Introduction
Materials and Methods
Reagents
Fungal Inoculum
DNA Extraction and PCR Amplification
Fruit Sampling
In Vitro Antifungal Assay
Effect of PAA on Growth of B. cinerea in vitro
Preharvest Treatment and Storage Conditions
Effect of PAA Treatment on Lesion Development in Strawberries
Microbiological Assay
Statistical Analysis
Results and Discussion
Introduction
Strawberries (Fragaria × ananassa Duch.) are perishable fruits that are susceptible to moisture loss, physical damage, and pathogen infections due to the lack of a protective rind and a high respiration rate (Lan et al., 2019). Gray mold, caused by Botrytis cinerea, is one of the most destructive diseases of strawberries, resulting in postharvest reductions in fruit quality (Romanazzi et al., 2016).
The fungus B. cinerea is an opportunistic pathogen that invades weak or senescent floral tissues early in the growing season, remains latent until fruit maturity, and later causes fruit decay through conidia that form on adjacent necrotic tissues, particularly infected stamens and calyces (McClellan and Hewitt, 1973; Mertely et al., 2000; Romanazzi et al., 2016). This pathogen can also infect plant tissues through physical injuries that occur during harvesting and subsequent processing. Damage to fruits that may occur during harvesting, packaging, transportation, and handling can significantly reduce fruit quality (Van Zeebroeck et al., 2007; Aliasgarian et al., 2015; Al-Dairi et al., 2022) and facilitate the entry of wound-invading necrotrophic fungi, such as Penicillium expansum and B. cinerea (Janisiewicz and Korsten, 2002). Although significant differences have been reported in the susceptibility of commercial strawberry cultivars to Botrytis fruit rot, none of the current cultivars have been found to be completely resistant (Mertely et al., 2000; Xiao et al., 2001; Legard et al., 2005). Therefore, the use of chemical fungicides is standard to ensure productivity and to protect susceptible crops from gray mold disease.
To minimize postharvest decay, the field application of chemical fungicides during the growing season has been a major disease management strategy over the past half century (Tripathi and Dubey, 2004; Mari et al., 2014). Although chemical control offsets yield losses caused by Botrytis rot, the deleterious effects of fungicides on pollination, seed set, and fruit formation (Kovach et al., 2000; Yi et al., 2003) cannot be overlooked. The emergence of B. cinerea mutants resistant to various active ingredients (well summarized in the literature by Romanazzi and Feliziani, 2014) that are used for gray mold control has been frequently reported worldwide (Leroch et al., 2013; Mari et al., 2014; Romanazzi and Feliziani, 2014). This has made it challenging to control diseases caused by B. cinerea. Consumer concerns regarding fungicide residue on fruits are even more pronounced in the case of strawberries. Repeated fungicide spraying is a common practice in strawberry cultivation, and fruits harvested every few days are susceptible to contamination by accumulated fungicides (Stensvand and Christiansen, 2000; Wang et al., 2021). Moreover, the potential adverse effects of chemical fungicides on humans, such as carcinogenicity, teratogenicity, and acute and chronic toxicity (Komárek et al., 2010; Oruc, 2010; Rouabhi, 2010), have prompted the search for alternative, safer, and sustainable methods of controlling postharvest diseases.
Peracetic acid (PAA) is a strong oxidant with a higher oxidation potential (E0 = 1.96 V) than other known sanitizing agents (Kim and Huang, 2021). Given its broad spectrum of antimicrobial activities, PAA has been widely used as an effective disinfectant in various industries, including food and beverage processing, medical device applications, pharmaceuticals, cooling systems, and sewage treatment (McDonnell and Russell, 1999; Kitis, 2004). PAA is a nonspecific oxidizing agent that reacts with reduced sulfur and C-C double bonds (Kitis, 2004; Finnegan et al., 2010; Wessels and Ingmer, 2013). Given the nonspecific modes of action of PAA, microorganisms are unlikely to be less susceptible or acquire resistance to it. Moreover, no reports of resistance have been found in the literature (Wessels and Ingmer, 2013). Microorganisms possess several potential targets sensitive to oxidation by PAA, e.g., sulfhydryl (-SH) groups present in the active domain of essential metabolic enzymes, in addition to unsaturated fatty acids (C-C double bonds) present in the cell membrane. In contrast, the active ingredients in chemical fungicides used for controlling gray mold are site-specific inhibitors (Romanazzi and Feliziani, 2014). The potential harmful impacts of PAA on the environment and human health are negligible. PAA is decomposed into water and acetic acid, which are not harmful products. Moreover, disinfection by-products (DBPs) formed by the reaction of PAA with organic matter mainly include carboxylic acids and aldehydes, which are less mutagenic and carcinogenic than halogenated DBPs generated from chlorine-based disinfectants (Monarca et al., 2002; Crebelli et al., 2005) and are readily biodegradable (Kitis, 2004). In a study by Lee and Huang (2019), who analyzed the formation of DBPs during washing processes, a PAA treatment led to the formation of negligible levels of haloacetic acids, nitrogenous DBPs, and carbonaceous DBPs and fewer aldehyde DBPs than a sodium hypochlorite (NaOCl) treatment in both lettuce wash water and fresh-cut lettuce. As mentioned above, compared with conventional fungicides, PAA is associated with a broader antimicrobial spectrum, a lower risk of resistance, and fewer toxic DBPs and may therefore be a promising alternative for controlling postharvest decay and thereby prolonging the shelf life of fruits.
