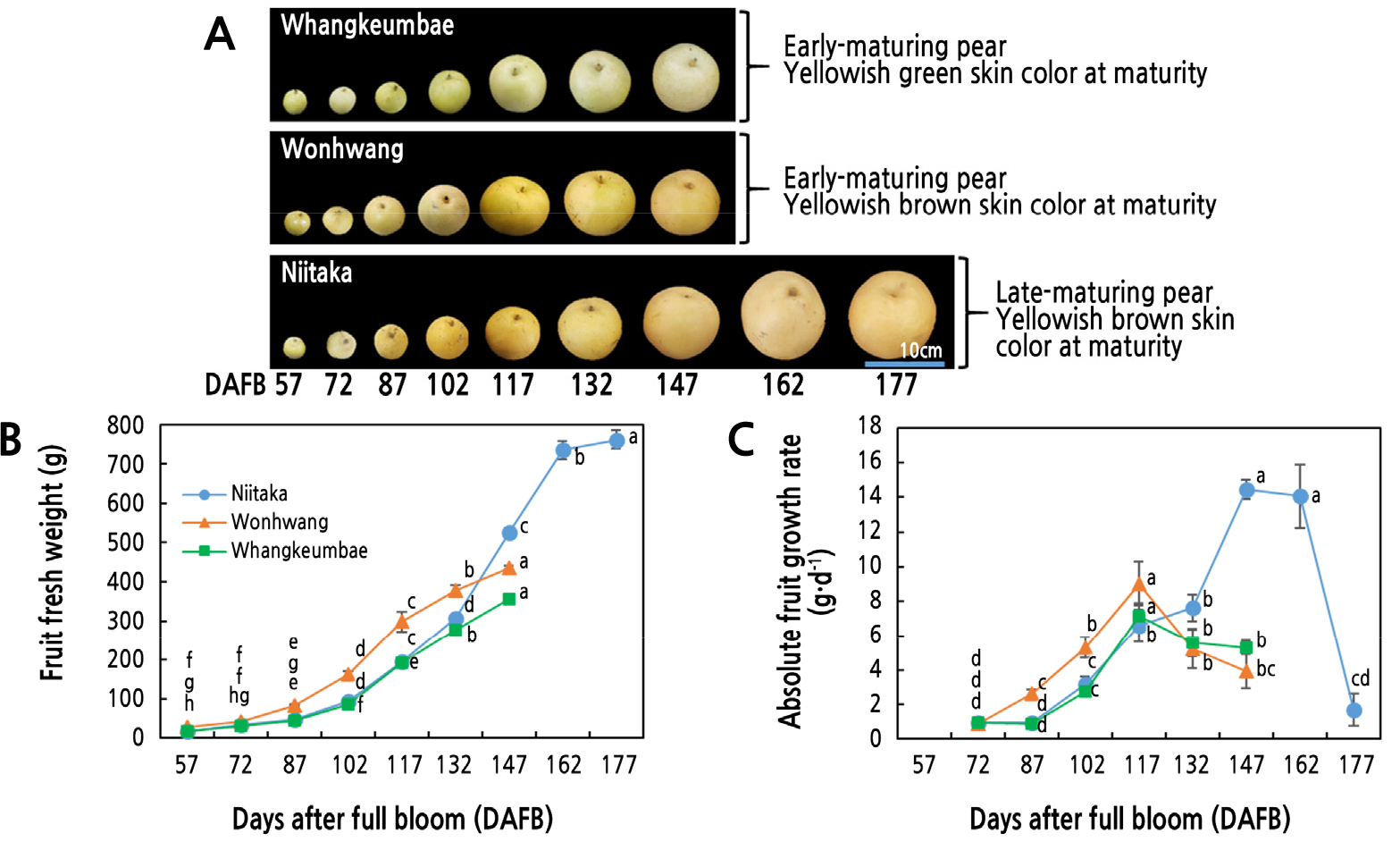
Fig. 1.
Seasonal changes in pear fruit development (A), fruit fresh weight (B), and absolute fruit growth rate (C) in three Asian pear cultivars having different maturity periods during fruit development and ripening. Values are presented as means ± SD of 20 fruits. Values marked with different letters are significantly different between stages of fruit development in each cultivar (p < 0.05).
