Introduction
Materials and Methods
Plant Materials and Treatments
Measurement of Fruit Quality Attributes
Extraction and Determination of Uronic Acid and Total Sugar Contents
Extraction and Determination of Cell Wall Hydrolase Activities
Statistical Analysis
Results and Discussion
Fruit Quality Attributes
Uronic Acid Content (UAC) and Total Sugar Content (TSC)
Cell Wall Hydrolases (CWH) Activities
Introduction
Apple is an economically important fruit in Korea. It accounts for 20.6% of the total fruit production, ranking as the second largest fruit industry in Korea (USDA, 2017). However, postharvest loss due to fruit softening during storage or shelf life conditions continues to be a problem in the marketplace, leading to economic loss for the growers. Apple is climacteric, and ethylene affects the ripening and senescence process of climacteric fruit, reducing the quality of fruit after harvest and during storage (Bouzayen et al., 2010). Therefore, an ethylene perception inhibitor, 1-methylcyclopropene (1-MCP), used with a low storage temperature would be effective for ethylene inhibition (Asif et al., 2006; Lee et al., 2016). Other factors including the reduction in firmness and titratable acidity, may also be delayed by 1-MCP (DeEll et al., 2002; Watkins, 2006, 2008). However, these favorable outcomes can vary according to the cultivars (Watkins et al., 2000).
The softening of fruit is generally due to the accumulation of osmotic solutes in the cell wall space that leads to a reduction in the cell wall turgidity (Shackel et al., 1991). Pectin is a major component of the middle lamella and primary cell wall structure, and its solubilization can result in decreased firmness (Chen et al., 2015, 2017a, 2017b). Pectin becomes soluble and depolymerized during the ripening process (Huber and O’Donoghue, 1993; Brummell and Labavitch, 1997). This depolymerization is also associated with changes in polyuronides, which are bound to uronic acid and soluble carbohydrates (Huber, 1983; Gross and Sams, 1984; Brummell and Harpster, 2001). In addition, the disassembly of cell wall structure in the ripening fruit tissues is mediated by a large number of enzymes including glycosidases (Fisher and Bennet, 1991). Glycosidases remove the galactosyl and arabinosyl residues from the cell wall (Wei et al., 2010). Yoo et al. (2018, 2019) observed that the activities of cell wall hydrolases (α-galactosidase, β-galactosidase, β-glucosidase, β- arabinosidase, β-xylosidase, and α-mannosidase) are involved in the softening mechanism of apple and peach fruits. These activities are also associated with flesh firmness.
‘Honggeum’ apple is known for its attractive skin color with high quality attributes, and it has excellent cross-compatibility with major apple cultivars such as ‘Fuji’ and ‘Hongro’ (Kim et al., 2008). However, information on the appropriate treatment and storage conditions for maintaining the quality of ‘Honggeum’ apple is still limited. Therefore, it is necessary to find out the storage method with 1-MCP treatment. In this study, we evaluated the fruit performance upon treatment and the delay in fruit softening in relation to cell wall hydrolase activities. The active concentration rate of 1-MCP to maintain the quality of apple fruit is varies according to the cultivar (Watkins et al., 2000); therefore, in this study, two 1-MCP concentrations were used to analyze the effect for cell wall degradation on ‘Honggeum’ apple during cold storage
Materials and Methods
Plant Materials and Treatments
‘Honggeum’ apples were harvested on September 12, 2018 from the Apple Research Institute (Gunwi, Republic of Korea). The harvested fruits were immediately sent to the laboratory of quality crops management, Department of Horticultural Science, at Kyungpook National University, Daegu, Korea. A total of 300 uniform-sized fruits free of physical damage and infections were carefully selected for this study. Fruits were fumigated with two 1-MCP concentrations (0.5 and 1 µL·L-1); 90 fruits of each treatment were sealed in containers for 18 h at 20°C by following the protocol of Watkins et al. (2000). Untreated fruits were used as the control. All fruits were stored together at 0 ± 1°C with 85 ‑ 90% relative humidity for up to 6 months. The assessments were conducted on 15 fruits for each treatment at 1-month intervals after 18 h at 20°C.
