Introduction
Materials and Methods
Study Site
Experimental Design
Measurement of the Photosynthetic Pigment Content
Measurement of Gas-Exchange Parameters
Measurement of Chlorophyll Fluorescence
Statistical Analysis
Results
Photosynthetic Pigments Content
Gas-Exchange Parameters
Chlorophyll Fluorescence Parameters
Correlation Analysis of the Photosynthetic Pigment Content and Gas Exchange and Chlorophyll Fluorescence Parameters
Discussion
Introduction
As a tree species of the Rutaceae family, Zanthoxylum armatum is one of the most promising and economically significant species in southwest China. It is also known as “green pepper” due to its green fruit. Z. armatum has a long cultivation history in China, has important economic and ecological value, and is widely distributed in most parts of southwestern China and Southeast Asia (Feng et al., 2017). It is commonly used in Chinese cooking and also is an essential ingredient in Sichuan hot pot due to its special numbing flavor. Furthermore, it has pharmacological effects, such as antibacterial, anaesthetic, insecticidal, larvicidal, anti-inflammatory and analgesic effects (Nooreen et al., 2019). Moreover, it is vital in rural revitalization and reforestation projects due to its good soil and water conservation functions along with the ability to not only survive harsh environments but also increase farmers’ incomes in poor areas (Xiang et al., 2016). However, Z. armatum is highly sensitive to waterlogging stress, which reduces photosynthesis and plant growth and yield. Therefore, selecting an effective measure to improve the photosynthesis of seedlings under waterlogging stress is crucial for Z. armatum growth.
Among the most widespread abiotic types of stress in plant life, waterlogging plays a major role (Zhao et al., 2018). Global climate change is causing an uneven distribution of precipitation, causing excessive rainfall and frequent waterlogging events. Waterlogging stress has become a serious problem, especially in poorly drained soils or lowlands, thereby affecting crop growth and yield globally (Phukan et al., 2016). Photosynthesis is the most critical primary plant physiological process that is affected by waterlogging. Photosynthetic reduction due to waterlogging is closely linked to several factors, including decreased chlorophyll biosynthesis (Ashraf et al., 2011), a decreased CO2 supply due to stomatal closure (He et al., 2018), a damaged photosynthetic apparatus (Ma and Guo, 2014) and the non-photochemical dissipation of heat energy (Yuan et al., 2014). Chen et al. (2022) proposed that the CO2 uptake, photosystem II maximum quantum efficiency (Fv/Fm) and electron transfer rate (ETR) of sacha inchi (Plukenetia volubilis L.) seedlings were significantly reduced after waterlogging stress. Furthermore, Li et al. (2020) found that the transpiration rate (Tr), net leaf photosynthetic rate (Pn) and stomatal conductance (Gs) of kiwifruit (Actinidia sp.) under waterlogging conditions all would decrease as the waterlogging time increases. Thus, it is necessary to find an effective way to enhance the photosynthetic efficiency of Z. armatum under waterlogging stress.
Salicylic acid (SA) is one of the most abundant and widely used hormones in plants. It is involved in the physiological and developmental metabolism of plants, including the regulation of abiotic stress. Therefore, SA is usually used as exogenous plant hormone (Iqbal et al., 2022; Wang et al., 2022) Various forms of abiotic stress can be mitigated with SA, including drought (Cha et al., 2020; Munsif et al., 2022), salinity (Fariduddin et al., 2018), heat (Shi et al., 2006), cold (Li and Wang, 2021) and heavy metals (Ahmad et al., 2011). According to studies of grapes (Vitis vinifera L.) and basil (Ocimum basilicum L.), SA plays a crucial role in regulating the photosynthetic characteristics of these plants during adverse environmental stress (Li and Wang, 2021; Da Silva et al., 2022a). Furthermore, Wang et al. (2021) reported that photosynthesis in maize was improved by SA application under waterlogging stress by increasing the Pn, Gs, Tr, Fv/Fm, actual quantum efficiency (ΦPSII) and non-photochemical quenching coefficient (NPQ) of seedlings under waterlogging stress. Similarly, Zhu et al. (2020) found that the Pn, Gs, Tr and intercellular CO2 concentration (Ci) of peony (Paeonia suffruticosa Andr.) decreased under varied waterlogging conditions, while a SA treatment could increase these parameters. Therefore, these findings suggest that the application of SA may provide a strategy by which to mitigate photosynthetic damage to plants under waterlogging stress.
