Introduction
Materials and Methods
Plant Material, Treatment, and Storage Conditions
Measurement of Ethylene Production and CO2 and O2 Concentration
Measurement of Fruit Tissue Firmness and Soluble Solids Content
Anatomical Determination of the Morphological Structure of Attachment Tissue
Analysis of Cell Wall-degrading Enzyme Activities
Statistical Analyses
Results
Cultivar Variation in Attaining Fruit Maturity
Fruit Physiological Responses to Various MAP Treatments
Effect of Combined 1-MCP and MAP Treatments on Fruit Physiology
Effect of Combined 1-MCP and PP MAP Treatment on Fruit
Morphological Alteration in the Structural Organization of the Joint
Enzymatic Activities of Cell Wall-degrading Enzymes
Discussion
Introduction
The production and consumption of tomato (Solanum lycopersicum L.) fruit are considerably high because of their rich nutritional and mineral value (Dorais et al., 2008; Fallah et al., 2021; Vélez-Terreros et al., 2021). Tomato fruit contain a variety of primary and secondary metabolites, including soluble carbohydrates, organic acids, vitamins A and B, and lycopene, which are also known as an antioxidant compound (Raiola et al., 2014; Carillo et al., 2019). The mineral and nutrient composition of tomato fruit varies significantly with size and shape (Tanksley, 2004; Chai et al., 2021). Thus, tomato fruit can be consumed as fresh vegetables, for example as an ingredient in a salad, or as processed products such as juice. In addition, tomato fruit can be processed further to obtain tomato-based products, such as tomato powder, flakes, soup, sauce, and ketchup. To satisfy the preferences and demands of consumers, diverse varieties of tomato varying in terms of fruit size and shape have been bred, developed, and grown (Gonzalo et al., 2009; Rodríguez et al., 2011; Sherzod et al., 2020). Initially, tomato cultivation was focused on optimizing the production system to obtain high yield; therefore, the cultivators with a consistent or typical size of tomato fruit were preferred. Later, consumers began to favor smaller tomato fruit, which are more convenient to hold, thus motivating high production of cherry tomato-type cultivars. However, these cultivars have certain disadvantages such as a lower yield and a high amount of labor required for harvesting. Therefore, the truss (or cluster) type of tomato fruit emerged as an alternate and promising approach to bridge the gap between cultivation and consumption.
Tomato fruit are classified as climacteric fruit based on the physiological and biochemical processes that occur during their ripening (Cherian et al., 2014; Gil et al., 2020). The regulatory mechanisms during ripening of climacteric fruit typically involve the generation of ethylene and increased respiration rate (Paul et al., 2012; Wang et al., 2018). During fruit ripening and maturation, the detachment force usually increases concomitantly with mass (Lipe and Morgan, 1973; Chen et al., 2014; Hu et al., 2020). The fruit detachment force (FDF) is correlated with the development of the abscission zone (AZ), which is affected by hormonal regulatory mechanisms (Jackson and Osborne, 1970; Bleecker and Patterson, 1997). However, in truss-type tomatoes, which are grown and served in clusters, the knuckle detachment force (KDF) is more important than FDF for postharvest processing. As reported previously, the FDF and KDF are highly correlated with several biological changes in the fruit including alteration in fresh weight, ethylene accumulation, and development of the AZ (Bleecker and Patterson, 1997; Yuan et al., 2001). However, there is a paucity of information available in relation to the knuckle detachment force specifically in cluster- (or truss-) type tomato cultivars.
In most horticultural commodities, postharvest handling technologies play a pivotal role in controlling the fruit and vegetable quality during distribution before consumption (Kader, 1986; Kader, 2002). Numerous environmental stresses are active during postharvest processing and distribution. The ethylene accumulation during ripening may decrease the quality of horticultural commodities, thus reducing fruit marketability while on the shelf (Li et al., 2010). Furthermore, the increased activity of cell wall-degrading enzymes, such as polygalacturonase (PG) and cellulase affects the fruit texture during storage (Abeles and Takeda, 1990; Sitrit and Bennett, 1998; Missang et al., 2004; Wei et al., 2010). To control early fruit maturity after harvest, 1-methylcyclopropene (1-MCP) is employed as an inhibitor of ethylene function and perception to delay fruit ripening and senescence by suppressing ethylene production (Blankenship and Dole, 2003; Watkins, 2006). 1-MCP has been commercialized in a wide range of horticultural industries (Mattheis, 2008; Watkins, 2008; Park, 2012; Win et al., 2021). 1-MCP binds to the ethylene receptor on the endoplasmic reticulum (ER) membrane, resulting in abortive binding between ethylene and its receptor (Sisler and Serek, 1997; Kamiyoshihara et al., 2012). Therefore, the application of 1-MCP can aid in retention of tomato quality, specifically in terms of fruit texture (Jeong et al., 2004; Guillén et al., 2007).
Modified atmosphere packaging (MAP) has been widely adopted in horticultural commodities to prolong the maintenance of quality and marketability of harvested products (Beaudry, 2000; Kader and Watkins, 2000; Oliveira et al., 2015; Lwin et al., 2022). Furthermore, combined treatment with 1-MCP and MAP has also been extensively applied to retain fruit and vegetable quality after harvest, during storage, and while on the shelf (Khan and Singh, 2008; Ketsa, 2010; Özkaya et al., 2016; Kim et al., 2018). Therefore, this study aimed to determine the optimum concentration of 1-MCP to treat cluster-type tomato cultivars, including ‘Amoroso’, ‘Campari’, and ‘Aranca’. In addition, the combined effects of 1-MCP and MAP applications on fruit quality attributes and cell wall-degrading enzyme activities were evaluated.
Materials and Methods
Plant Material, Treatment, and Storage Conditions
Fruit of four hybrid tomato (S. lycopersium L.) cultivars (‘Momotaro’, ‘Amoroso’, ‘Campari’, and ‘Aranca’) were obtained from the Agricultural Products and Logistics Export Center in Nonsan, Chungcheongnam-do, Republic of Korea. The ‘Momotaro’ tomato cultivar was only used for the determination of 1-MCP application as shown in Fig. 1. The fruit of the other three cultivars were used for the rest of the study. The three tomato cultivars were planted in a greenhouse on May 10th and cultivated for ten weeks according to the standard cultivation protocol recommended by the Rural Development Administration (RDA), Republic of Korea. Each tomato seedling was planted at 70 cm, and the lateral shoots were removed to induce the fruiting on the main branch at each flower cluster. From each flower cluster, the ninth floral bud was removed to maintain fruit growth and the remaining flowers were allowed to mature until harvest.