Measurement of Fruit Quality Attributes
Internal ethylene concentration (IEC) was measured according to the method of Jung and Watkins (2014) by withdrawing 1 mL of sample gas from the core cavity of apple fruit and injecting it into a gas chromatograph (GC2010; Shimadzu, Kyoto, Japan) activated with a Porapak Q (80/100 1m, Restek, Bellefonte, PA, USA) column. During the measurement, the temperature for injector and flame ionization detector was set at 200°C and 100°C, respectively, with a 90°C oven temperature with 20 mL·min-1 He flow rate. Flesh firmness (Newton/ 11-mm diameter) was assessed by a rheometer (Compac-100II; Sun Scientific, Tokyo, Japan) at three locations on each of the 15 individual fruits around the equatorial regions. The soluble solids content (SSC) was measured in the juice using a refractometer (PR-201α; ATAGO, Tokyo, Japan). Titratable acidity (TA) was recorded using juice samples followed by a reduction method for malic acid (Win et al., 2019), titrating with NaOH to pH 8.1 (DL-15; Mettler Toledo, Zurich, Switzerland). Weight loss was measured based on the decrease in fresh weight of individual fruits before and after storage. Peel color variables (L*, a*, and b*) were taken at three different locations on the sunlit and shaded (background) sides of the 15 fruits by a chroma-meter (CR-200; Minolta, Tokyo, Japan) as described by Kim et al. (2018). During the experiment, flesh tissue samples were frozen in liquid nitrogen and stored at ‑ 80°C for determination of total sugar content, uronic acid content, and cell wall hydrolase activities. Cell wall metabolism was evaluated every 2 months.
Extraction and Determination of Uronic Acid and Total Sugar Contents
The extraction and determination of uronic acid (UAC) and total sugar contents (TSC) were performed according to Yamaki et al. (1979) and Yoo et al. (2018). Frozen tissue samples (10 g) were heated with 100 mL of boiling 80% ethanol for 40 min to remove alcohol-soluble solids and inactivate possible endogenous enzymes, followed by homogenization. The resulting slurry was then filtered using Whatman No#541 filter paper (GE Healthcare Ltd, Buckinghamshire, UK) and washed with boiling ethanol (100 mL, 2 times) and acetone (200 mL, 1 time), respectively. Alcohol-insoluble substance (AIS) was obtained by air-drying the crude cell wall extract overnight at 30°C (Lee and Kim, 2010). The AIS was prepared by extracting air-dried powder (10 mg) with 2 mL sulfuric acid, which was diluted to 50 mL with distilled water.
For the UAC measurement, diluted AIS solution (0.5 mL) was mixed with 3 mL of sulfuric acid and vortexed. The mixture was activated in boiling water for 20 min. Then, 0.1 mL of 0.1% carbazole was added to the mixture, vortexed, and stood for 2 h at 30°C. The final solution was read at 490 nm using a spectrophotometer (UV-1800; Shimadzu, Tokyo, Japan), and D-galacturonic acid was used as a standard.
For the TSC measurement, diluted AIS solution (0.5 mL) was mixed with 0.5 mL of 5% phenol and vortexed. Sulfuric acid (2.5 mL) was then added and it stood for 30 min. The final solution was read at 490 nm using a spectrophotometer, and glucose was used as a standard. Three replicates were used for the measurement of UAC and TSC.