Concentrations of Soluble Sugars and Starch in Three Asian Pear Cultivars During Fruit Development and Ripening
The content of the soluble sugars, such as glucose, fructose, and sucrose and starch were measured during fruit development and ripening. Total soluble sugars content in all three cultivars gradually increased with fruit development, showing higher levels in ‘Wonhwang’ and ‘Whangkeumbae’ than in ‘Niitaka’ (Fig. 2D). Glucose content gradually increased by 102 DAFB in ‘Wonhwang’, 117 DAFB in ‘Whangkeumbae’, and 147 DAFB in ‘Niitaka’ and then slightly decreased or remained steady until the end of harvest. Glucose content was the highest in ‘Whangkeumbae’, followed by ‘Wonhwang’, and the lowest in ‘Niitaka’ during the early stage of fruit development (Fig. 2A). Fructose content was similar in ‘Wonhwang’ and ‘Whangkeumbae’ but higher than in ‘Niitaka’ (Fig. 2B). Hexose (glucose + fructose) is used as a carbon source for fruit growth and development, while excess carbon is converted to starch for storage in plastids or transported into the vacuole by sugar transporters for accumulation. In this study, starch accumulation was observed only during the early stage of fruit development to store excess carbon (Fig. 2F). Accumulation of hexose in the vacuole increases osmotic potential, driving the influx of water, which results in an increase in turgor pressure and, thus, irreversibly extended cell walls (Wang and Ruan, 2013). Moreover, glucose also acts as a signaling molecule, regulating the cell cycle and cell wall expansion (Ruan et al., 2010). Indeed, endogenous hexose levels are positively associated with cell proliferating activity. For instance, exogenous glucose up-regulated expression of CycB and CycD,key cell cycle genes, results in fruit enlargement (Perrot-Rechenmann, 2010). In addition, high fructose levels may stimulate expression of genes responsible for cell division such as Cyclin delta2 and 3 (Soni et al., 1995). Therefore, the content of hexose for cell expansion is crucial for determining fruit size.
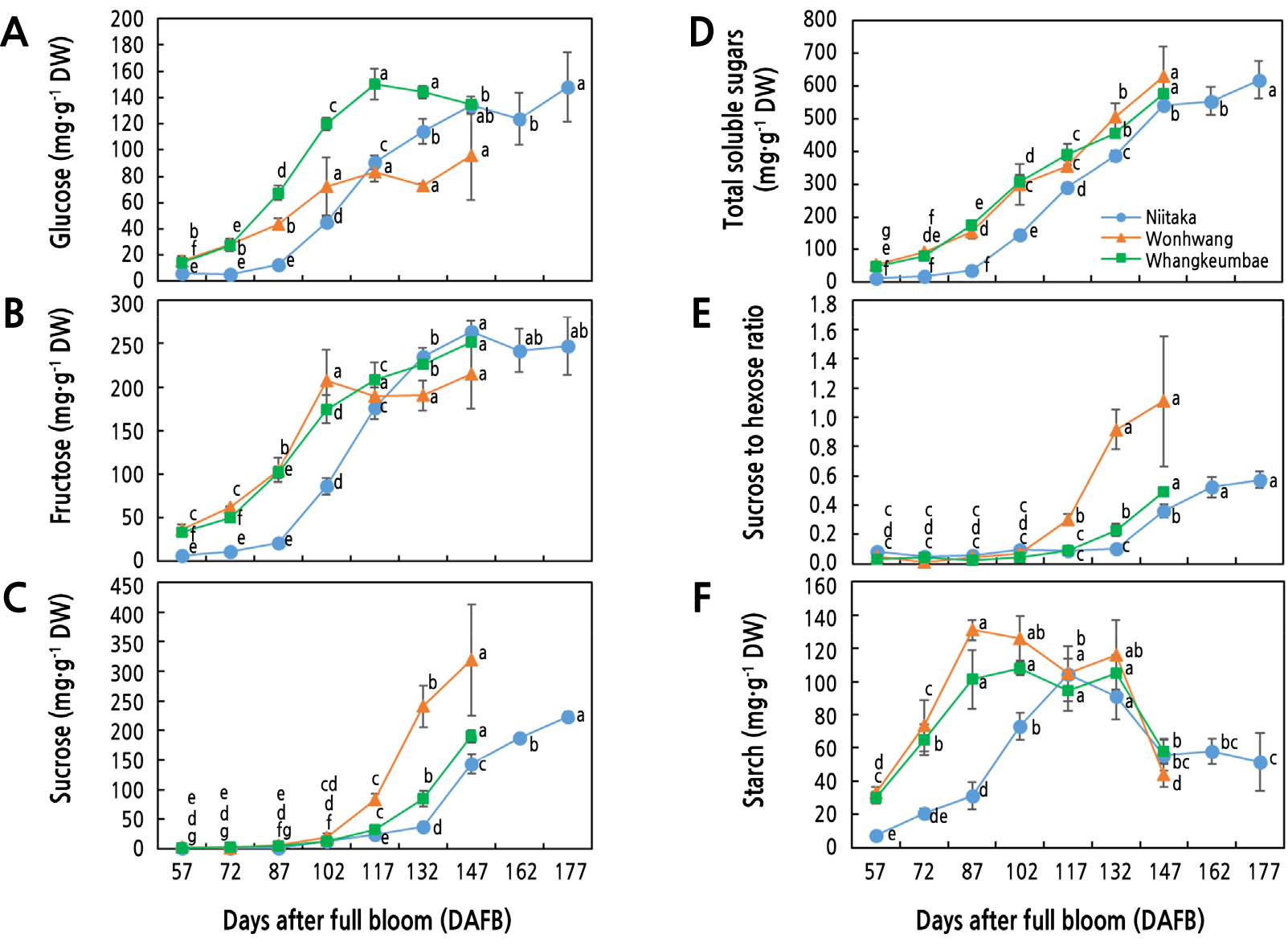
Fig. 2.
Seasonal changes of carbohydrate compounds in three Asian pear cultivars having different maturity periods during fruit development and ripening. A: Glucose, B: fructose, C: sucrose, D: total soluble sugars, E: sucrose-to-hexose ratio, and F: starch. Values are presented as means ± SD of four biological replicates. Values marked with different letters are significantly different between stages of fruit development in each cultivar (p < 0.05).