To evaluate the fruit quality attributes and effectiveness of 1-MCP treatment along with modified atmosphere packaging (MAP) application, the fruit of the selected tomato cultivars were harvested from the commercial tomato greenhouse in Nonsan, Chungcheongnam-do on July 5th but ‘Amoroso’ tomatoes were harvested from a commercial greenhouse in Jeollabuk-do. The fruit were selected for harvest from each whole cluster based on a light red pericarp color. The fruit were then transferred to the laboratory and immediately sprayed with 80% ethanol, air-dried, and used for the subsequent analysis.
The degree of fruiting and maturation within flower clusters was determined at three fruit maturity stages: between flowering and the breaker stage, after the 1st fruit in a cluster showed a light red pericarp coloration, and during the red ripening stage. Fruit sampling was carried out from the beginning of pericarp coloration of the first fruit to reach breaker stage through the 8th fruit until uniform pericarp color obtained.
To elucidate the optimum dosage of 1-methylcyclopropene (1-MCP; SmartFreshTM, AgroFresh Inc., Dow Chemical Co., Seoul, Republic of Korea) applied through fumigation, fruit of the ‘Momotaro’ tomato cultivar were treated with varying concentrations (0, 0.01, 0.1, 1.0, or 10.0 µL·L-1) of 1-MCP at harvest. The preliminary results revealed that 1.0 µL·L-1 of 1-MCP considerably delayed fruit ripening; therefore, this concentration of 1-MCP was recorded as the optimum application dosage (Fig. 1). Briefly, tomato fruit were treated in a SmartFreshTM chamber (AgroFresh Co., Dow Chemical Co., Spring House, PA, USA) containing 0.33% 1-MCP powder. The 1-MCP powder was allowed to react with distilled water at 40°C and then vaporized for 12 h. The MAP treatment was applied using polyethylene (PE), polypropylene (PP), or oriented polypropylene (OPP) films (40 µm in thickness) and then transferred to 10 ± 1.0°C for cold storage. As an untreated control batch, air-processed tomato fruit were used to compare the alteration in fruit quality attributes.
Measurement of Ethylene Production and CO2 and O2 Concentration
Ethylene accumulation was measured by injecting 1 mL of headspace air sample using a syringe into a gas chromatograph (GC) (Young Lin Corp., M600D, Seoul, Republic of Korea). Ethylene analysis was conducted with an Al2O3-Na column (Chrompack, 0.53 mm × 50 m) with helium as a gas carrier under the following conditions: oven temperature of 70°C, injector temperature of 220°C, and detector temperature of 220°C using a flame deionized detector (FID) (Yoo et al., 2020). In addition, a gas analyzer (PBI-Dansensor, Checkmate 9900, Ringsted, Denmark) was used to measure the change in the composition of CO2 and O2 concentrations resulting from the respiration in tomato fruit after MAP application by injecting a 0.8 mm needle into the interior of the film.
Measurement of Fruit Tissue Firmness and Soluble Solids Content
To obtain a plot for each treatment, 15 fruit were evaluated for texture and soluble solids content (SSC) at 14 d after treatment (Lwin and Lee, 2022). To measure the fruit tissue firmness after peeling at its equator, a texture analyzer (TA-HDi, Stable Micro Systems Ltd., Surrey, England) was used along with a 5 mm cylinder probe (Fig. 2A) and a custom-made probe (Fig. 2B). The conditions for the measurement of pericarp firmness are listed in Table 1. The first peak value appearing on the load curve was selected and the corresponding load force (N) was marked as the hardness value. Subsequently, juice was extracted from the fruit and used for SSC measurement using a portable refractometer (CR-10, Atago Co., Tokyo, Japan).
Table 1.
Conditions applied for analysis of tomato flesh texture and receptacle tension
Anatomical Determination of the Morphological Structure of Attachment Tissue
To determine the effects of MAP treatment on the morphological structure of the abscission layer of the knuckle tissue, microscopic examination of the tissue was conducted (Torres-Pio et al., 2021). Knuckle tissue fragments were collected and submerged in 2.5% glutaraldehyde for 90 min for primary stage fixation. All fixation processes were undertaken at 4°C. After primary fixation, the samples were washed 4–5 times with phosphate buffer (pH 7.2) at 15 min intervals. Then, the samples were submerged in 1% osmic acid for 90 min to implement the second fixation. After the second fixation, the tissue fragments were cleansed with 0.1 M phosphate buffer (pH 7.2) 4–5 times at 20 min intervals. The tissues were then dehydrated using 40%, 60%, 80%, 90%, and 95% ethanol for 5 min in each concentration and for 5, 15, and 30 min with 100% ethanol. After dehydration, the collected sections were submerged in ethanol + propylene oxide (1:1) solution for 15 min and then submerged in propylene oxide for 15 and 30 min. Next, the samples were processed with propylene oxide + epon at 2:1 and 1:1 ratios for 90 min each and finally submerged in epon solution overnight. Subsequently, the epon solution was swapped, processed for 15 min, and then placed in a silicon mold with a section of the epon sample containing DMP 30 (approximately 1.5% of epon). The sections were hardened at 60°C for 4 d to produce an epon block. The epon block was sliced at a width of 1.5 µm using an ultra-microtome (RMC Boeckeler, ST Instruments B.V., Groot-Ammers, Netherlands) and placed on a glass slide. Thereafter, the slides were treated with distilled water, stained, and dried for over 5 h. Staining was carried out by submerging the slide in 0.5% periodic acid (H2IO6) solution for 30 min, followed by washing 2–3 times with distilled water for 10 min. The sections were subsequently processed with Schiff’s reagent for 15 min, followed by treatment with 1% sodium bisulfite solution for 5 min and cleansing with running water for 30 min. The stained samples were re-dried for over 6 h, covered with cover glass, and examined under a light microscope (Olympus Corp., Tokyo, Japan) at 100 × magnification.