Several studies have introduced effective and applicable methods to extend the shelf life of strawberries (Gol et al., 2013; Velickova et al., 2013; Khan et al., 2019; Chu et al., 2020; Kalantari et al., 2020; Shehata et al., 2020; Kim et al., 2021). However, to the best of our knowledge, no studies have examined the effects of a preharvest PAA treatment on the natural decay of strawberries during postharvest storage at ambient temperatures. Therefore, this study aimed to assess the effect of a preharvest PAA treatment on the natural decay of strawberries during postharvest storage. In particular, the delay in fruit spoilage under room-temperature conditions equivalent to those during commercial operations in a retail market was assessed. Moreover, the susceptibility of B. cinerea to PAA was assessed in vitro using various assays, and the concentration of PAA required to inhibit mycelial growth completely was determined. To confirm the effect of the PAA treatment on the natural decay of strawberries, the decay incidence and density of microbial populations in fruits treated with various concentrations of PAA 1 h before harvest and packaged in expanded polystyrene (EPS) boxes were investigated during storage at room temperature for seven days. The efficacy of PAA was also confirmed by examining whether lesion development in strawberries artificially inoculated with B. cinerea was inhibited by PAA in a dose-dependent manner. The obtained results may provide an alternative method to ensure the postharvest quality of strawberries by slowing down their natural decay and thereby extending their shelf life.
Materials and Methods
Reagents
Potato dextrose agar (PDA) and dichloran rose bengal chloramphenicol (DRBC) agar were purchased from BD Difco (Lawrence, KS, USA). Commercial PAA (5% PAA, 18.78% H2O2, and 15.53% acetic acid by weight) was obtained from Kumsung E&C Co., Ltd. (Ansan, Korea). All other chemicals were purchased from Sigma-Aldrich (St. Louis, MO, USA), unless stated otherwise.
Fungal Inoculum
Isolates of B. cinerea were obtained from rotten strawberries collected from a local farm (Naju, Korea) and maintained on PDA at 24°C. Conidia of B. cinerea were prepared by flooding 8–10-day-old PDA cultures of B. cinerea with 10 mL of PD broth containing 0.1% (v/v) Tween 20 and rubbing the plates with an L-shaped glass rod. The resulting conidial suspension was filtered using a 70-µm cell strainer (Corning, NY, USA) and adjusted to the desired concentration using a hemocytometer (Marienfeld, Lauda-Königshofen, Germany).
DNA Extraction and PCR Amplification
Isolation of genomic DNA was conducted as described previously (Yuan, 2019). The sequence-characterized amplified region (SCAR) marker sets used for the species identification of fungal isolates were C729+/C729‒, Bc108+/Bc563‒, and Bc-f/Bc-r; these were obtained from Rigotti et al. (2002), Rigotti et al. (2006), and Fan et al. (2015), respectively. PCR was performed with a total reaction volume of 20 µL consisting of 10 ng genomic DNA, 0.5 µM of each primer, 0.2 mM dNTPs, 1× PCR buffer, and 0.5 U Taq DNA polymerase (Takara, Tokyo, Japan) using a TProfessional Thermocycler (Biometra, Göttingen, Germany). Thermocycling was conducted under the following conditions: 98°C for 2 min; 30 cycles of 98°C for 10 s, 52°C for 30 s, and 72°C for 1 min; and a final step at 72°C for 5 min. PCR products were visualized by electrophoresis on 1% (w/v) agarose gel in 0.5× Tris acetate–EDTA buffer, followed by staining using ethidium bromide and UV exposure.
Fruit Sampling
Strawberries (Fragaria × ananassa Duch., cv. Seolhyang) were obtained from a local farm in Hwasun, Jeollanam-do, Korea. Strawberries that had a uniform size with three-fourths of the surface being red in color, were free of physical damage, and were free of fungal decay were used.
In Vitro Antifungal Assay
Disc Diffusion Assay
Antifungal susceptibility of B. cinerea was assessed using the disc diffusion assay method. In brief, a 100-µL aliquot of spore suspension (1 × 107 cells/mL) was uniformly spread over the surface of PDA. PAA and NaOCl dilutions were prepared at concentrations ranging from 62.5 to 2000 ppm in PBS. Sterile paper discs (8 mm diameter) were treated with 50 µL of the PAA or NaOCl dilutions and then placed on the surface of the PDA. PBS was used as a negative control. NT denotes the sample that was left untreated. The plates were incubated at 24°C for four days and the growth inhibition zone around the discs was measured.
Radial Mycelial Growth
The antifungal activity of PAA was also assessed using the radial mycelial growth assay method. The spore suspension (1 × 106 cells/mL) was mixed with an equal volume of PAA for dilution. Following this, 50 µL of the mixture was pipetted into the center of the PDA plates. The radial mycelial growth of fungal spores treated with various concentrations of PAA was compared with that of non-treated fungal colonies on day 1 and after days 3 and 5 of incubation.
Spray Treatment
The inhibitory effect of PAA on fungal growth was assessed by directly adding it to growing mycelium. An agar block (10 mm diameter) was transferred from a 6–7-day-old culture of B. cinerea to fresh PDA and incubated at 24°C for three days. PAA dilutions ranging from 250 to 8000 ppm were directly sprayed onto fungi growing on PDA. PBS was used as a negative control. The inhibition of fungal growth by PAA was assessed by incubating the plates at 24°C for two days.
WST-1-based Microdilution Assay
A colorimetric WST-1 assay (Roche Diagnostics GmbH, Mannheim, Germany) was utilized to assess the susceptibility of B. cinerea to PAA, in accordance with the manufacturer’s instructions. In brief, 100-µL aliquots of spore suspensions treated with various concentrations of PAA were transferred to the individual wells of a 96-well microplate, following which the plates were incubated at 24°C for 24 h. Subsequently, 10 µL of WST-1 was added to each well. The plates were then incubated for an additional 18 h at 24°C. To quantify the production of yellow-colored formazan, absorbance at 450 nm was measured using a Mobi microplate reader (MicroDigital Co., Ltd., Seongnam, Korea). The measured absorbance was adjusted by subtracting the background absorbance at 570 nm.
Effect of PAA on Growth of B. cinerea in vitro
The growth of B. cinerea was measured in a 1% malt extract broth containing different concentrations of PAA. The spore suspension (7.5 × 104 cells/mL) was dispensed as 200-µL aliquots into six replicate wells of a 96-well plate. The plate was incubated at 24°C, and the growth of B. cinerea was monitored over a 72-h period by measuring the optical density at 490 nm. In another set of experiments, PAA was treated at 24 or 48 h after incubation and fungal growth was monitored every 24 h up to 96 h.