In contrast to hexose, an increase in sucrose content was detected during late stage of development and ripening. Indeed, sucrose content rapidly increased from 102 DAFB to the end of harvest, and it was the highest in ‘Wonhwang’, the cultivar with the earliest maturity, followed by ‘Whangkeumbae’ and ‘Niitaka’ (Fig. 2C). It has been reported that sucrose accumulation is closely associated with fruit ripening. For example, overexpression of sucrose transporter in apple promoted fruit ripening due to accumulation of endogenous sucrose (Peng et al., 2020). Moreover, sucrose content remained low and relatively stable in the early stages of the green phase and increased sharply when fruit began to enter the yellow-green or yellow-brown color phases (Figs. 1A and 2C), as suggested by Jia et al. (2021). This was consistent with the increase in sucrose-to-hexose ratio (Fig. 2E). The sucrose-to-hexose ratio began to significantly increase from 102 DAFB in ‘Wonhwang’ and Whangkembae and 132 DAFB in ‘Niitaka’, concomitant with the highest AGR (Fig. 1C), indicating a shift in the fruit development process from cell expansion to maturity. Therefore, ‘Wonhwang’ and ‘Whangkeumbae’, having an earlier increase in the sucrose-to-hexose ratio, may have faster fruit maturity than ‘Niitaka’.
In all the three pear cultivars, fructose was the most abundant soluble sugar during the early stage of fruit development, and the proportion of sucrose rapidly increased as fruit matured and ripened (Fig. 2B and 2C). In ‘Niitaka’, fructose accounted for 60% of the total soluble sugars during the early stage of fruit development, but it decreased to 40% during the late stage of fruit development. Sucrose rapidly increased and accounted for 36% of the soluble sugars during the late stage of fruit development. Similar results were observed in ‘Whangkeumbae’. Fructose and sucrose in ‘Wonhwang’ were the most abundant soluble sugars at 70% and 51% during the early and late stages of fruit development, respectively.
Activities of Key Enzymes Related to Sugar Metabolism in Three Asian Pear Cultivars During Fruit Development and Ripening
During fruit growth and development, sucrose produced from leaves is loaded into phloem, and unloaded into fruit cells, and then hydrolyzed into glucose and fructose by CWINV, NINV, and VINV. CWINV activity gradually increased from 87 DAFB to the end of harvest day in all three pear cultivars (Fig. 4A). Similar findings were reported in other fruit, including apple (Zhang et al., 2004) and grape (Zhang et al., 2006), suggesting that sucrose unloading through the apoplastic pathway may be related to maintenance of the sucrose gradient between phloem and apoplast. It has been reported that CWINV is closely related to sink strength. For example, elevation of the endogenous CWINV activity by silencing its inhibitor promoted fruit yield in tomato (Jin et al., 2009), whereas inhibition of CWINV resulted in small size seed phenotype due to reduced mitotic activity, leading to low cell number (Vilhar et al., 2002).