Analysis of Cell Wall-degrading Enzyme Activities
Prior to the analysis of enzyme activity, the cell wall-degrading enzymes were extracted using the following procedure. Approximately 3 g samples representing each storage period, stored at –70°C, were powdered in liquid nitrogen. The samples were immediately homogenized in 20 mL of 50 mM sodium acetate buffer (pH 4.0) and then filtered with miracloth before centrifugation for 20 min at 14,000 × g to acquire the supernatant. Subsequently, 80% ammonium sulfate solution was added to the supernatant and samples were re-centrifuged before a final elution using 2.5 mL of 50 mM sodium acetate buffer (pH 4.0) followed by a desalting step using a PD-10 column (Amersham Biosciences AB, Uppsala, Sweden). The eluted product was then employed for further enzyme characterization.
PG activity was determined according to the method described by Gross (1982). Briefly, 100 µL of a solution containing 0.2% polygalacturonic acid (Merck KGaA, Darmstadt, Germany) dissolved in 50 mM sodium acetate buffer (pH 4.0) was mixed with 50 µL of crude extract and 50 µL of distilled water. The mixture was allowed to react at 40°C for 120 min. Subsequently, the reaction was interrupted by adding 1 mL of 100 mM borate buffer (pH 9.0). After adding 200 µL of 1% 2-cyanoacetamide and boiling for 10 min followed by cooling at 22°C, the mass of the generated reducing sugar was measured as the absorbance at 276 nm. As a control, borate buffer was added to crude extract prior to the addition of the substrate.
Cellulase activity was determined according to the method described by Massiot et al. (1989). Briefly, 100 µL of crude extract was mixed with 100 µL of a solution containing 0.2% CMC (Merck KGaA, Darmstadt, Germany) dissolved in 100 mM Na-acetate buffer (pH 5.0) and then incubated at 40°C for 60 min. Thereafter, the reaction was interrupted with 1 mL of 100 mM borate buffer (pH 9.0) and boiled for 10 min after adding 200 µL of 2-cyanoacetamide. Subsequently, the samples were cooled to room temperature and the absorbance was measured at 276 nm.
Statistical Analyses
A completely randomized design was implemented for each study, along with one-way or two-way analysis of variance (ANOVA). ANOVA was applied to evaluate the significance of the main factors and the effects of their interaction during cold storage. This was followed by the least significant difference test for the determination of the mean difference at P < 0.05. Statistical analyses were performed using SAS statistical software (Version 9.3; SAS Institute Inc., Cary, NC, USA). All experimental data are presented as mean ± standard error.
Results
Cultivar Variation in Attaining Fruit Maturity
Three different cluster-type tomato cultivars, ‘Campari’, ‘Amoroso’, and ‘Aranca’, were used to determine the fruit ripening time defined as the time interval between pollination and the breaker stage. The fruit of the ‘Aranca’ cultivar displayed the shortest ripening time, whereas the ‘Amoroso’ fruit took the longest time to reach the breaker stage (Table 2). However, the fruit of the ‘Campari’ cultivar experienced the longest time interval between the breaker stage and the red ripening stage, while this time interval of ‘Aranca’ fruit was the shortest, similar to the time period between pollination and the breaker stage (Table 2).
Table 2.
Days taken to ripening of 1st fruit in cluster-type tomato
Cultivar | Days taken to reach break stagez | Days taken to reach red stagey |
Campari | 51.8 ± 1.0 bx | 7.6 ± 0.9 a |
Amoroso | 54.4 ± 3.2 a | 7.4 ± 1.0 b |
Aranca | 49.0 ± 2.5 c | 4.3 ± 0.9 c |
The responses pertaining to color development in pericarp tissue differed depending on the cultivar and vine number of cluster-type tomatoes, as shown in Table 3. The time interval between when the first fruit reached the breaker stage and when the 8th fruit on the same vine reached the breaker stage was longest in the ‘Amoroso’ cultivar and shortest in the ‘Campari’ cultivar. In addition, the time period between the first and 8th fruit reaching the breaker stage increased, although there was no significant effect interpreted from the ANOVA (Table 3). Furthermore, there was no interaction effect between the cluster tomato cultivar and vine number.
Table 3.
Pericarp color development response of each cluster tomato cultivar
Vine No. | Amoroso | Campari | Aranca |
Days taken to reach break maturity stage from 1st fruit to 8th fruit | |||
2 | 8.6 ± 1.2 az | 5.4 ± 1.5 de | 6.7 ± 1.4 b |
3 | 7.1 ± 2.5 b | 4.6 ± 0.9 ef | 6.5 ± 1.0 cd |
4 | 6.9 ± 1.4 b | 4.0 ± 1.7 f | 5.7 ± 1.2 cd |
Overall mean | 7.5 ± 1.8 | 4.6 ± 1.1 | 6.3 ± 1.2 |
LSDα=0.05 | 1.48y | ||
Significancex | Cultivar**, Vine numberNS, Cultivar×Vine numberNS |
Fruit Physiological Responses to Various MAP Treatments
Three different types of MAP treatments, utilizing polyethylene (PE), polypropylene (PP), and oriented polypropylene (OPP) films, were applied to elucidate the effectiveness and responsiveness to the corresponding MAP applications. The ethylene production rate gradually increased during MAP treatment and remained similar among the cluster-type tomato cultivars until day 4. After that, the ethylene production rate of ‘Aranca’ fruit remained constant during the rest of cold storage, while that of ‘Campari’ fruit declined slowly and remained the lowest among all cultivars (Fig. 3). In untreated (control) fruit, SSC was highest in ‘Amoroso’ fruit and lowest in the ‘Aranca’ cultivar after two weeks of cold storage. Nonetheless, SSC was the highest in the ‘Amoroso’ cultivar and lowest in the ‘Aranca’ cultivar (Fig. 3), like the trend observed in the untreated fruit.