The current work aimed to study the photosynthetic response of Z. armatum leaves after an exogenous SA application under waterlogging stress. We tested the following hypotheses: (1) Waterlogging stress can cause photosynthetic changes such as reductions of the photosynthetic pigments content, net photosynthesis, transpiration and chlorophyll fluorescence of Z. armatum seedlings, ultimately severely impacting the plant. (2) An exogenous SA application can alleviate waterlogging stress-induced damage and improve the waterlogging tolerance of Z. armatum seedlings. Considering these hypotheses, this study can provide a reference for further explorations of the SA-induced mechanism of photosynthesis regulation and provide a theoretical basis for the cultivation management of Z. armatum seedlings.
Materials and Methods
Study Site
The experiment was conducted in a greenhouse at Sichuan Agricultural University (30°42'N, 103°52'E) in China. The average elevation and annual temperature were 580 m and 17.2°C, respectively, and the average temperature in August is 23°C–32°C.
Experimental Design
Here, pest- and disease-free Z. armatum seedlings 20 cm tall with nearly identical growth were selected as the experimental material and were planted in polyethylene pots (19 cm × 18 cm), each containing 3 kg of soil. The soil used for the experiments was obtained from the cultivated layer (0–20 cm) of agricultural fields, shade dried, all residues removed, passed through a 5 mm sieve and mixed thoroughly. The initial properties of the soil samples were as follows: 26.2% sand, 63.5% silt, 10.3% clay, 31.2% field water capacity (FWC), pH 6.74, organic matter - 12.147 g·kg-1, total N - 0.563 g·kg-1, alkali-hydrolysable N - 65.4 mg·kg-1, available P - 27.5 mg·kg-1 and available K - 112.1 mg·kg-1.
In August of 2021, the experiment was established with twelve treatments. SA included three concentrations (including 0, 1 and 2 mM). The waterlogging time included waterlogging for 0 days (CK), 1 day (W1d), 2 days (W2d) and 3 days (W3d) after a SA application by root irrigation. There were three replicates for each treatment, and nine similar growth seedlings were selected for each replicate. The pots with the Z. armatum seedlings were placed in non-porous plastic pots (25 cm × 25 cm) and filled with water until the root neck reached a water level of 3 cm.
Measurement of the Photosynthetic Pigment Content
In the waterlogged state, the fully expanded third leaf of each treatment seedling was collected, fresh leaves (0.02 g) immersed in a 10 mL acetone-ethanol mixture and soaked in the dark for 48 h until the leaf was completely discolored. The extracts were measured at wavelengths of 663 nm, 645 nm and 470 nm. The chlorophyll (Chla, Chlb, totalChl) and carotenoid (Car) contents were calculated according to the description of Arnon (1949).
Measurement of Gas-Exchange Parameters
A portable photosynthesis system (Li-6800; LI-COR, Inc., Lincoln, NE, USA) was used to determine the net Pn, Gs, Ci and Tr between 9:00 and 11:00 am. WUE was calculated as WUE = Pn/Tr. Based on earlier work (Shi et al., 2021), the measurement conditions were maintained at a photosynthetic photon flux density (PPFD) of 1000 µmol·m-2·s-1 and CO2 concentration of 400 µmol·mol-1, a temperature of 27 °C, a gas flow rate of 500 µmol·s-1 and relative humidity of 60%.
Measurement of Chlorophyll Fluorescence
A chlorophyll fluorescence imaging system (Technologica, UK) was used to measure the chlorophyll fluorescence on the same leaves used in the gas exchange test. The PPFD was set to 1000 µmol·m-2·s-1. To ensure that the PSII reaction center is completely open, all samples were adapted to the dark for at least 30 minutes prior to the measurement. The Fo, Fv/Fm, ΦPSII, Fv'/Fm' and NPQ values of the leaves were measured using a chlorophyll fluorescence imager. The ETR was then calculated as ETR = PPFD × ΦPSII × 0.84 × 0.5 (Correia et al., 2021).