On the contrary, the activity of both NINV and VINV, which hydrolyze sucrose to glucose and fructose in cytosol and vacuolar, respectively, was found to be significantly increased at the early stage but decreased or maintained at the late stage (Fig. 3B and 3C). The activity of NINV and VINV increased until 102 DAFB in ‘Wonhwang’ (13.3-fold) and ‘Whangkeumbae’ (4.2-fold) and 162 DAFB in ‘Niitaka’ (14.2-fold); the activities were much higher in ‘Wonhwang’ and ‘Whangkeumbae’ than that in ‘Niitaka’ at the early stage of fruit development (Fig. 3B and 3C). The higher VINV activity in the early stage is accompanied by greater hexose accumulation, which results in osmotic effects that promote the uptake of nutrients and water in the vacuole, consequently enhancing cell expansion (Ruan et al., 2010). Indeed, AGR increased along with an increase in glucose and fructose content, indicating that the increase in hexose content might be related to cell expansion (Figs. 1C, 2A, and 2B). Kohorn et al. (2006) suggested that VINV promotes cell expansion through an effect on cell wall extensibility in mutants of a cell wall-associated receptor-like kinase. Similarly, NINV was recently reported to be responsible for cellulose biosynthesis by providing uridine 5'-diphosphoglucose (UDPG) (Rende et al., 2017). Therefore, this study suggests that an increase in VINV and NINV in the early stage of fruit development may have more of an effect on cell structure through glucose signaling.
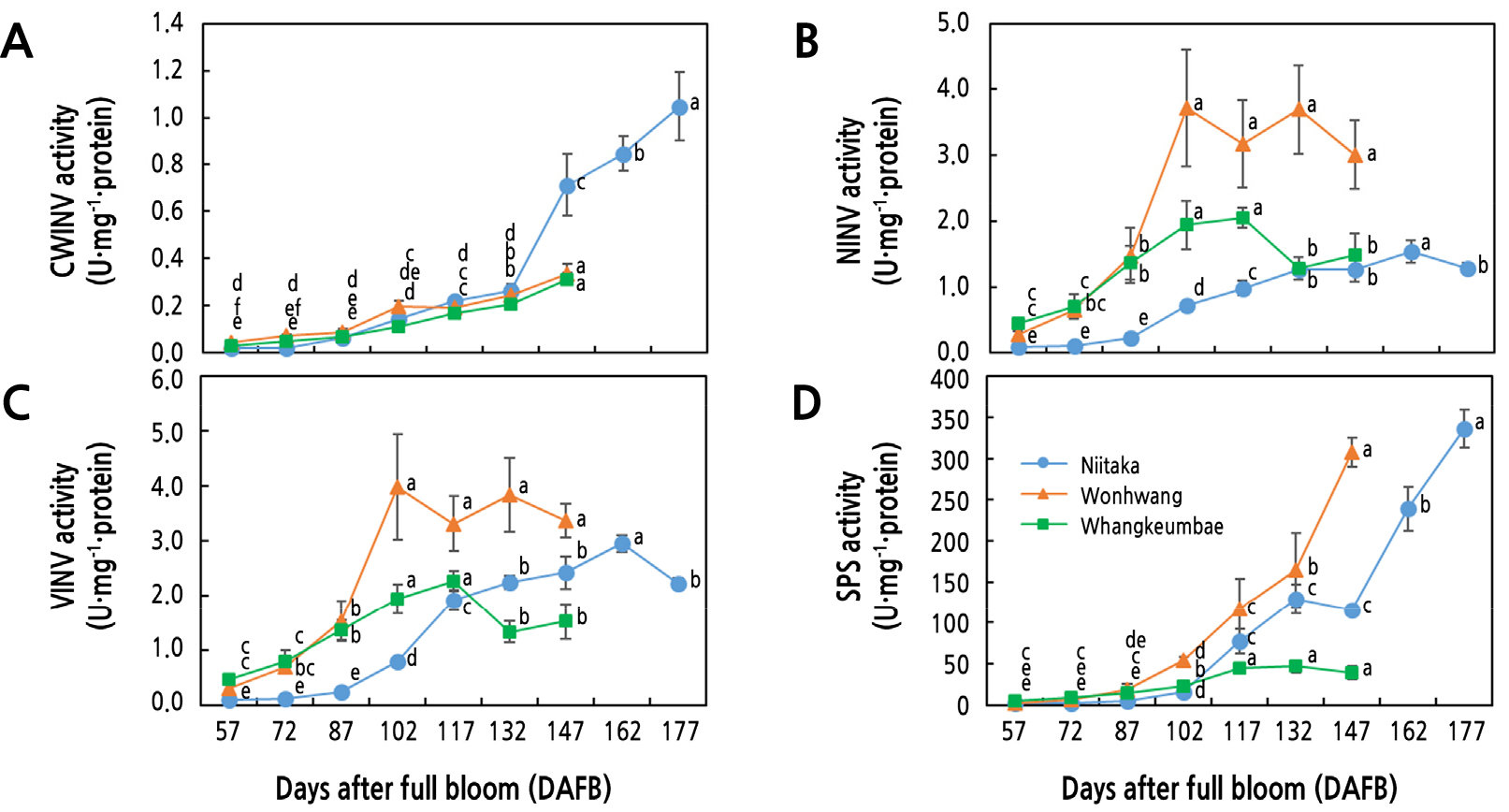
Fig. 3.
Activities related to sugar metabolism in three Asian pear cultivars having different maturity periods during late stage of fruit development and ripening. A: CWINV; cell wall invertase, B: NINV; neutral invertase, C: VINV; vacuolar invertase, and D: SPS; sucrose phosphate synthase. Values are presented as means ± SD of four biological replicates. Values marked with different letters are significantly different between stages of fruit development in each cultivar (p < 0.05).