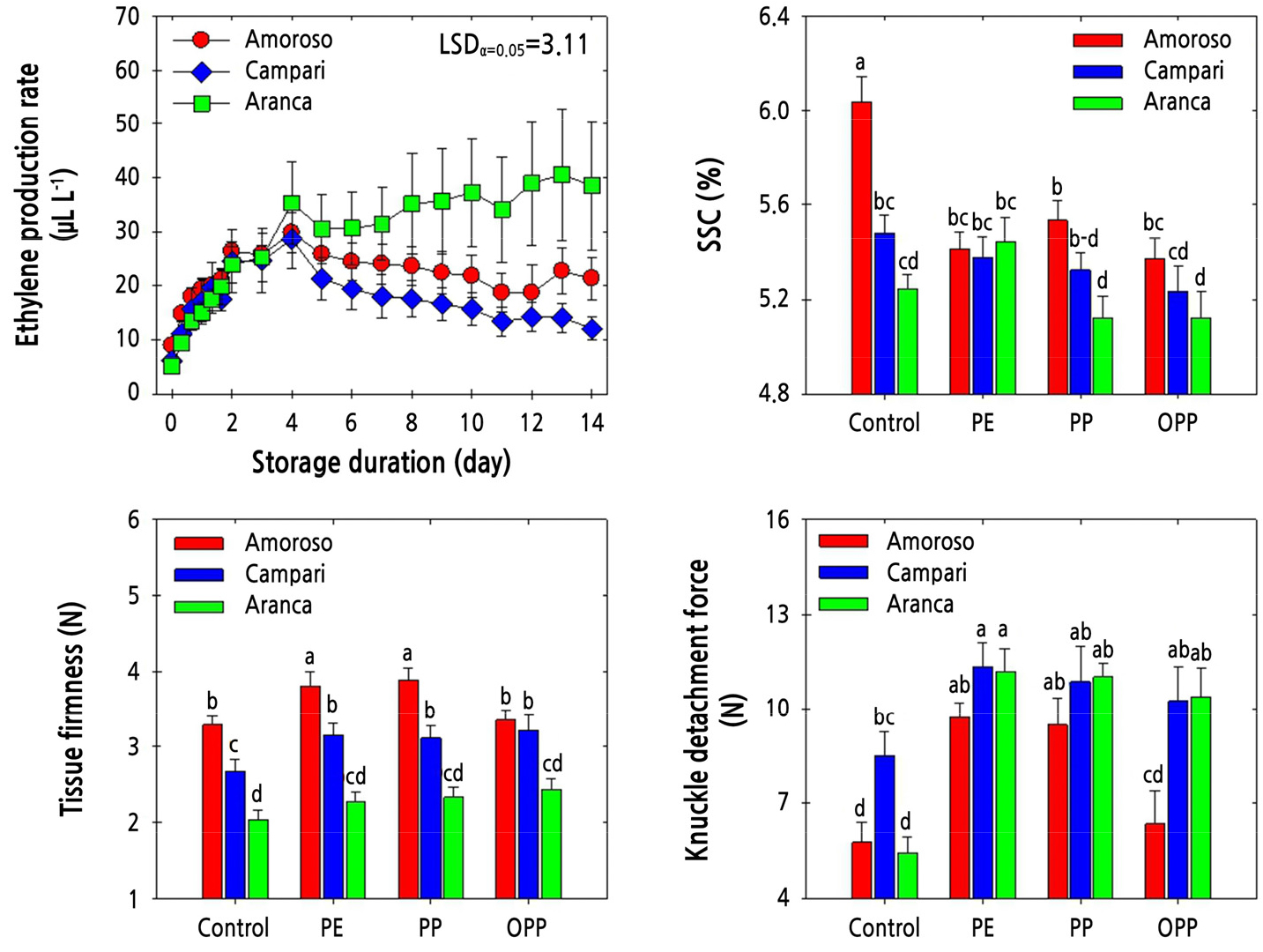
Fig. 3.
Ethylene production rate, soluble solids content (SSC), pericarp tissue firmness, and knuckle detachment force in ‘Amoroso’, ‘Campari’, and ‘Aranca’ cluster tomato fruit packed with polyethylene (PE), polypropylene (PP), or oriented polypropylene (OPP) MAP films and stored at 10°C for up to 14 d. The data point of ethylene production rate indicates the mean of five replicates (n = 5) ± standard error (SE), whereas the measurement of SSC, pericarp tissue firmness, and fruit detachment force are represented as the mean of 15 replicates (n = 15) ± standard error (SE).
Pericarp tissue firmness was also highest in ‘Amoroso’ and lowest in ‘Aranca’ after cold storage, irrespective of the MAP treatment type. The ‘Amoroso’ fruit subjected to PE and PP MAP treatments displayed the highest pericarp tissue firmness (Fig. 3). On the other hand, KDF was higher in the fruit treated with MAP than in the untreated fruit. Furthermore, KDF was higher in the ‘Campari’ and ‘Aranca’ cluster tomato cultivars than in the ‘Amoroso’ cultivar, irrespective of the MAP treatment type. In general, the PE and PP MAP treatments were associated with a higher KDF than the OPP MAP treatment and the absence of treatment (Fig. 3).
Effect of Combined 1-MCP and MAP Treatments on Fruit Physiology
To identify the relevant dosage of 1-MCP, 1-MCP fumigation treatments were performed at several concentrations as a preliminary study (Fig. 1). Based on the results, 1 µL·L-1 1-MCP treatment was identified as the optimal dosage to control the fruit ripening of the cluster-type ‘Momotaro’ tomato cultivar when stored at 20°C for up to 7 d. In addition, the optimum level of 1-MCP treatment was determined over four fruit maturation stages, including the mature green, breaker, turning red, and light red stages. The results indicated that 1 µL·L-1 of 1-MCP applied via fumigation treatment could control cluster tomato fruit ripening at all stages of fruit maturity, except the light red stage (Fig. 1).
The ethylene production rate in ‘Amoroso’ fruit gradually increased during cold storage. However, the samples treated with 1-MCP showed a lower rate of ethylene production than the untreated control fruit. To elaborate, 1-MCP treatment reduced the ethylene production rate until day 4 during cold storage. Subsequently, the ethylene production rate remained unchanged in the 1-MCP-treated samples but slowly declined in the untreated fruit (Fig. 4). The SSC level was higher in the untreated fruit compared with that in the fruit subjected to MAP treatment, irrespective of 1-MCP treatment. However, the SSC levels were not altered significantly by MAP treatment or by 1-MCP treatment (Fig. 4). Pericarp tissue firmness was higher in the 1-MCP-treated fruit than in the untreated fruit in the absence of MAP treatment. Furthermore, the pericarp tissue firmness of fruit treated with 1-MCP but not MAP was similar to that of fruit from all MAP treatments, except that of fruit treated with OPP MAP but not 1-MCP (Fig. 4). KDF followed a similar trend to that observed for pericarp tissue firmness. However, KDF tended to be highest with combined 1-MCP and MAP application, independent of the MAP treatment type (Fig. 4).