Statistical Analysis
The correlation coefficients between the photosynthetic pigment content, gas-exchange parameters and chlorophyll fluorescence parameters were calculated by determining the Pearson’s correlation. Statistical analysis of the data was performed using IBM SPSS 20.0, and a two-way analysis of variance (ANOVA) was used to analyze the significance of the differences between the waterlogging treatment and the SA treatment. The graphs were plotted with GraphPad Prism 8 and R Studio software.
Results
Photosynthetic Pigments Content
Fig. 1 shows the effects of the addition of SA on the content of the photosynthetic pigments of Z. armatum under waterlogging stress. Compared with CK, the Chl a content decreased significantly (p < 0.05) by 6.3%, 11.8% and 9.7% after 1 d, 2 d and 3 d of waterlogging, respectively (Fig. 1A). The total Chl and Car content trends were similar to that of Chl a. The total Chl and Car content decreased gradually with an increase in the waterlogging time, finally reaching the lowest value after 3 d of waterlogging (Fig. 1C and 1D). However, the SA application was found to be able to inhibit the reduction of the content of photosynthetic pigments effectively. The SA treatment significantly increased the Chl a, total Chl and Car contents (p < 0.05) as compared to the 0 mM SA treatment, and the 1 mM SA treatment was more effective. Furthermore, although the exogenous SA application increased the Chl b content, the different treatments did not differ significantly in this regard (p > 0.05, Fig. 1B).
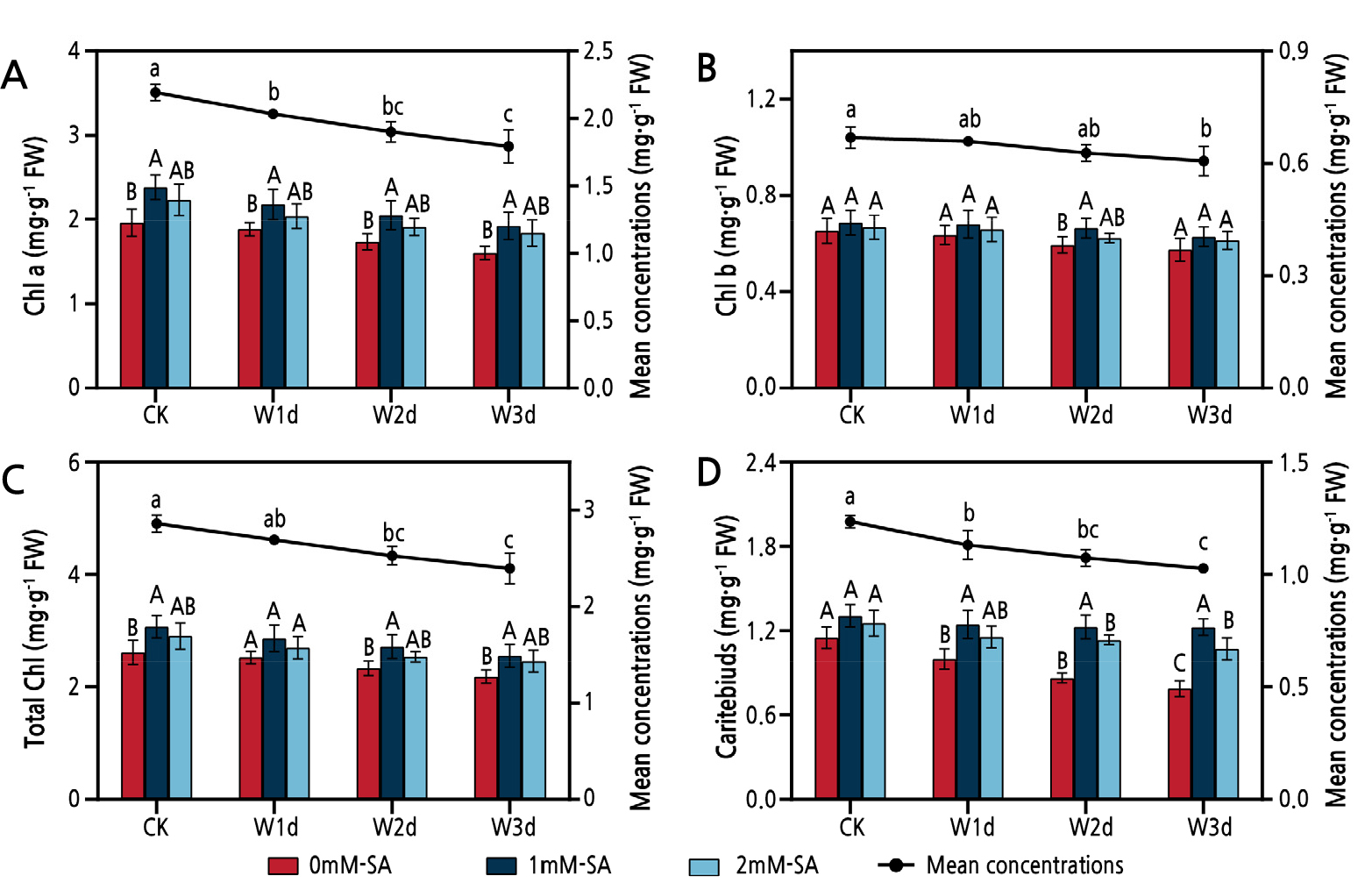
Fig. 1.