During the late stage of fruit development, the decreases in VINV and NINV were consistent with sucrose accumulation in ‘Wonhwang’ and ‘Whangkeumbae’ but not in ‘Niitaka’ (Figs. 2 and 3). Sucrose accumulation in ‘Niitaka’ (from 102 DAFB) occurred before VINV activity decreased (from 162 DAFB), which may be due to an increase in SPS activity (Figs. 2 and 3). The SPS enzyme is related to re-synthesis of sucrose from fructose-6-phosphate and UDPG or glucose released from starch breakdown, which is closely associated with sucrose accumulation. In addition, Lombardo et al. (201l) observed a dramatic increase in SPS in maturing peach. SPS activity in ‘Niitaka’ was significantly increased from 87 DAFB to the end of harvest (Fig. 3D). Therefore, these results indicate that sucrose accumulation is differently regulated by SPS or invertase according to fruit maturity.
Heatmap Analysis of Relative Abundance of Sugar Metabolites and Related Enzyme Activities According to Fruit Maturity in Three Asian Pear Cultivars
To demonstrate the effect of maturity on fruit growth, sugar metabolites, and related enzyme activities, we created a heatmap visualization by comparing early-maturing cultivars and the late-maturing cultivar during fruit development and ripening (Fig. 4). Fruit maturity altered the relative abundance of a broad range of sugar metabolites and related enzyme activities. During the early stage of fruit development, the early-maturing cultivars, ‘Wonhwang’ and ‘Whangkeumbae’, showed relatively higher levels of fruit growth, sugar metabolites, and its related enzymes than did the late-season maturing cultivar (‘Niitaka’), with the exception of sucrose. Compared to ‘Niitaka’, the higher hexose content and invertase activities in ‘Wonhwang’ and ‘Whangkeumbae’ during the early stage of fruit development may be involved in promoting cell expansion, as shown by the higher fresh weight and AGR, as suggested by Ruan et al. (2010). In contrast, during the late stage of fruit development, sucrose and the sucrose-to-hexose ratio were relatively higher in ‘Wonhwang’ and ‘Whangkeumbae’ than in ‘Niitaka’, but the other parameters were relatively lower. Recently, enhancement of sucrose accumulation has been shown to promote fruit ripening in grape (Zhang et al., 2006) and peach (Lombardo et al., 2011), accompanied by an increase in the sucrose-to-hexose ratio. These results indicate that the rapid transition of the increase in sucrose from the increase in hexose results in the faster fruit maturity.