Preharvest Treatment and Storage Conditions
The effect of the preharvest PAA treatment on the natural decay of strawberries during room-temperature storage was assessed. One hour before harvest, each experimental block was sprayed using a hand sprayer with various concentrations (200, 400, or 800 ppm) of PAA or with water to cover all surfaces of the plants completely. The control group was left untreated. After picking strawberries with gloved hands, they were placed in EPS foam boxes (245 × 190 × 110 mm; 65–70 fruits per box), similar to that done during commercial operations. The boxes were then wrapped with a polyvinyl chloride (PVC) stretch film and taken back to the laboratory. To simulate retail display conditions, the treated fruits were placed at room temperature at 20 ± 2°C and 45–50% relative humidity (RH) for up to seven days. Fruits from each treatment group were inspected daily during the storage period to detect visible fungal infection, brown spots, or softening of wounded areas. Decay incidence was recorded and expressed as the percentage of infected fruits.
Effect of PAA Treatment on Lesion Development in Strawberries
The conidial suspension, prepared as described above, was diluted with distilled water (DW) to yield a final concentration of 2 × 104 conidia per mL. A single 3-mm-deep wound was made on the equatorial zone of each fruit using a sterile yellow tip. Following this, 500 µL of 200, 400, or 800 ppm PAA or DW was sprayed to thoroughly cover the whole fruit surface. To ensure PAA absorption into the wounded area of the fruit, the pathogen was inoculated 2 h after the PAA treatment. Pathogen inoculation was performed by pipetting 10 µL of conidial suspension. All inoculated fruits were maintained in closed plastic containers (five fruits per container) at 20 ± 2°C and 45–50% RH for up to five days and their lesion diameters were then recorded.
Microbiological Assay
Four strawberries (60 ± 4 g) from each treatment group were randomly taken from EPS boxes zero, five, and seven days after storage and placed in a sterile zipper bag. Following this, 240 mL of 0.1% peptone water was added to the bag. The bag was thoroughly massaged by hand for 5 min to dislodge any microorganisms present on the surface of the fruits. The resulting suspension was then serially diluted with 0.1% peptone water, and 0.1-mL aliquots were plated in triplicate on DRBC agar plates. The plates were incubated in the dark at 24°C for three days. The yeast and mold populations from each treatment group were counted. The results are expressed in log CFU/g.
Statistical Analysis
The results were analyzed using SAS 9.4 (SAS Institute Inc., Cary, NC, USA). The average logarithms of the number of colony-forming units per gram (log CFU/g), the decay incidence, and the lesion diameter for each treatment group were compared using Duncan’s multiple range test at 5% probability.
Results and Discussion
For species identification, PCR analyses of eight fungal isolates was conducted using SCAR markers derived from random amplified polymorphic DNA (RAPD) fragments of B. cinerea. Species-specific fragments (729, 475, and 327 bp) of B. cinerea were successfully amplified in all fungal isolates collected from rotten strawberries (Fig. 1). All fungal isolates were identified as B. cinerea by a PCR analysis using species-specific SCAR markers. SCAR markers are being developed to detect and identify various plant pathogens on their host plants. Two SCAR markers, C729+/C729‒ and Bc108+/Bc563‒, have been designed to specifically detect the sequences derived from RAPD fragments of B. cinerea (Rigotti et al., 2002, 2006). Moreover, the primer set C729+/C729‒ has successfully been used to detect B. cinerea in strawberries (Rigotti et al., 2002), grapes (Gindro et al., 2005), and tomatoes (Mirzaei et al., 2007). Fan et al. (2015) reported that the SCAR primers Bc-f/Bc-r are highly specific for B. cinerea and can be used to differentiate B. cinerea, B. fabae, and B. fabiopsis.
In a preliminary experiment, the antifungal effects of PAA on B. cinerea were assessed by comparing the effects with those of NaOCl using a disc diffusion assay. The concentrations of PAA that inhibited the mycelial growth of B. cinerea were found to be identical to those of NaOCl. After three days of incubation, the inhibition zone was clearly visible around discs treated with PAA or NaOCl at concentrations of 1000 and 2000 ppm (Fig. 2A). However, no apparent inhibition of fungal growth was observed upon a treatment with PAA or NaOCl at concentrations below 1000 ppm until the fourth day after incubation. Mycelial growth began to move closer to the discs on the fifth day; however, the inhibition zones resulting from the PAA treatment were still evident (data not shown).
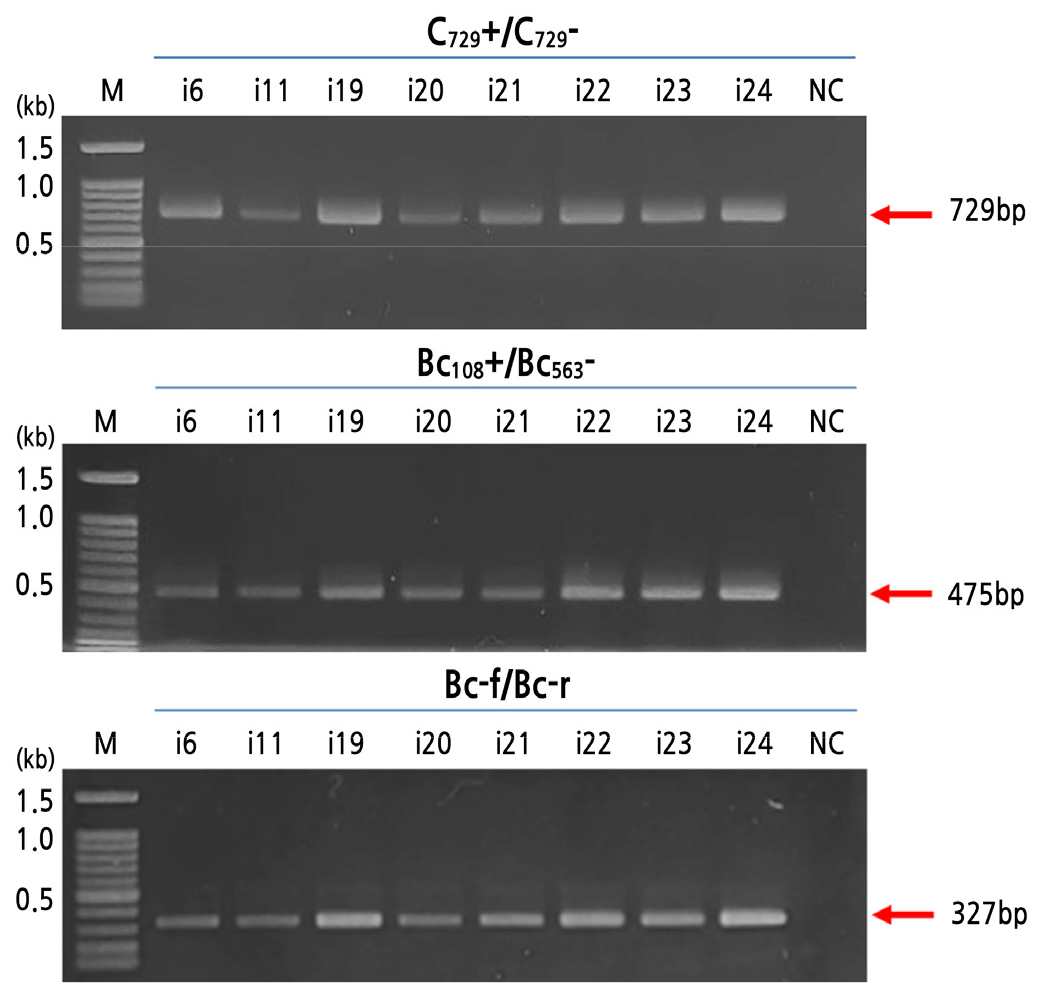
Fig. 1.