Effects of waterlogging and salicylic acid treatments on the Chl a (A), Chl b (B), Total Chl (C) and Car (D) contents of Z. armatum. Vertical bars show the standard deviation (SD) of the means (three replicates). Different uppercase letters denote statistically significant differences between the different salicylic acid treatments, while different lowercase letters denote statistically significant differences between days of waterlogging according to Duncan's test at the level of p < 0.05.
Gas-Exchange Parameters
Waterlogging stress significantly affects the gas exchange parameters (Fig. 2). The Tr, Pn, Gs and WUE outcomes exhibited a declining trend from 1 to 3 d of waterlogging, with corresponding decreases of 30.2%, 41.8%, 39.0% and 16.6%, while Ci showed an upward trend, recording an increase of 11.8%. The SA (1 mM and 2 mM) application alleviated the waterlogging-stress-induced decrease in the gas exchange parameters. As compared to 0 mM SA, the 1 mM SA treatment significantly increased Tr, Pn, Gs, and WUE (p < 0.05). Meanwhile, this treatment significantly reduced (p < 0.05) Ci under the waterlogged conditions.
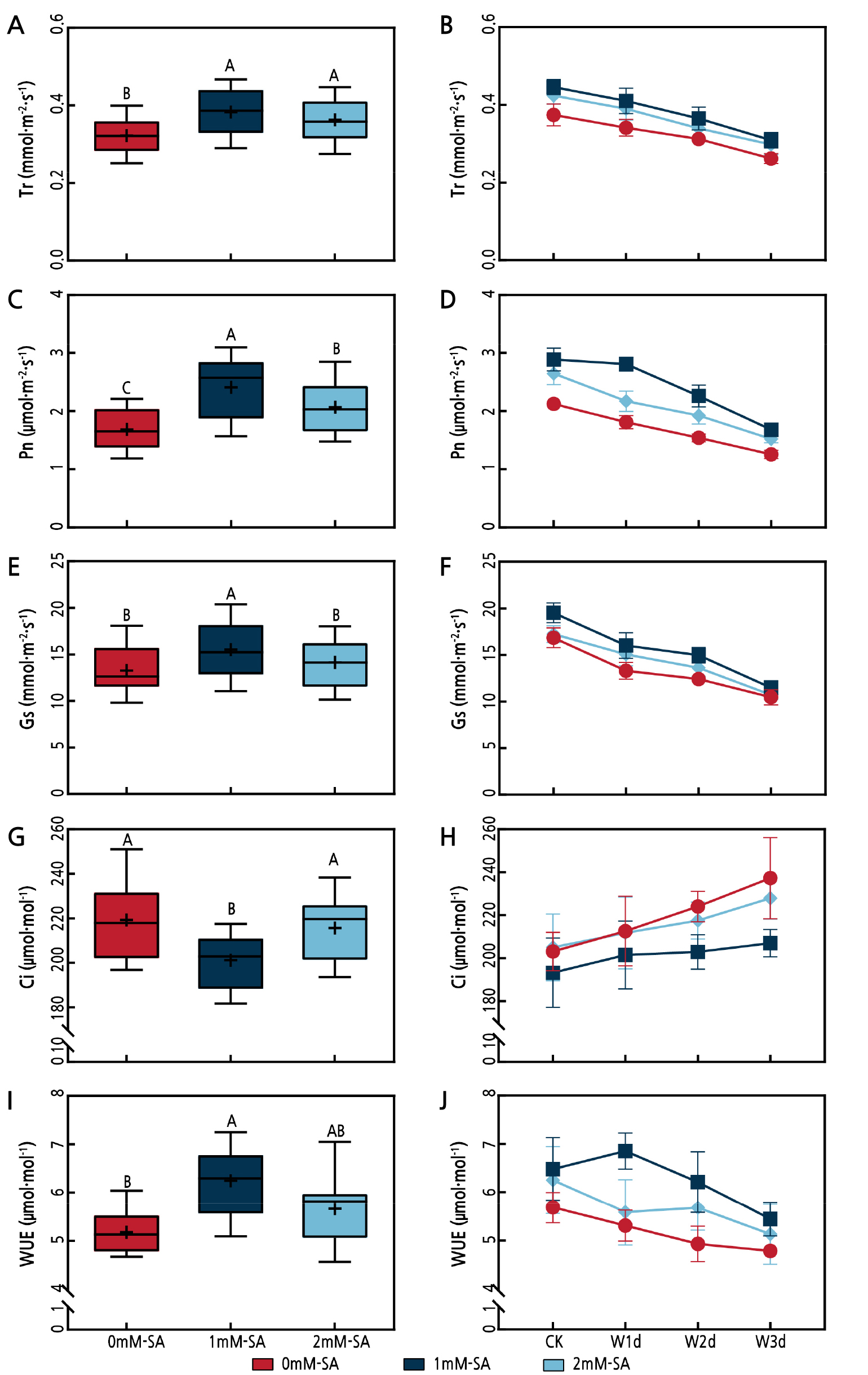
Fig. 2.