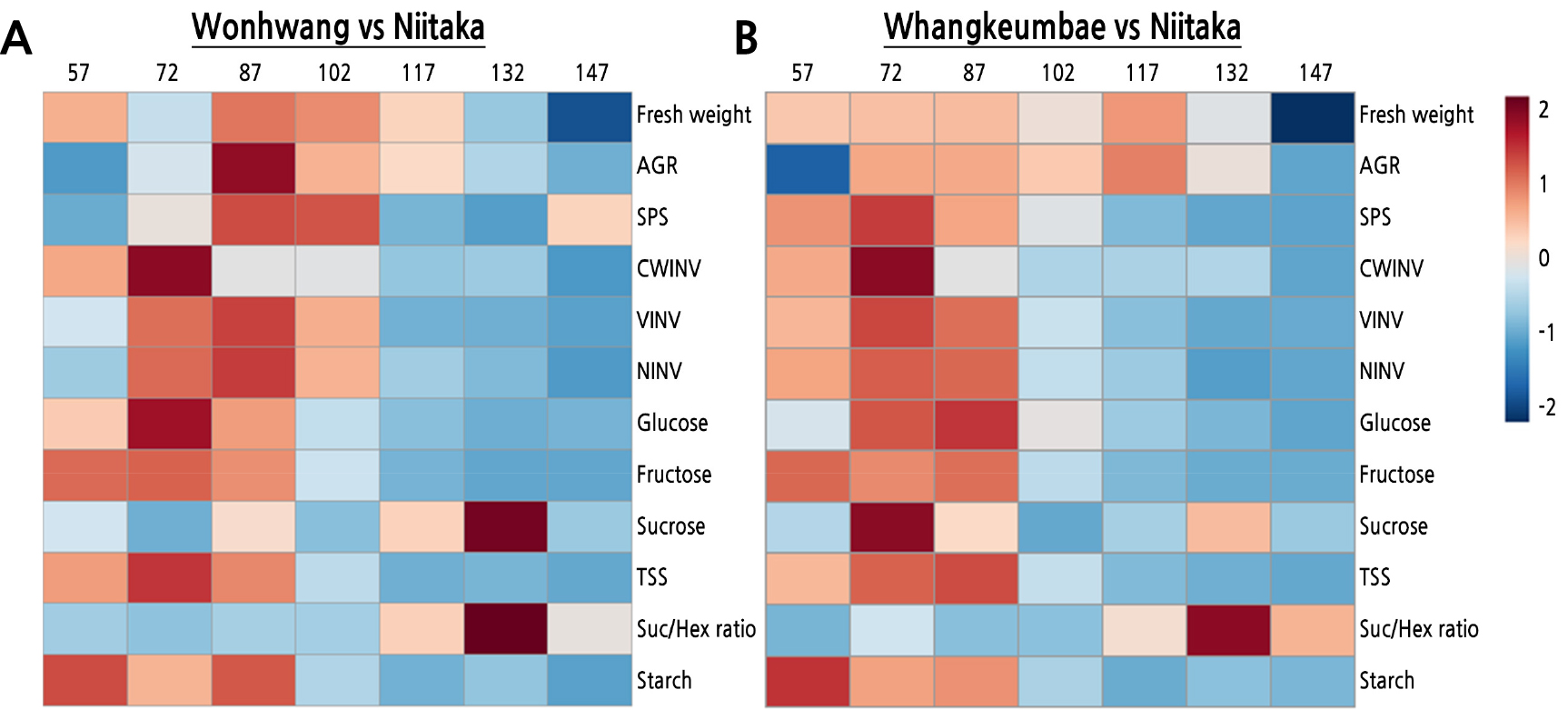
Fig. 4.
Heatmap displaying changes of relative abundances in identified morphology, metabolites, or enzyme activities in three Asian pear cultivars during fruit development and ripening. A: Wonhwang vs Niitaka, B: Whangkeumbae vs Niitaka. The normalization procedure consisted of mean row centering with color scales. 57, 72, 87, 102, 117, 132, and 147 indicate days after full bloom (DAFB). AGR, absolute fruit growth rate; SPS, sucrose phosphate synthase; CWINV, cell wall invertase; NINV, neutral invertase; VINV, vacuolar invertase; TSS, total soluble sugars; Suc/Hex ratio, sucrose to hexose ratio.