PCR amplification of genomic DNA from fungal isolates using SCAR markers specific to Botrytis cinerea. Amplified fragments were resolved by 1% agarose gel electrophoresis. Gel images illustrate PCR products amplified from fungal isolates (lanes 2–9) using the SCAR markers C729+/C729‒ (upper panel), Bc108+/Bc563‒ (middle panel), and Bc-f/Bc-r (lower panel). The fragment size is indicated on the right. Lane M, molecular weight marker (100 bp DNA ladder). Lane NC, negative control (no DNA). The primers used in this study have been previously described by Rigotti et al. (2002), Rigotti et al. (2006), and Fan et al. (2015).
The antifungal activity of PAA was assessed by comparing the mycelial growth of fungi treated with various concentrations of PAA with that of non-treated fungi. As shown in Fig. 2B, PAA concentrations of ≤125 ppm resulted in little or no inhibition of mycelial growth. In contrast, PAA concentrations exceeding 250 ppm completely inhibited mycelial growth until the fifth day after incubation. A previous study (Yang et al., 2022) assessing the antifungal activity of PAA against Cladosporium species also reported that PAA concentrations exceeding 200 ppm are required to inhibit radial mycelial growth.
The effect of PAA on the growth inhibition of B. cinerea was assessed by spraying PAA directly on growing mycelia. Mycelial growth was partially inhibited in plates treated with PAA at concentrations from 1000 to 2000 ppm; however, no inhibition was observed at concentrations of ≤500 ppm (Fig. 2C). Growth inhibition was apparent in plates treated with 8000 or 4000 ppm PAA, with the reduction in mycelial growth being more than 75% greater than that in the control plate.
To assess the susceptibility of B. cinerea to PAA, the fungal metabolic activity was measured by means of a colorimetric WST-1 assay. No differences in metabolic activity were noted between B. cinerea conidia exposed to PAA concentrations of less than 25 ppm and non-treated conidia (Fig. 2D). However, PAA concentrations exceeding 50 ppm inhibited the metabolic activity of conidia. Consequently, yellow-colored formazan did not form.
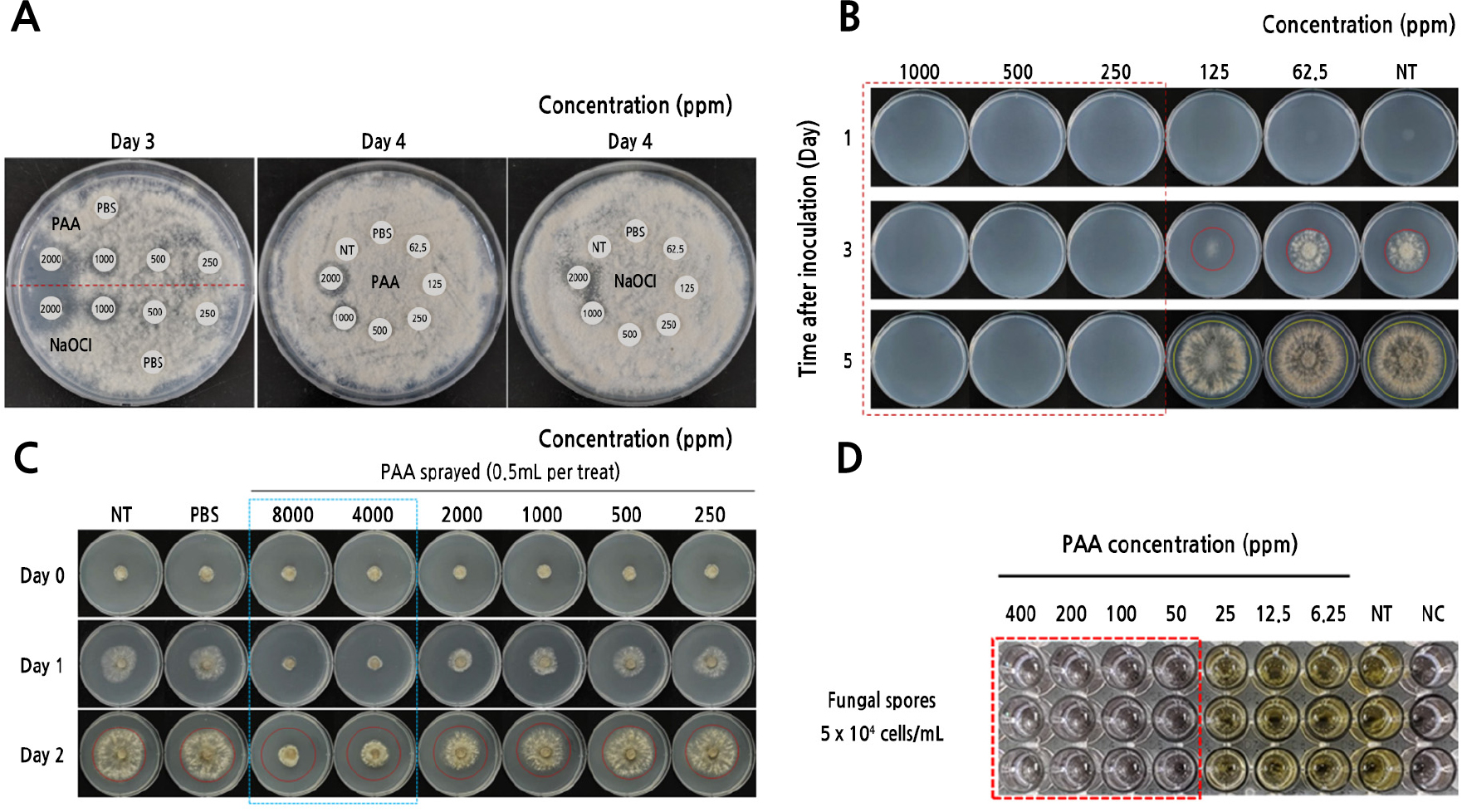
Fig. 2.