Effects of waterlogging and salicylic acid treatments on the Tr (A, B), Pn (C, D), Gs (E, F), Ci (G, H) and WUE (I, J) outcomes of Z. armatum. In the box plot, the positive sign represents the mean of the treatment (n = 12), and the line and vertical bars reflect the median line and 95% confidence intervals, respectively. In the line chart, the points and vertical bars represent the mean ± SD (three replicates). Different uppercase letters denote statistically significant differences between the different salicylic acid treatments according to Duncan's test at the level of p < 0.05.
Chlorophyll Fluorescence Parameters
The Fv/Fm, ΦPSII, Fv'/Fm' and ETR outcomes exhibited a declining trend with an increase in the waterlogging time, whereas Fo and NPQ showed an upward trend (Fig. 3). As compared with CK after 1 d, 2 d and 3 d of waterlogging, Fv/Fm decreased by 4.8%, 10.0% and 21.7%, respectively (Fig. 3A), and ΦPSII decreased by 10.0%, 19.9% and 34.0%, respectively (Fig. 3D). SA noticeably affected the chlorophyll fluorescence parameters. As compared with CK after 3 d of waterlogging, Fv/Fm and ΦPSII increased by 27.5% and 12.7% for the 1 mM SA treatment and by 14.9% and 7.71% for the 2 mM SA treatment, respectively. Moreover, the SA treatment noticeably reduced Fo and NPQ (Fig. 3B and 3C).