PCA and Pearson Correlation Analysis of Parameters of Fruit Growth, Metabolites, and Enzyme Activity
PCA was conducted to compare impacts of the physiological and morphological parameters on fruit development and ripening in three pear cultivars (Fig. 5). The cumulative contribution of the first and the second principal components attained 98.4%, 96.3%, and 98.9% in ‘Niitaka’, ‘Wonhwang’, and ‘Whangkeumbae’, respectively. In ‘Niitaka’, PCA distinctly separated the fruit development into two groups, from 52 to 117 DAFB in the negative region of PC1 and from 132 to 177 DAFB in the positive region of PC1 (Fig. 5A). In both ‘Wonhwang’ and ‘Whangkeumbae’, PCA also indicated a fruit development-specific response from 52 to 102 DAFB and from 102 to 147 DAFB, clearly separated into negative and positive regions of PC1, respectively (Fig. 5B and 5C). This analysis highlights a clear metabolic and morphologic shift from the early to late stage of development during fruit development and ripening, as reported by Lombardo et al. (2011). Similarly, Jing and Malladi (2020) suggested that the early stage of development is metabolically separated from the late stage of development due to changes in sucrose metabolism. Sucrose catabolism provided carbon backbones and energy for cell production during the early stage of development, but starch breakdown and resumption of the increase of sucrose occurred for cell expansion and ripening during late stage of development. In this study, the late stage of fruit development according to PC1 is significantly consistent with an increase of the sucrose-to-hexose ratio (Fig. 2E).
To further investigate the physiological relationship between measured variables in the three pear cultivars based on the period of increase of the sucrose-to-hexose ratio on each cultivar (a period corresponding to the positive region of PC1), we used Pearson's correlation coefficient among the descriptive parameters of fruit growth, sugar metabolites, and related enzyme activity (Fig. 6). In all three pear cultivars, the sucrose-to-hexose ratio, which was positively correlated with fresh weight, sucrose, CWINV, and total soluble sugars was negatively correlated with starch. The correlations between the sucrose-to-hexose ratio and NINV or VINV were positive in ‘Niitaka’ (late-maturing cultivar) but negative in ‘Wonhwang’ and ‘Whangkeumbae’ (early-maturing cultivar). The correlations with SPS were positive in ‘Niitaka’ and ‘Wonhwang’ but negative in ‘Whangkeumbae’.