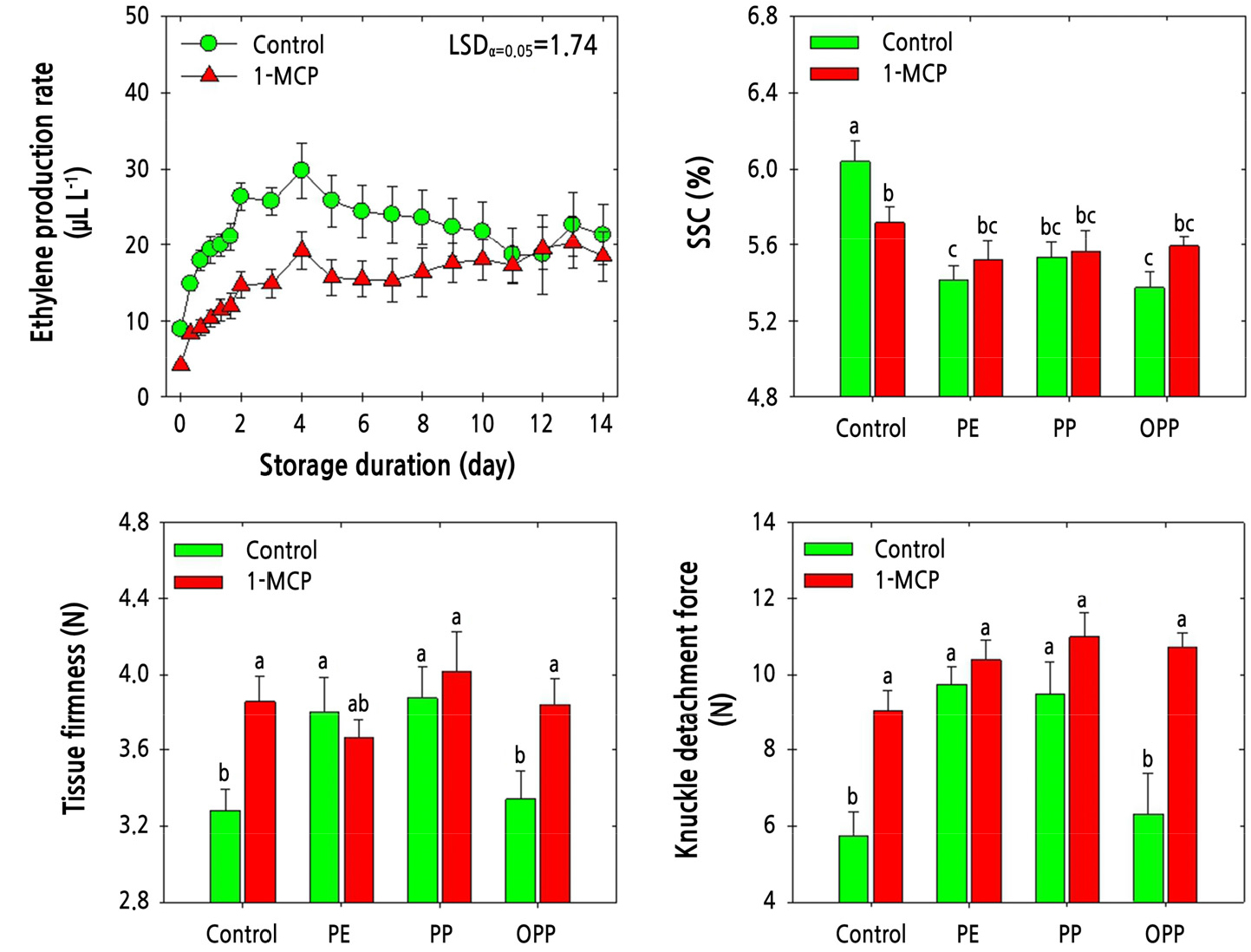
Fig. 4.
Physiological responses of ethylene production rate, soluble solids content (SSC), pericarp tissue firmness, and knuckle detachment force in ‘Amoroso’ cluster tomato fruit treated with or without 1 µL·L-1 1-MCP and packed with polyethylene (PE), polypropylene (PP), or oriented polypropylene (OPP) MAP films at harvest, then stored at 10°C for up to 14 d. The data point of ethylene production rate indicates the mean of five replicates (n = 5) ± standard error (SE), whereas the measurement of SSC, pericarp tissue firmness, and fruit detachment force are represented as the mean of 15 replicates (n = 15) ± standard error (SE).
Effect of Combined 1-MCP and PP MAP Treatment on Fruit
The ethylene production rate of ‘Amoroso’ fruit stored at 10°C for 8 d with PP MAP and with or without 1-MCP increased sharply for the first 4 d and then remained unchanged (Fig. 5). There was no significant difference in ethylene production rate between the combined 1-MCP and PP MAP treatment and the control (PP MAP alone). The KDF remained unchanged in fruit treated with both 1-MCP and PP MAP application; however, PP MAP treatment alone caused a decrease in KDF at 4 d after cold storage (Fig. 5). The concentration of CO2 gradually increased during cold storage but was lower in the combined 1-MCP and PP MAP treatment than in the PP MAP treatment (control). The reduction in O2 concentration during cold storage was less pronounced in the combined 1-MCP and PP MAP treatment than in the PP MAP treatment (Fig. 5).