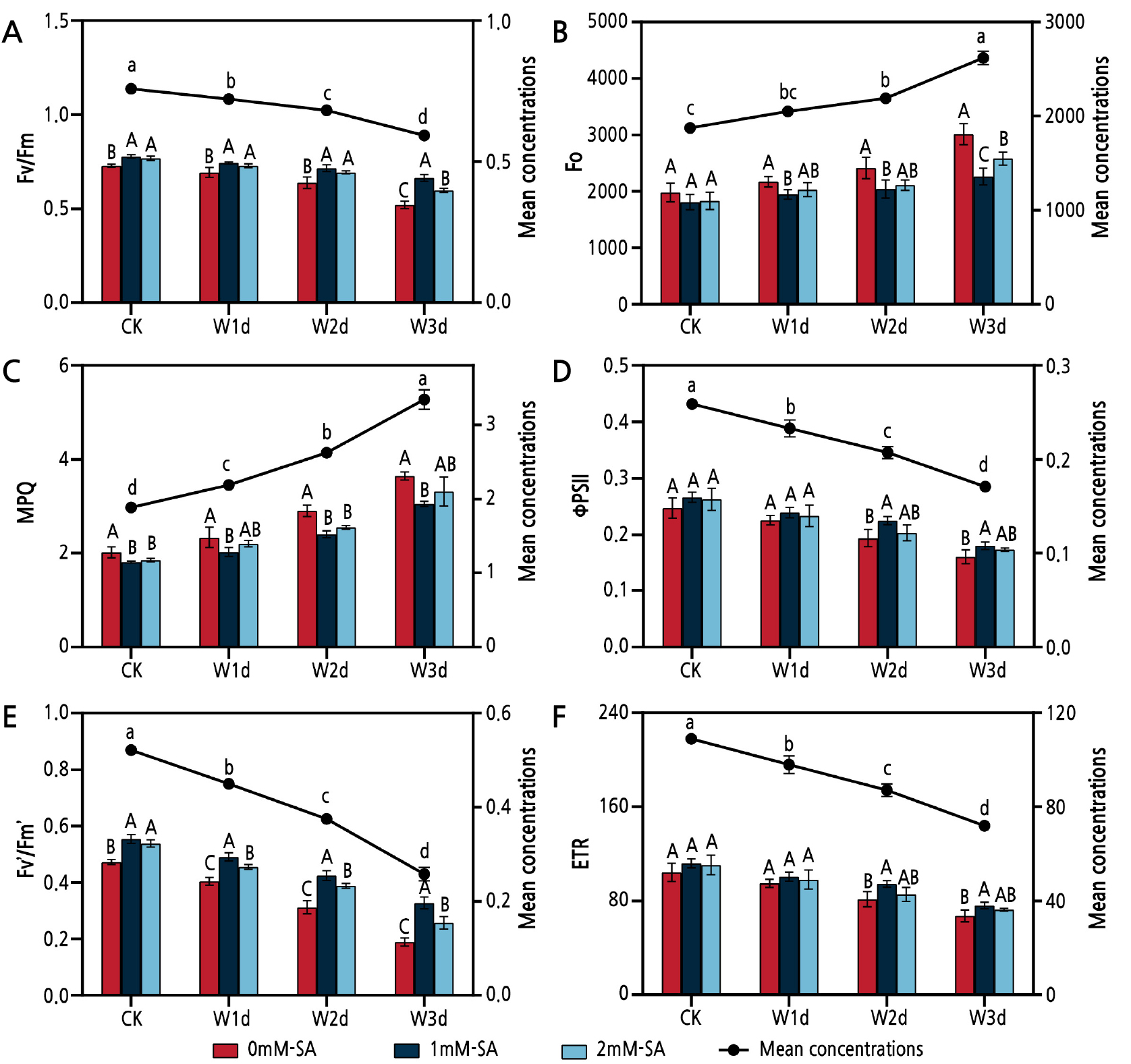
Fig. 3.
Effects of waterlogging and salicylic acid treatments on the Fv/Fm (A), Fo (B), NPQ (C),ΦPSII (D), Fv’/Fm’ (E) and ETR (F) outcomes of Z. armatum. Vertical bars show the SD of the means (three replicates). Different uppercase letters denote statistically significant differences between the different salicylic acid treatments, while different lowercase letters denote statistically significant differences between the days of waterlogging according to Duncan's test at the level of p < 0.05.
Correlation Analysis of the Photosynthetic Pigment Content and Gas Exchange and Chlorophyll Fluorescence Parameters
The correlations among the photosynthetic parameters under waterlogging stress are shown in Fig. 4. NPQ and Fo were significantly correlated with Ci (p < 0.01). In contrast, they showed a significant negative correlation with the other gas exchange and chlorophyll fluorescence parameters. Pn was significantly and positively correlated with Chl a, Chl b, total Chl, Gs, WUE, Fv/Fm, ΦPSII, Fv'/Fm' and ETR (p < 0.01). Fv/Fm was significantly and positively correlated with ΦPSII and ETR (p < 0.01).