In vitro susceptibility of B. cinerea to PAA. To evaluate the antifungal effect of PAA on B. cinerea, various susceptibility tests, in this case a disc diffusion assay (A), a radial mycelial growth assay (B), a spray treatment on growing mycelium (C), and a colorimetric WST-1 assay (D), were conducted. The figures indicate the final concentration of PAA applied to the conidial suspension, growing mycelium, or paper disc. The concentration of PAA required to inhibit fungal growth differed depending on the type of fungal inoculum, the conidial density, and the application mode of the agent. NT, non-treated (no drug added); NC, negative control (media instead of spore suspension).
The growth of B. cinerea was assessed in a 1% malt extract broth containing different concentrations of PAA. As shown in Fig. 3A, PAA did not inhibit the growth of B. cinerea at concentrations ranging from 1.5625 to 12.5 ppm. In contrast, fungal growth was slightly inhibited during 27 h of treatment with 25 ppm PAA, but not thereafter. No measurable growth was observed in the conidia of B. cinerea treated with 50 or 100 ppm PAA over the 72-h incubation period. Moreover, to confirm whether the inhibitory effect of PAA was affected by differences in the inoculum density, PAA treatments were performed at 24 and 48 h after incubation. After the preincubation period, the conidia had formed hyphal fragments (data not shown). The hyphal fragments did not exhibit further growth when exposed to 100 ppm PAA; further growth was not observed even after prolonged incubation with PAA until 96 h, regardless of the time point (i.e., PAA treatment after 24 or 48 h of preincubation) (Fig. 3B and 3C). In the group treated after 24 h of incubation, fungal growth was slightly inhibited by 25 ppm PAA (Fig. 3B). However, compared with the control group, 25 ppm PAA did not cause any noticeable difference in fungal growth in the group treated after 48 h (Fig. 3C). These results indicate that the concentrations of antifungal agents required to inhibit fungal growth depend on the density of the inoculum.
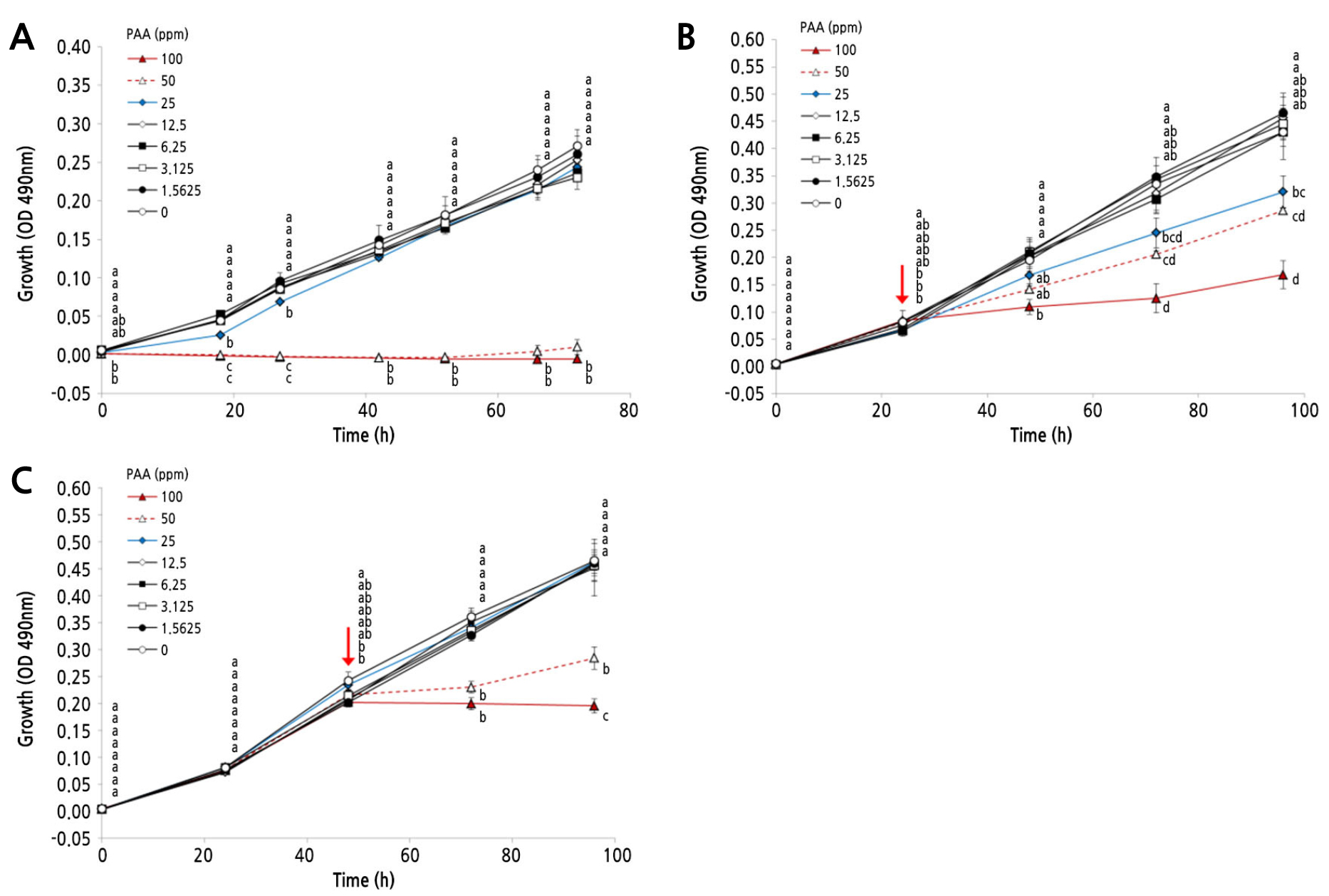
Fig. 3.