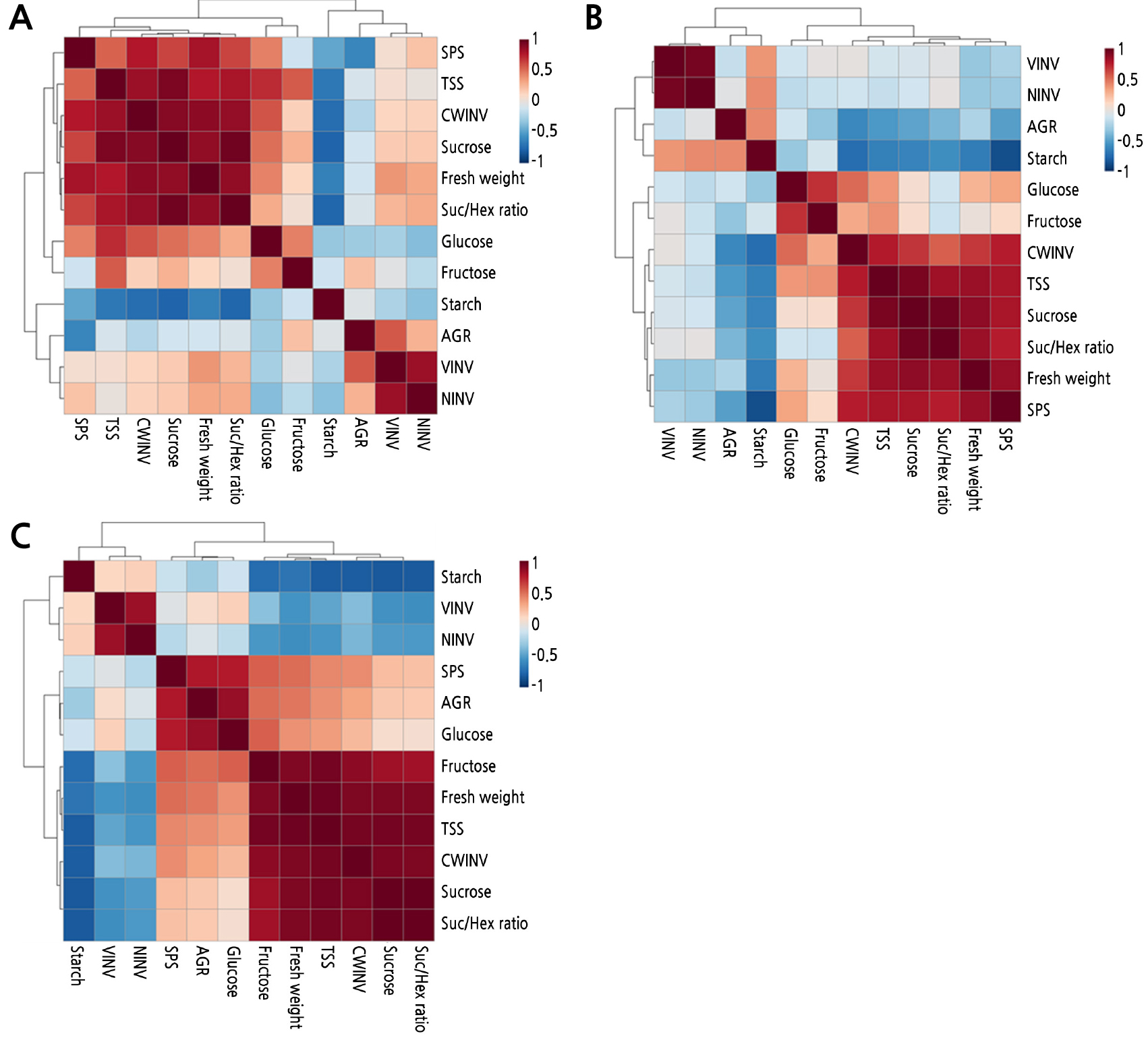
Fig. 6.
Heatmap showing the correlations among the identified morphology, metabolites, or enzyme activities in three Asian pear cultivars, based on period for increase of sucrose-to-hexose ratio in each cultivar. A: Niitaka, B: Wonhwang, and C: Whangkeumbae. Correlation coefficients were calculated based on Pearson’s correlation. Red indicates a positive effect, whereas blue indicates a negative effect. Color intensity is proportional to the correlation coefficients. AGR, absolute fruit growth rate; SPS, sucrose phosphate synthase; CWINV, cell wall invertase; NINV, neutral invertase; VINV, vacuolar invertase; TSS, total soluble sugars; Suc/Hex ratio, sucrose to hexose ratio.
Conclusions
The early-maturing cultivars, ‘Wonhwang’ and ‘Whangkeumbae’, showed smaller fruit size and reached the highest AGR for a short period, compared to the late-maturity cultivar, ‘Niitaka’. A dramatic increase in the activity of CWINV, NINV, and VINV was observed during the early stage of fruit development, concomitant with an increase in hexose content (glucose and fructose); the values were relatively higher in ‘Wonhwang’ and ‘Whangkeumbae’ than in ‘Niitaka’. On the contrary, when the activity of NINV and VINV was the highest, sucrose content started to increase until the end of harvest. Sucrose content and the sucrose-to-hexose ratio were relatively higher in Wohnwhang and ‘Whangkeumbae’ than in ‘Niitaka’ during late stage of fruit development and ripening. In this stage, the sucrose-to-hexose ratio is positively correlated with fresh weight, CWINV, sucrose, and SPS (especially in ‘Wonhwang’ and ‘Niitaka’) and is negatively correlated with the activity of NINV and VINV (especially in ‘Whangkeumbae’) (Fig. 6). Based on these data, we found that sucrose is differently accumulated by an increase in sucrose re-synthesis by SPS using glucose hydrolyzed by CWINV in ‘Wonhwang’ and ‘Niitaka’ or by a decrease in NINV and VINV activities in ‘Whangkeumbae’. These results indicate that maturity of Asian pear fruit during fruit development and ripening may be closely related to an increase in the sucrose-to-hexose ratio regulated by invertase and sucrose re-synthesis. The integration of this information with knowledge of carbohydrate metabolites and enzyme activities will provide a better understanding about the relationship between carbohydrate metabolism and fruit development and ripening.