Discussion
Globally, plants are subject to recurring rainfall and flooding conditions, which further limits the growing environment for waterlogging-sensitive plants (Xu et al., 2022). The effect of waterlogging on plant growth and photosynthesis is gradually aggravated (Hu et al., 2022). A number of strategies were developed in previous studies to reduce the adverse effects of waterlogging on plants, such as exogenous applications of SA (Wang et al., 2021), melatonin (Gu et al., 2020), 24-epibrassinolide (Ma and Guo, 2014) and ethylene (Kim et al., 2018). Frequent waterlogging stress has increasingly threatened the growth and development of Z. armatum in recent years (Zhou et al., 2021). In this study, although waterlogging stress significantly affected the photosynthetic capacity, the application of SA mitigated it and enhanced the photosynthetic efficiency, thus improving the waterlogging tolerance of Z. armatum. In previous studies of salicylic acid reactions, concentrations have typically ranged from 0.1 mM to 10 mM (Zhu et al., 2020; Wang et al., 2021; Errazuriz-Montanares et al., 2023). In the first preliminary test here, we selected concentrations of 0, 0.5 and 1 mM, and the results indicated that the resistance improved with an increase in the concentration. The concentration of 2 mM was added during the second preliminary test, and these results showed that 1 mM was more effective against waterlogging than 2 mM. Thus, the present concentration levels were selected for use in a formal experiment. Despite the progress made here, the optimal concentration of salicylic acid may need to be researched further.
Photosynthetic pigments play an important role in photosynthesis by converting light energy into chemical energy, but waterlogging stress generally causes critical photosynthetic pigment damage. Chlorophyll absorbs and transmits light energy and is directly related to the light energy conversion of photosynthesis. Meanwhile, carotenoids act as antioxidant single-oxygen scavengers and protect the photosynthetic apparatus from damage by reactive oxygen species. Therefore, the content of photosynthetic pigments is usually used as a stress marker (Allel et al., 2018). In this study, waterlogged Z. armatum seedlings exhibited lower chlorophyll and carotenoid contents (Fig. 1). Ashraf et al. (2011) and Saikia et al. (2021) published similar results for cotton (Gossypium hirsutum L.) and rice, respectively. As a result, chlorophyll decomposition is likely responsible for the reduced levels of photosynthetic pigments under waterlogging stress. Nevertheless, it was observed in the present study that an exogenous SA application significantly increased the chlorophyll and carotenoid contents, most likely due to the ability of exogenous SA to promote chlorophyll biosynthesis effectively and improve the antioxidant function. This was consistent with previous findings demonstrating that SA effectively protects the chlorophyll and carotenoid contents under salinity stress conditions (Dehnavi et al., 2022). Thus, an exogenous SA application could effectively promote the chlorophyll and carotenoid contents of Z. armatum seedling leaves. Previous studies found that the beneficial effects of SA on photosynthesis also depend on the concentration of the solution (Da Silva et al., 2022b). Çag et al. (2009) showed that a 1000 µM SA treatment (high concentration) induced a decrease in the chlorophyll and carotenoid contents, while a 2 µM SA treatment (low concentration) significantly increased these levels. Similarly, Nazar et al. (2011) found that low concentrations of salicylic acid (0.1 mM, 0.5 mM) promoted photosynthesis and growth of mung bean by increasing the photosynthetic pigment content and stomatal conductance, whereas high concentrations of salicylic acid (1.0 mM) showed inhibitory effects. This may be related to the fact that SA is a substance chemosensitive to phenolic acid, and at high concentrations can have a detrimental effect on plant photosynthetic pigments and cystoid membranes, among other factors (Jung et al., 2004; Vicente et al., 2011; Janda et al., 2014). The promoting or inhibiting effect of the SA concentration varies from species to species. Furthermore, our results are also similar to those in previous studies, further demonstrating that 1 mM is a more suitable concentration for Z. armatum.