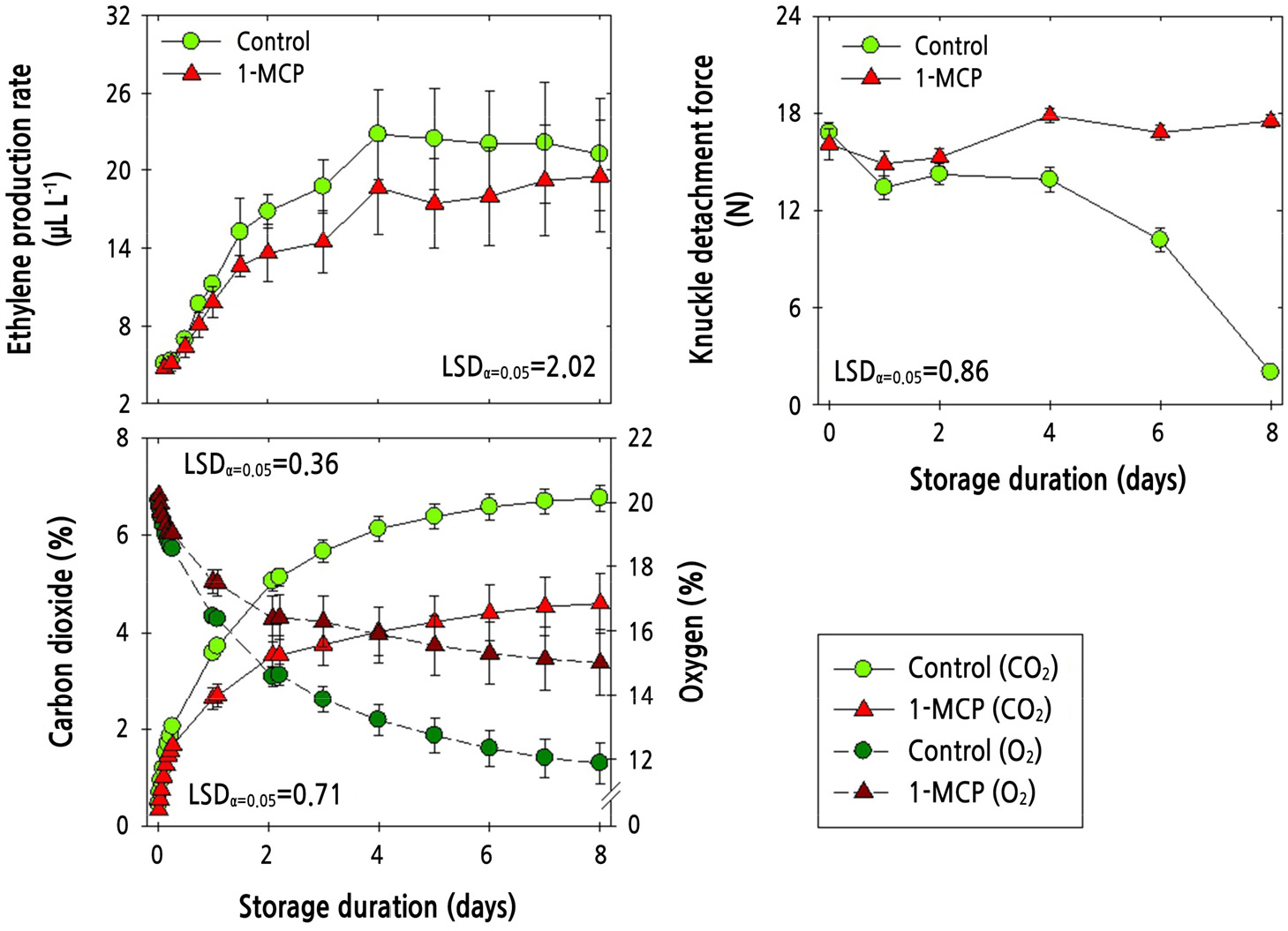
Fig. 5.
Physiological responses of ethylene production rate, knuckle detachment force, and the concentration of carbon dioxide (CO2) and oxygen (O2) in ‘Amoroso’ cluster tomato fruit treated with or without 1 µL·L-1 1-MCP treatment and packed with polypropylene (PP) MAP film at harvest, and then stored at 10°C for up to 8 d. The data point for ethylene production rate indicates the mean of five replicates (n = 5) ± standard error (SE), whereas the measurement of fruit detachment force and the measurement of the CO2 and O2 concentration are represented as the mean of 24 replicates (n = 24) ± SE and six replicates (n = 6) ± SE, respectively.
Morphological Alteration in the Structural Organization of the Joint
Two days after the PP MAP treatment, tomato fruit cells appeared to be more condensed, leading to the formation of a darker area between the cells, than cells after the combined 1-MCP and PP MAP treatment (Fig. 6C). Moreover, the bilayer extended to the phloem area and the cells became larger in the PP MAP treatment (Fig. 6E). As the phloem and the cytoplasm of adjacent cells were constricted, the pores were enlarged, coinciding with the formation of fusion cells (Fig. 6G). Furthermore, the tissues displayed damage to the phloem and phloem terminal, indicating internal tissue breakdown and dissociation of cells between abscission zones (Fig. 6I). In contrast, the samples treated with 1-MCP prior to PP MAP application did not exhibit any cell damage, and the cellular morphology was like that of the control group at 8 d after cold storage (Fig. 6J).
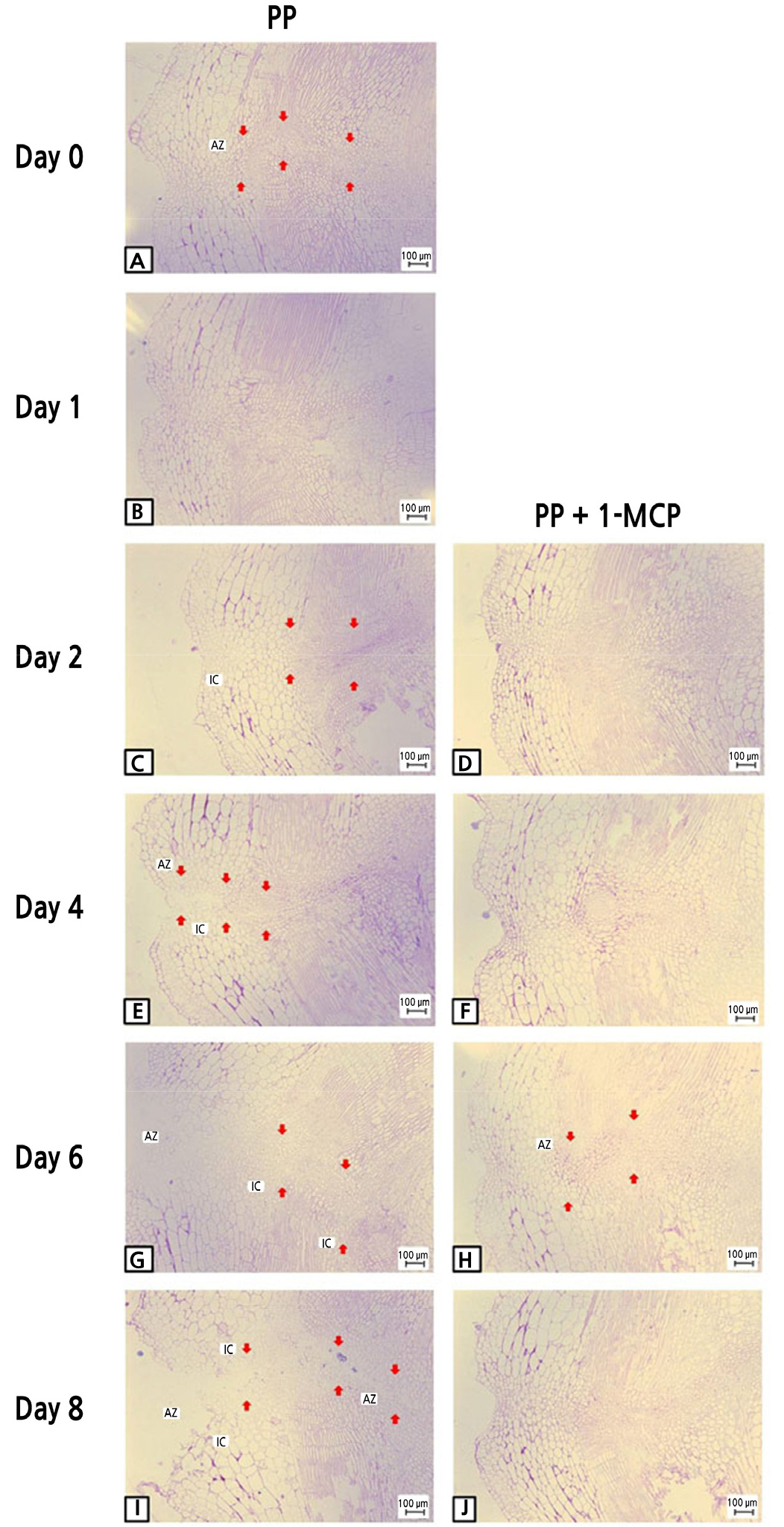
Fig. 6.