Effect of PAA on growth of B. cinerea. Spore suspensions (7.5 × 104 cells/mL) containing different concentrations of PAA (1.5625–100 ppm) were dispensed as 200-µL aliquots into six replicate wells of a 96-well plate (A). The plate was incubated at 24°C, and the growth of B. cinerea was monitored for up to 96 h by measuring the optical density at 490 nm. Red arrows indicate a PAA treatment after 24 h (B) or 48 h (C) of preincubation. Data are presented as the means ± standard deviation (SD). Different letters represent significant differences at p < 0.05.
The results of in vivo susceptibility testing revealed that the concentrations of PAA required for the complete inactivation of fungal growth or metabolic activity were dependent on the density of the inoculum. In a radial mycelial growth test, in which a conidial density of 5 × 105 cells/mL was used, fungal growth was completely inhibited by 250 ppm PAA; however, 50–100 ppm PAA was required to achieve complete inhibition of mycelial growth or metabolic activity when the inoculum density was 5–7.5 × 104 cells/mL. These results are consistent with our previous results (Yang et al., 2022), which demonstrated that the susceptibility of conidia to PAA decreases with an increase in the spore density.
During room-temperature storage, a marked increase was noted in the natural decay of all fruit samples when increasing the storage period, regardless of the PAA treatment (Fig. 4). However, the decay was found to be lower in strawberries treated with 800 ppm PAA. Visual decay began quickly in the control strawberries, with 65.9% of the fruits spoiling after five days of storage. While all four treatment groups exhibited signs of decay after three to four days of storage, the fruits treated with 800 ppm PAA did not exhibit any decay until four days, resulting in approximately 33% less spoilage than that of the untreated control fruits on day 5. PAA concentrations less than 800 ppm were ineffective in reducing the decay incidence in strawberries. The water-treated strawberries exhibited the highest decay incidence, even higher than that noted in the untreated control fruits on the fifth day of storage. As the storage period increased, the shrinkage and water spillage of fruits gradually progressed in all the experimental groups, except in strawberries treated with 400 or 800 ppm PAA. Moreover, a high prevalence of mold contamination was confirmed even inside the package after seven days of storage. On the other hand, strawberries treated with 800 ppm PAA maintained their original size and color throughout the storage period and exhibited a decay incidence of 78.6% on day 7; however, the severity of fungal spoilage in individual fruits remained at a low level. On the seventh day of storage, 96.9% of the control fruits were spoiled, while the decay incidence rates in strawberries treated with 200, 400, and 800 ppm PAA were 96.3%, 87.8%, and 78.6%, respectively.
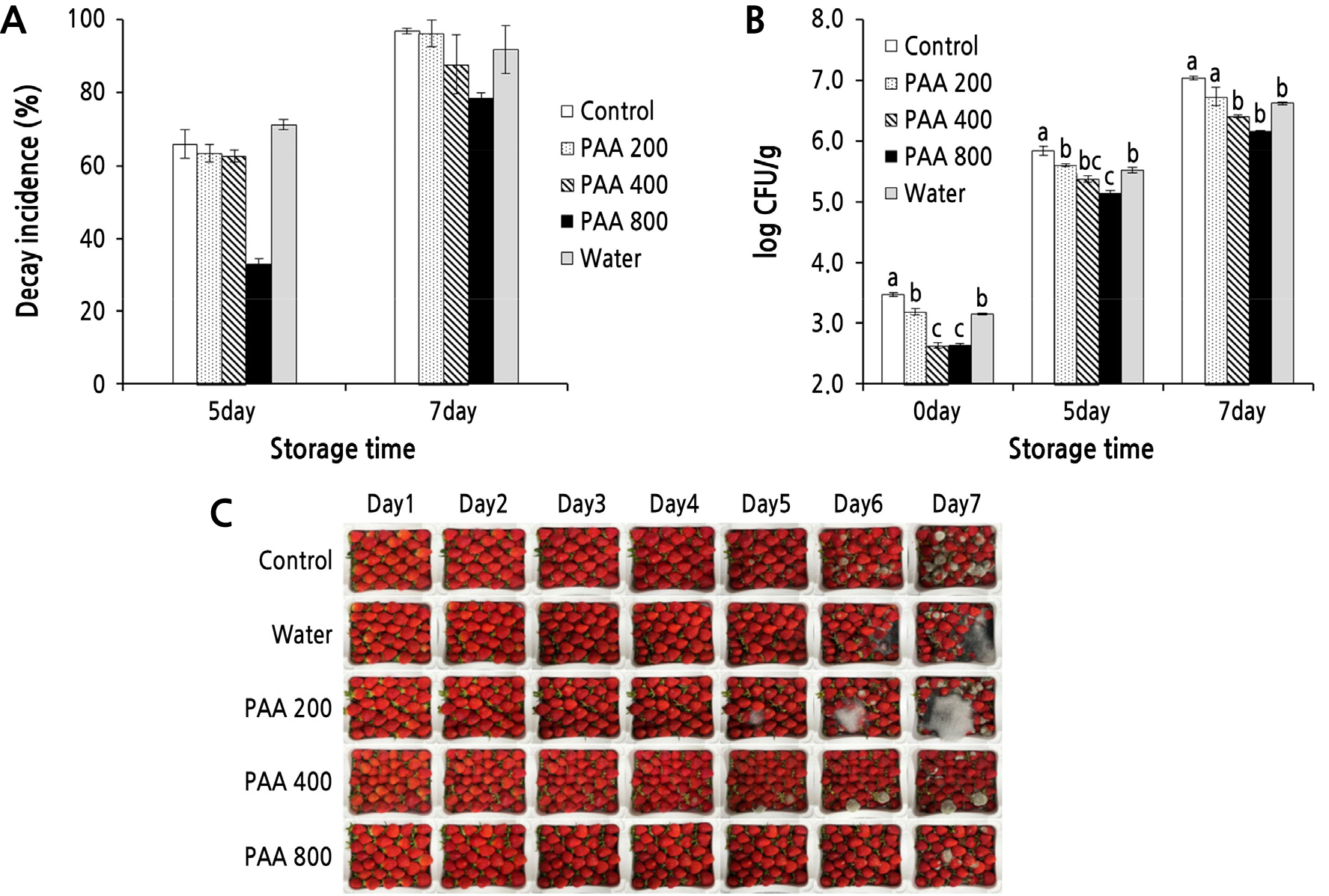
Fig. 4.