Extraction and Determination of Cell Wall Hydrolase Activities
Cell wall hydrolases were extracted and measured according to Pressey (1983) and Yoo et al. (2018). All of the extraction steps were performed at around 4°C. Frozen tissue samples (50 g) were homogenized with 100 mL of 10 mM sodium phosphate buffer (pH 7.0) including 0.1 g of polyvinylpolypyrrolidone (PVPP). NaCl (8.766 g) was added to the extracted samples and stirred for 3 h. The incubated suspension was centrifuged at 12,000×g for 1 h, and ammonium sulfate was added and stirred overnight. Then, the supernatant was centrifuged at 12,000×g for 1 h. The pellets were collected and dialyzed for 2 d in 10 mM sodium phosphate buffer. The crude extract was collected after centrifuging at 22,000×g for 1 h.
For the measurement of cell wall hydrolase activities (α-galactosidase, β-galactosidase, β-glucosidase, β-arabinosidase, β-xylosidase, and α-mannosidase), the extracted solution (250 µL) and 250 µL of 10 mM sodium acetate buffer (pH 4.0) were added to a test tube. After adding 125 µL of each 2% p-nitrophenoyl-pyranoside, the mixture was incubated at 30°C for 1 h. Then, 1 mL of 1 M sodium carbonate was added to the reacting solution, and the absorbance was read at 410 nm using a spectrophotometer. Three replicates were used for the measurement of cell wall hydrolase activities, and one unit of enzyme was defined as the amount of enzyme that released from 1 mmol of p-nitrophenol per kg per min at 30°C.
Statistical Analysis
All data were analyzed with SPSS software (IBM SPSS Statistics 23; IBM Corp., Armonk, NY, USA). The data were subjected to two-way analysis of variance, and means were analyzed with least significant difference (LSD) test at a significant level p = 0.05. The data were presented as mean ± standard error.
Results and Discussion
Fruit Quality Attributes
Flesh firmness was decreased with all treatments during storage. However, 0.5 and 1 µL·L-1 1-MCP significantly maintained the loss of flesh firmness compared with untreated fruits during cold storage (p < 0.01) (Fig. 1). Similar to flesh firmness, TA also decreased during storage. The loss of TA was also maintained by 0.5 and 1 µL·L-1 1-MCP, which were significantly higher than untreated fruits (p < 0.05) at the end of cold storage (Fig. 1). However, flesh firmness and TA were not different between the two 1-MCP treatments during whole storage at low temperature. Firmness is an important index for determining the maturity and quality of fruit, and its measurement also indicates the softening of fruit during ripening. Fruit acidity is also one of the maturity indicators of the fruit that contributes to taste, and it goes down during storage. In addition, flesh firmness and TA are parameters that are economically important in the marketplaces to determine the quality of fruits and consumer acceptability (Harker et al., 2008). The flesh firmness and TA are closely associated with each other (Win et al., 2019). Additionally, Win et al. (2019) indicated that a longer storage period would result in a greater loss in flesh firmness and TA, especially for fruits without 1-MCP treatment. Apple appears to be one of the crops that respond to 1-MCP treatment during postharvest storage (Watkins, 2008). Bai et al. (2005) reported that 1-MCP is effective in retaining the TA and firmness of apple fruits during air storage or controlled atmosphere storage. The effect of 1-MCP treatment on flesh firmness can vary by concentration rate; however, TA was not affected during cold storage (Watkins et al., 2000). Interestingly, for ‘Honggeum’ apples, this study showed that 1-MCP concentrations affected both firmness and TA, which delayed the maturity and ripening of ‘Honggeum’ apple fruits in cold storage.
Ethylene is important in apple fruit ripening; it directly influences changes in fruit quality, including texture, flavor, and color, during ripening (Yang et al., 2013). Therefore, the internal ethylene concentration (IEC) was measured in this study. Ethylene signaling is based on the biosynthesis genes ACC synthase and ACC oxidase, and the inhibition of ethylene production is associated with inhibited of expression of these two genes (Li et al., 2013; Yang et al., 2013). Jung and Watkins (2014) reported that 1-MCP resulted in a low amount of IEC and also decreased ACC concentration in apple fruit. In addition, 1-MCP also effectively blocks the ethylene responses at the receptor level (Sisler and Serek, 1997; Serek et al., 2006). The IEC of untreated fruits was increased during storage, and both 1-MCP concentrations significantly inhibited the increase in IEC during storage (p < 0.0001) (Fig. 1). Yoo et al. (2016) reported that a higher IEC is closely associated with greater reduction in flesh firmness. In addition, Watkins et al. (2000) reported that the relationship between the IEC and firmness is strongly negatively correlated, and a higher 1-MCP concentration can proportionally delay the rise in IEC; however, the active concentration rate of 1-MCP also depends on the cultivar and storage conditions. In this study, the magnitudes of the IEC and firmness for ‘Honggeum’ apples were not affected by the 1-MCP concentrations.