Light microscopy of longitudinal sections representing pedicel abscission zones in ‘Amoroso’ cluster tomato fruit treated with or without 1 µL·L-1 1-MCP treatment and packed with polypropylene (PP) MAP film at harvest, and then stored at 10°C for up to 8 d. AZ and IC stand for abscission zone and intercellular cavity, respectively. Arrows indicate the abscission zones. Scale bar = 100 µm.
Enzymatic Activities of Cell Wall-degrading Enzymes
The enzymatic activities of PG and cellulase were determined in the knuckle areas of cluster tomato fruit of the ‘Amoroso’ cultivar. The combined 1-MCP and PP MAP treatment resulted in a marked reduction in the enzymatic activities of PG and cellulase compared with the PP MAP treatment alone (Fig. 7).
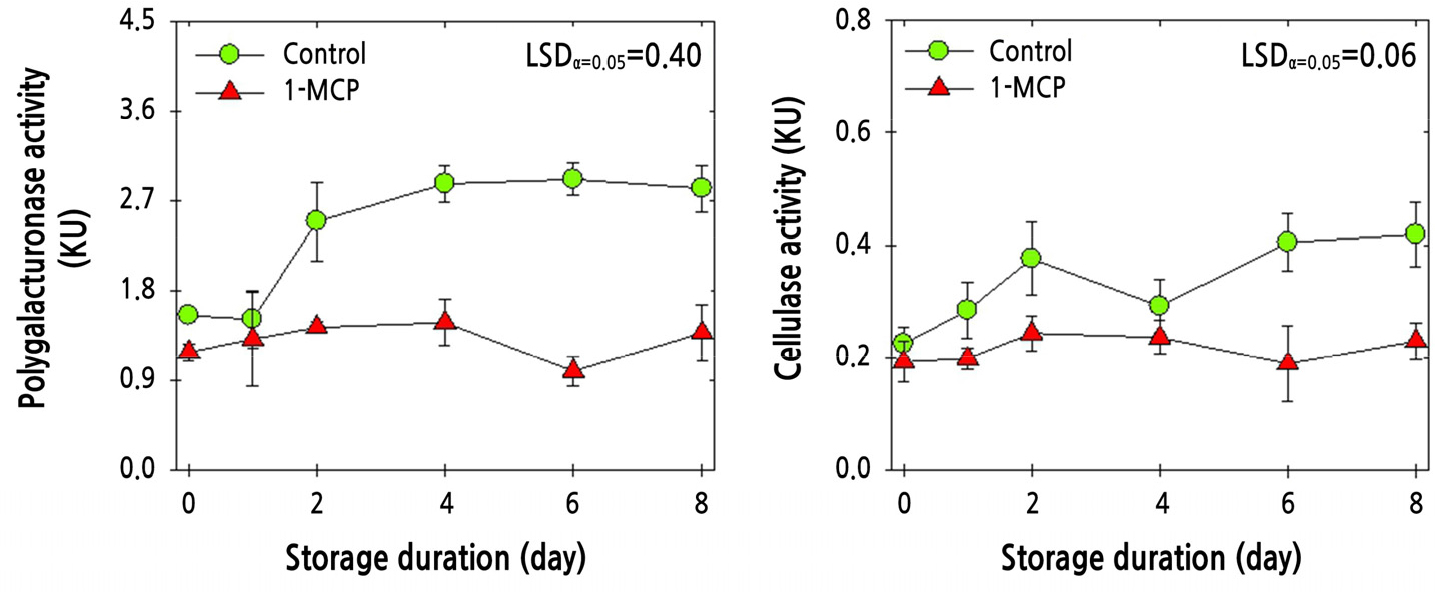
Fig. 7.
Enzymatic activities of polygalacturonase (PG) and cellulase in ‘Amoroso’ cluster tomato fruit with or without 1 μL·L-1 1-MCP treatment and packed with polypropylene (PP) MAP film at harvest and then stored at 10°C for up to 8 d. The data point indicates the mean of five replicates (n = 5) ± standard error (SE).
Discussion
With the ever-changing preferences and demands of consumers for tomatoes, the cluster-type tomato fruit is gaining popularity due to a lower harvest intensity and an extended shelf life. However, fruit quality attributes and storability of cluster-type tomato cultivars have not yet been elaborated. To retain a consistent fruit quality after harvest, 1-MCP is applied profusely in the fruit industry (Watkins, 2006; Park, 2012). In this study, the ‘Momotaro’ tomato cultivar was used to evaluate the optimum concentration of postharvest 1-MCP treatment during shelf life. The effectiveness of 1-MCP treatment on controlling fruit ripening and retaining fruit quality was most prominent at a concentration of 1 µL·L-1, which was thus considered the optimum dosage. While the responsiveness to 1-MCP treatment was not consistent at 0.1 µL·L-1, its effectiveness in controlling fruit ripening and quality maintenance was consistent at concentrations higher than 1 µL·L-1. Tomato fruit ripening was also significantly delayed when green tomatoes were treated with 1-MCP at concentrations higher than 1 µL·L-1 during shelf life (Wills and Ku, 2002). In addition, fruit quality attributes of long-term cold-stored apple fruit, such as firmness, ethylene production, superficial scald incidence, and volatile production, were found to be affected by a 1-MCP concentrations >1 µL·L-1 (Rupasinghe et al., 2000). However, in ‘Royal Gala’ apples, the fruit quality attributes were not affected by 1-MCP concentrations >0.25 µL·L-1 when compared with untreated fruit during long-term cold storage (Lee et al., 2016). Therefore, it may be inferred that while 1-MCP concentrations < 0.1 µL·L-1 have no influence on fruit ripening and quality, a concentration of 1 µL·L-1 is most suited for controlling fruit ripening and storability during cold storage and shelf life.