Effect of preharvest PAA treatment on the natural decay of strawberries (A) and yeast and mold populations in strawberries (B) during room-temperature storage. One hour before harvest, strawberries were sprayed with PAA at different concentrations (200, 400, and 800 ppm) or with distilled water (DW). Control fruits were left untreated. The treated fruits were packaged in expanded polystyrene (EPS) boxes and then placed at room temperature at 20 ± 2°C and 45–50% relative humidity for up to seven days. The decay incidence and yeast and mold populations were measured on day 0 and after days 5 and 7 of storage. Representative images depicting natural decay that occurred in strawberries from each treatment group (C) were taken during storage at 20°C for up to seven days. Vertical bars represent the SD of three replicates. Mean values followed by different letters within the storage time are significantly different at p < 0.05, as assessed by Duncan’s multiple range test.
The PAA treatment markedly reduced lesion diameters in artificially inoculated strawberries. The efficacy of PAA in controlling gray mold in strawberries was significantly affected by the PAA concentration. When wounded fruits were inoculated with B. cinerea spore suspensions, no visible lesions were noted until two days post-inoculation (dpi). In strawberries treated with 400 ppm PAA without artificial inoculation, no noticeable signs of infection were detected until 5 dpi. In fruits treated with DW, a typical brown lesion appeared at the inoculation site at 3 dpi. However, lesion development was significantly inhibited by the PAA treatment. As shown in Fig. 5, the lesion diameter decreased in a dose-dependent manner in infected strawberries following the PAA treatment. At 3 dpi, the lesion diameter in strawberries treated with 800 ppm PAA was only 3.27 mm, being significantly (p < 0.05) less than that in strawberries treated with DW (9.04 mm). The lesion diameter at 5 dpi in DW-treated strawberries was nearly 1.5 times larger (18.42 mm) than that in strawberries treated with 800 ppm PAA (12.42 mm).
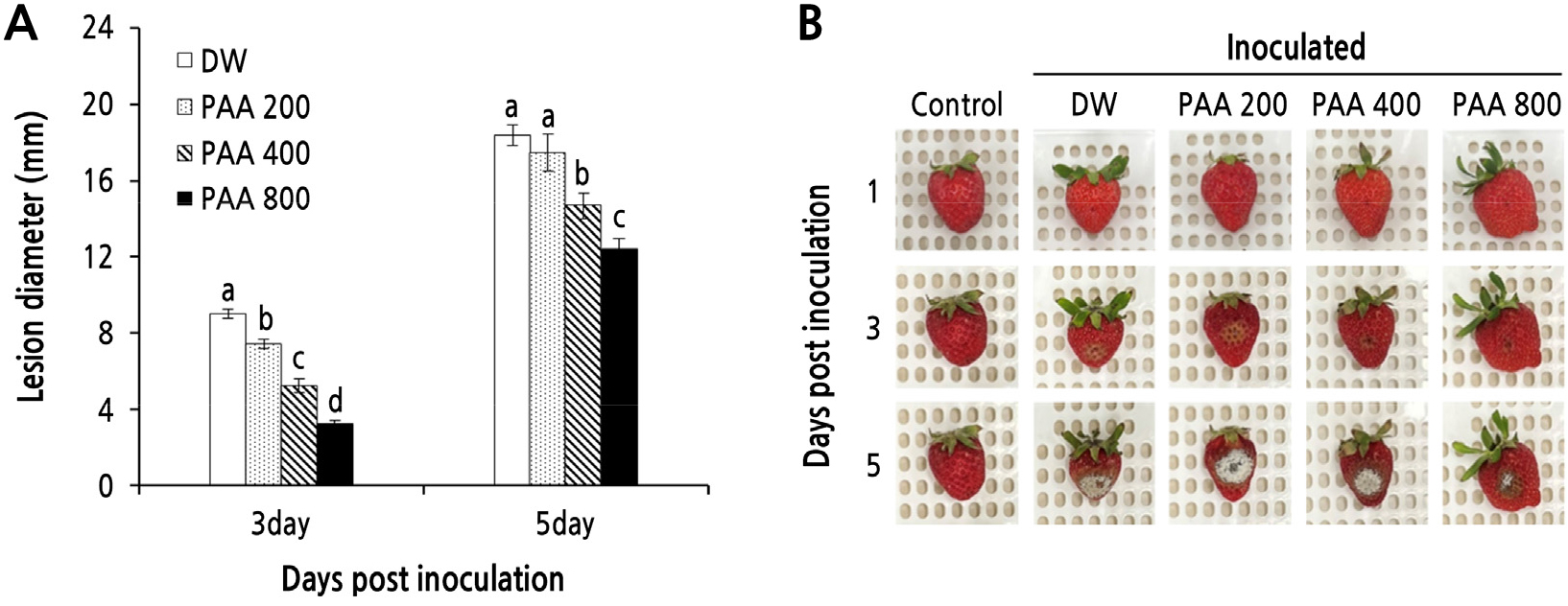
Fig. 5.