Weight loss increased with all treatments during storage. In this study, 1 µL·L-1 1-MCP treated fruits delayed the increase in fresh weight loss compared to untreated fruits (p < 0.05), whereas the weight loss of 0.5 µL·L-1 1-MCP-treated fruits was not significantly lighter than untreated fruits during storage (Fig. 1). Therefore, this study showed that the higher 1-MCP concentration delayed the increase in weight loss. The SSC was also increased with all treatments during the early period up to 1 or 2 months of storage, and was maintained or fluctuated slightly after this period. In this study, the SSC of 0.5 µL·L-1 1-MCP-treated fruits was higher than in untreated fruits (p < 0.05) and that of 1 µL·L-1 1-MCP- treated fruits (p < 0.05) during storage (Fig. 1). The weight loss may be associated with the increase in transpiration rate of fruits, and the delay in weight loss may be attributed to the blocking of ethylene receptors by 1-MCP (Mahajan et al., 2010). However, 1-MCP treatment can result in lower, higher or similar SSC compared with untreated fruits during storage (Watkins et al., 2000; DeEll et al., 2002), which was observed only in 0.5 µL·L-1 1-MCP-treated fruits. In addition, a strong positive correlation between weight loss and SSC was reported in both untreated and 1-MCP-treated fruits (Win et al., 2019). In this study, weight loss and SSC were more affected by 1 µL·L-1 1-MCP than 0.5 µL·L-1 1-MCP, compared with untreated fruits.
Fruit peel color variables (L*, a*, and b*) on both the sunlit and shaded sides were evaluated. Color values (L* and b*) of the shaded side were higher than that of the sunlit side at harvest time and during cold storage (Fig. 2). However, color values were insignificant on the sunlit side. On the shaded side, the a* of untreated fruits was decreased significantly at 3 and 4 months, compared with that of fruits treated with 0.5 µL·L-1 1-MCP (Fig. 2). Lee et al. (2016) reported that lightness (L*) was not different in the stem-end, equatorial, and calyx-end regions for 6 months of ‘Royal Gala’ apple storage among 1-MCP concentrations. However, 1-MCP delays the loss of background peel color and chlorophyll degradation of fruit, and the tissue of apple fruits on the shaded side was more vulnerable to alterations during storage, similarly observed in ‘Gamhong’ apple (Saftner et al., 2003; Kim et al., 2018) and in this study.