Photosynthesis, as a process highly sensitive to stress, will be significantly affected by waterlogging (Zhang et al., 2017). Gaseous exchanges in the leaves during photosynthesis can be considered as an effective non-destructive parameter for characterizing the plant abiotic stress response (Najar et al., 2019). The present study found that waterlogging stress significantly decreased Pn, Gs, Tr and WUE of Z. armatum leaves while increasing Ci (Fig. 2). The reductions in Gs and Tr suggest that waterlogging stress reduces stomatal opening, leading to reduced transpiration in the leaves, which in turn affects CO2 uptake and water loss (Zeng et al., 2020). The rise in Ci indicates that the CO2 assimilation capacity, photosynthetic pigment content and PSII reaction center activity of the leaves may undergo damage (Sharma et al., 2022). This is consistent with reports by Wu et al. (2014) and Gao et al. (2021). A previous study found that SA helped to improve plant gaseous exchanges under waterlogging stress (Zhu et al., 2020). According to our study, the application of exogenous SA significantly increased Pn, Gs, Tr and WUE of the Z. armatum leaves (Fig. 2). Similar results were obtained in basil under salinity stress (Da Silva et al., 2022a). This is attributable to the SA application, which increased the leaf carbon assimilation capacity; this not only regulates stomatal movement during stress but also improves the activity of Rubisco and carbonic anhydrase (Khan et al., 2013; Parashar et al., 2014; Ahmad et al., 2018). Therefore, the SA application can be considered an effective measure for ameliorating the adverse effects of waterlogging stress on the Z. armatum photosynthetic performance. The microstructure of the stomata was not observed in this study, and we consider that it is necessary to observe stomatal changes under waterlogging stress. This is left for future study.
As an important photosynthetic parameter, chlorophyll fluorescence indicates how PSII absorbs and utilizes light energy, and this was generally estimated by the Fv/Fm, ΦPSII, Fv'/Fm' and NPQ outcomes (Luo et al., 2021). Fv/Fm represents the conversion efficiency of primary light energy in the PSII reaction center. ΦPSII represents the efficiency of the actual PSII light energy capture in the presence of a partially closed PSII reaction center. In addition, ETR reflects the rate of the nonlinear electron transfer processes in the optical system, whereas NPQ reflects the absorption and conversion of chlorophyll, which is an effective heat-dissipating element related to the resistance of photodamage. Our results demonstrated that Fv/Fm, ΦPSII, Fv'/Fm' and ETR of Z. armatum leaves decreased significantly under waterlogging stress, whereas Fo and NPQ increased significantly (Fig. 3). Furthermore, Fv/Fm and ΦPSII sharply decreased, which indicated that a reduced formation of assimilatory forces (e.g. ATP and NADPH) decreased the net photosynthetic rate. Shi et al. (2021) reported similar results in Hordeum jubatum. This showed that the photosynthetic apparatus of Z. armatum was disrupted or damaged by waterlogging stress. The significant decrease in the Fv'/Fm' and ETR outcomes also indicates that the electron transfer efficiency of the Z. armatum photosystem was reduced under the waterlogged condition, most likely due to the disruption of the leaf photoresponse center activity which occurred under waterlogging stress. In contrast, NPQ increased significantly, implying that Z. armatum eliminated the excess energy generated due to the waterlogging stress of the photochemical device by dissipating heat. However, the SA application increased Fv/Fm, ΦPSII, Fv'/Fm' and ETR and decreased Fo and NPQ under waterlogging stress (Fig. 3). This effect has also been found in various plants, such as maize (Wang et al., 2021) and Aristotelia chilensis (Gonzalez-Villagra et al., 2022). Thus, although these results indicate that SA can improve plant photosynthesis under waterlogging stress, the underlying mechanism requires further investigations.
In conclusion, waterlogging stress will adversely affect the photosynthetic characteristics of seedlings. The Chl a, Chl b and total chl contents as well as Pn, Gs, Tr, WUE, Fv/Fm, ΦPSII, Fv'/Fm' and ETR outcomes all decreased with the extension of the waterlogging stress time, whereas Ci, Fo and NPQ increased. An exogenous SA application can alleviate the reduction of the contents of photosynthetic pigments as well as the photosynthetic rate and can enhance PSII activity under waterlogging stress by increasing the photochemical efficiency and reducing heat dissipation to mitigate the resulting photodamage (Fig. 5). It was confirmed here that the appropriate SA concentration significantly promotes the photosynthetic capacity of Z. armatum seedlings, and the results provide detailed insight into the photosynthetic physiological processes involved in the SA-mediated adaptation of Z. armatum seedlings to waterlogging stress.