Effect of PAA on lesion diameters (A) in strawberry fruits inoculated with B. cinerea. Artificially wounded strawberries were sprayed with PAA at different concentrations (200, 400, and 800 ppm) or with distilled water (DW) and dried for 2 h. The wounded area was then inoculated with 10 µL of spore suspension. Control fruits were left uninoculated. The strawberries were stored at 20°C for five days. Representative images depicting lesion development in strawberries in each treatment group (B) were taken at one, three, and five days post-inoculation (dpi). Vertical bars represent the SD of three replicates. Mean values followed by different letters within the dpi are significantly different at p < 0.05, as assessed by Duncan’s multiple range test.
Dose-dependent reductions in lesion development following a PAA treatment have also been reported in previous studies. When plums inoculated with the conidia of Monilinia laxa were dipped in 500 and 1000 ppm PAA solution for 1 min, their decay rate was reduced by 11.4 and 26.7%, respectively, in comparison with that of control fruits (Mari et al., 1999). In an experiment confirming the effect of PAA on blueberries artificially inoculated with B. cinerea, disease incidence in fruits dipped in 24 and 85 ppm PAA for 45 s was reduced by 12% and 60%, respectively, in comparison with that of undipped fruits (Saito et al., 2021). When assessing the effect of PAA on disease reduction in blueberries, the dipping treatment was found to be superior to a spraying treatment. The duration of the treatment can also affect the inhibition of disease incidence by PAA. Disease incidence in stone fruits artificially inoculated with Rhizopus stolonifer decreased in proportion to the dipping time in a 250-ppm PAA solution, and Rhizopus rot was completely suppressed by a prolonged treatment of 8 min (Mari et al., 2004).
The efficacy of PAA in reducing the microbial load of strawberries was assessed during storage at 20°C. A preharvest application of PAA significantly affected the yeast and mold populations on the fruit surface. The densities of yeasts and molds recovered from strawberries treated with various concentrations of PAA ranged from 2.6 to 3.2 log CFU/g immediately after harvest; propagule densities were lower in the treated fruits than in the untreated fruits (3.5 log CFU/g). The water-treated strawberries had lower initial populations of yeasts and molds than the untreated control strawberries; however, they had a higher microbial load than the PAA-treated fruits, except for those treated with 200 ppm PAA, throughout the storage period. Compared with the control, treatment with 800 ppm PAA caused the maximum reductions (0.9 log CFU/g) in the yeast and mold populations in the fruits. However, the water treatment only induced a reduction of 0.3 log CFU/g. The yeast and mold populations in the strawberries increased during storage in all treatment groups; however, they were lower in the PAA-treated fruits than in the untreated fruits. On the seventh day of storage, the propagule density in control strawberries reached 7.0 log CFU/g, while the propagule densities in strawberries treated with 0 (water), 200, 400, and 800 ppm PAA were 6.6, 6.7, 6.4, and 6.2 log CFU/g, respectively. These results indicate that a preharvest PAA treatment reduces the initial microbial load in strawberry fruits, thereby affecting the natural decay that occurs during storage.
The effect of PAA on the microbial load in fruits has been described previously. The initial population present on the fruit surface was significantly reduced by the preharvest spraying of immature strawberries with 100 ppm PAA (Narciso et al., 2007) or by the postharvest dipping of mangoes in 100 ppm PAA for 3 min (Narciso and Plotto, 2005). Several studies have reported the efficacy of PAAs against various pathogens causing spoilage of agricultural products. However, the antifungal effects of PAA have been found to vary with the exposure time, PAA concentration, and species tested. Mari et al. (1999) assessed the effect of PAA on conidial germination of M. laxa for controlling brown rot in stone fruits and noted complete inhibition of germination after 5 min of exposure to 500 ppm PAA. In contrast, 500 ppm of PAA had no inhibitory effect on P. digitatum, which causes green rot in oranges (Brown, 1987). Smilanick et al. (2014) reported that complete inactivation of spore germination occurred only when P. digitatum conidia were exposed for 10 min to a 0.47% Jet-Oxide solution consisting of 230 ppm PAA and 1250 ppm hydrogen peroxide. Chen et al. (2004) reported a 4-log reduction in P. expansum spore germination after 5 min of exposure to 1% Storox®, which consists of 200 ppm PAA and 2700 ppm hydrogen peroxide. The different concentrations of PAA used for the complete inactivation of fungal pathogens may be due to differences in the susceptibility of the tested species to antifungal agents and differences in the amount of hydrogen peroxide present in the PAA dilutions.
In summary, the preharvest PAA treatment as described here reduced the initial microbial load and thereby delayed the natural spoilage of strawberry fruits during room-temperature storage without changing their size or color. The results obtained from in vitro susceptibility testing revealed that PAA had an inhibitory effect on the mycelial growth and metabolic activity of B. cinerea at concentrations ranging from 50 to 250 ppm. The reductions in the densities of yeasts and molds ranged from 0.3 to 0.9 log CFU/g in strawberries immediately after harvest upon a treatment with 200–800 ppm PAA. The natural decay of strawberries receiving the preharvest spray treatment with 800 ppm PAA was significantly lower than that of the untreated control fruits after five days of storage at 20°C. Furthermore, compared with DW-treated fruits, PAA significantly reduced lesion development caused by artificially inoculated B. cinerea in a dose-dependent manner. These results suggest that a preharvest PAA treatment, particularly using 800 ppm PAA, would be a useful approach to prolong the postharvest quality of strawberries.
In conclusion, our results indicate that a PAA treatment can extend the shelf life of strawberries by reducing their microbial load and thus slowing down their natural decay during storage. However, further studies are warranted to gain a more complete understanding of the mechanisms by which PAAs affect the physicochemical properties of strawberries.