Uronic Acid Content (UAC) and Total Sugar Content (TSC)
Fruit softening may be primarily attributed to changes in cell wall components and the middle lamella. Pectin is a major component of middle lamella and the primary cell wall, which is generally composed of a neutral sugar-acidic sugar relationship (Gross, 1983; Gross and Sams, 1984; Manrique and Lajolo, 2004). Pectin is rich in polyuronides and the solubilization of polyuronides during fruit ripening is associated with the depolymerization of pectic substances in the cell wall, resulting in loss of firmness (Huber, 1983; Brummell et al., 2004). The cell wall is composed of cellulose microfibrils, which are depolymerized to sugar forms by the action of cell wall-degrading enzymes during ripening (Brummell et al., 2004). Therefore, the UAC and TSC were measured to reveal the involvement of these two contents in degradation of cell wall metabolism. The UAC was decreased with all treatments; however, untreated fruits had the lowest UAC in fruits stored for six months, and the amounts in fruits with both 1-MCP treatments were not significantly different during storage (Fig. 3). The decline in the amount of uronic acid content may be due to the loss of polyuronides in the cell wall during the ripening period (Brummell et al., 2004), similarly observed in this study. The TSC of 0.5 µL·L-1 1-MCP-treated fruits increased at 6 months of storage, which was significantly higher than 1 µL·L-1 1-MCP treated fruits (Fig. 3). Yoo et al. (2018) observed the solubilization of UAC and TSC during softening-related cell wall degradation in ‘Fuji’ apples. The release of UAC and TSC from cell wall materials during fruit softening is known, and the increased solubilization of these contents is correlated with cell wall-degrading enzyme activities (Martin-Cabrejas et al., 1994; Lazan et al., 1995). Therefore, this study showed the important role of UAC and TSC in degradation of the cell wall structure during fruit ripening. However, in this study, the solubilization of UAC and TSC in the cell wall structure was observed more in untreated fruits and fruits treated with a lower concentration than a higher concentration of 1-MCP. This indicates that cell wall metabolism is more affected by the 1 µL·L-1 concentration than 0.5 µL·L-1 during apple ‘Honggeum’ fruit storage.
Cell Wall Hydrolases (CWH) Activities
The activities of CWH (α-galactosidase, β-galactosidase, β-glucosidase, β-arabinosidase, β-xylosidase, and α- mannosidase) were measured during storage (Fig. 4). Most CWH, except β-galactosidase of control fruits, showed increasing and then decreasing activities during storage, but the CWH activities were reduced by 1 µL·L-1 1-MCP, compared with untreated fruits from 4 to 6 months of storage. In addition, 1 µL·L-1 1-MCP-treated fruits exhibited lower CWH activities at 6 months of storage compared with 0.5 µL·L-1 1-MCP (Fig. 4). Therefore, this study demonstrated that the CWH activities were more affected by higher 1-MCP concentrations. To reveal the action of enzymes involved in the fruit softening mechanism of apple, the activities of six cell wall-degrading enzymes were measured in this study. β- Galactosidase and β-arabinosidase are responsible for cell wall integrity, which is associated with the loss of galactosyl and arabinosyl residues from cell wall polymers, leading to loss of firmness (Vicente et al., 2007; Payasi et al., 2009; Chen et al., 2015). Vicente et al. (2007) additionally reported that α-mannosidase and α-galactosidase are a class of glycosidase that acts on short-chain oligosaccharides. Also, β-glucosidase and β-xylosidase are the enzymes involved with degradation of hemicellulose and cellulose microfibrils of the cell wall (Wu et al., 2018). Similarly, the increase in CWH activities during storage was also observed for ‘Fuji’ apples and ‘HetsalHaunkeybee’ peach (Yoo et al., 2018, 2019), as reported in this study. Furthermore, the CWH activities were strongly correlated with the loss of firmness (Yoo et al., 2019) and closely related with ethylene production (Chang et al., 2017). This study confirmed that the softening of ‘Honggeum’ apple is mainly due to the changes in CWH activities and the delayed softening by 1-MCP may be partially due to the relatively lower activities of CWH.
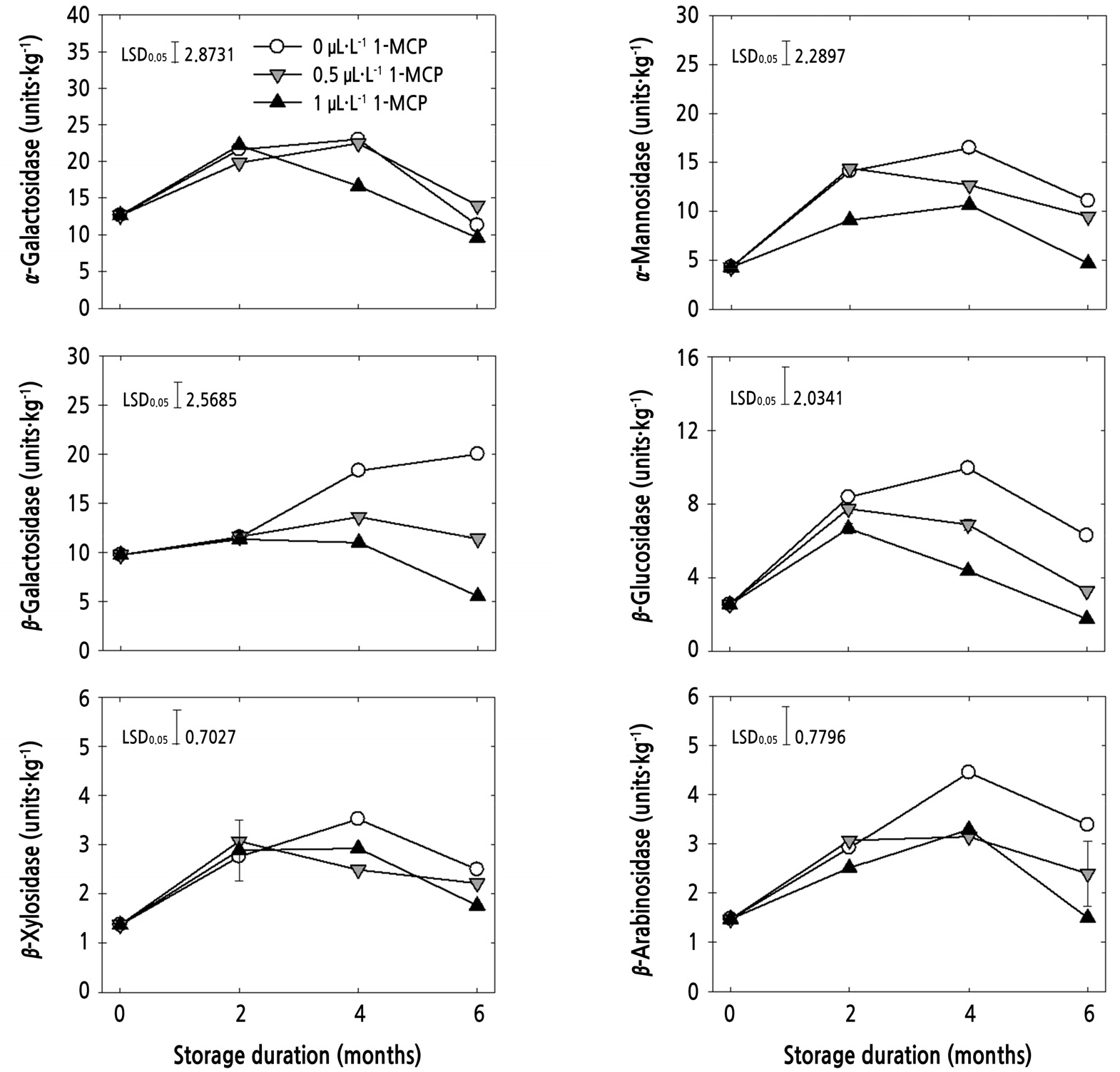
Fig. 4
Cell wall hydrolase activities (β-galactosidase, α-galactosidase, β-glucosidase, β-arabinosidase, β-xylosidase, and α- mannosidase) of ‘Honggeum’ apples treated with 1-MCP at harvest and stored at 0°C for up to 6 months. Data are presented as means ± standard error (n = 3). One unit is the amount of enzyme activity that released 1 mmol of p-nitrophenol per kg per min at 30°C.
In conclusion, similar to 0.5 µL·L-1 1-MCP, 1 µL·L-1 1-MCP treatment retained fruit quality; however, the effect of 1 µL·L-1 1-MCP concentration on cell wall metabolism was much greater than that of 0.5 µL·L-1 1-MCP in delaying the softening of ‘Honggeum’ apple fruits during cold storage.