Among the three truss-types of tomato cultivars analyzed in the present study, fruit ripening was the most delayed in the ‘Campari’ cultivar (Table 2). The results indicated that genotypic differences among cultivars affect physiological and biochemical quality parameters and longevity of fruit after harvest (Cebolla-Cornejo et al., 2011; Kavitha et al., 2014). In addition, ethylene production was cultivar-dependent during cold storage; the highest amount of ethylene was produced in the ‘Aranca’ cultivar compared to other cultivars during cold storage. Furthermore, ethylene production can also be affected by the cold storage temperature and cultivar (Natalini et al., 2021). During cold storage, MAP was used to maintain the fruit quality and storability. PE and PP MAP were found to be most effective in retaining fruit quality in terms of SSC and pericarp tissue firmness. However, a wide range of physiological responses to MAP treatments was noted in cluster tomato cultivars (Fig. 3). Similar to these findings, MAP treatment reduced SSC in cold-stored medlar fruit (Ozturk et al., 2019) and nectarine fruit (Özkaya et al., 2016). This result may be attributed to the lower respiration rate in MAP-treated samples during cold storage and shelf life (Özkaya et al., 2016; Ozturk et al., 2019). Besides the lower respiration rates and SSC, MAP contributed to delaying the reduction in fruit firmness during cold storage and shelf life (Özkaya et al., 2016; Aglar et al., 2017; Ozturk et al., 2019). In the current study, the effects of PE, PP, and OPP MAP on the fruit of three different cluster-type tomato cultivars during cold storage were evaluated. PE and PP MAP performed better than OPP MAP in terms of SSC and pericarp tissue firmness (Fig. 3). In congruence with these results, fruit quality attributes were found to be highly affected by MAP type in cold-stored bell pepper fruit; wherein Xtend® MAP resulted in significantly higher SSC than PE MAP (Sharma et al., 2018). Moreover, fig fruit were also affected differentially by MAP type during cold storage (Bouzo et al., 2012). However, in the current study, the physiological response of treated fruit in terms of KDF was the opposite of that recorded for pericarp tissue firmness, regardless of MAP type and tomato cultivar. The contrasting results obtained for the detachment force and pericarp tissue firmness could be attributed to the advancement of fruit ripening, which contributes to an increase in the loss of fresh weight thus resulting in insufficient development of the abscission layer. Therefore, the results suggest that PE and PP MAP were sufficient to allow the retention of tissue firmness, along with a reduction in SSC levels during cold storage, even though a wide range of cultivar-based variation was observed in terms of SSC and firmness.
Based on the observations made for the performance of tomato fruit with MAP, the ‘Amoroso’ cultivar was selected to evaluate the effectiveness of postharvest 1-MCP treatment on fruit quality attributes in terms of ethylene production, SSC, and pericarp tissue firmness when applied along with several types of MAP. As shown earlier, 1-MCP treatment reduced ethylene production during cold storage, but the overall ethylene production measured under 1-MCP treatment was the same as that of untreated fruit (Fig. 4). The responses of ethylene production and respiration were not differentially affected by 1-MCP treatment as the storage duration increased, regardless of the concentration of 1-MCP treatment (Wills and Ku, 2002; Sabir and Agar, 2011b). In addition, the physiological responses of tomato fruit to 1-MCP treatment can be differentially affected according to their maturity stage at harvest; for example, the effectiveness of 1-MCP treatment was found to be lower in pink-stage fruit than in pink-stage fruit during cold storage (Sabir and Agar, 2011b). Therefore, the lower effectiveness of 1-MCP treatment in the current study may be attributed to the advancement of fruit maturity at the end of cold storage. Furthermore, MAP treatment maintained SSC in fruit during cold storage, regardless of 1-MCP treatment. On the other hand, pericarp tissue firmness and KDF were sufficiently higher in 1-MCP-treated than in untreated fruit, irrespective of MAP (Fig. 4). Nevertheless, fruit quality attributes such as pericarp tissue firmness and detachment force were highly affected by combined 1-MCP and PP MAP treatment during cold storage. The combined treatment minimized the weight loss in cold-stored tomato fruit, although a similar effectiveness was noted with 1-MCP treatment alone (Sabir and Agar, 2011a). This may be explained by the fact that 1-MCP treatment reduces ethylene production under MAP treatment; a consequent reduction in fruit respiration was also noted, which could lead to a higher retention of weight compared with that in untreated fruit. Therefore, the combined 1-MCP and MAP treatment retains the pericarp tissue firmness and detachment force in tomato fruit during cold storage. Among the various MAP technologies tested, PP MAP was the most effective in terms of SSC and firmness. Consistent with these findings, the fruit quality attributes of bell peppers were differentially affected by MAP technologies; that is, Xtend® film was more effective in terms of SSC and protection against chilling injury and decay than PE film during cold storage (Sharma et al., 2018). Furthermore, sea buckthorn berry fruit are also differently influenced by MAP film type during cold storage (Li et al., 2015). In addition, combined 1-MCP and MAP treatment reduced fruit respiration and ethylene production in long-term cold stored pear fruit, thus reducing the severity of core browning (Li et al., 2013). In the current study, combined 1-MCP and PP MAP treatment was remarkably more effective in reducing CO2 levels and delaying the decrease in O2 levels than MAP treatment alone during cold storage (Fig. 5). Thus, the retention of the fruit detachment force was more prominent in the combined 1-MCP and PP MAP treatment than in the PP MAP treatment.
The combined 1-MCP and PP MAP treatment was more effective in terms of fruit quality attributes, including SSC, pericarp tissue firmness, and detachment force, than the MAP treatment alone (Figs. 4 and 5). The maintenance of higher pericarp tissue firmness and detachment force under the combined treatment may have resulted from the lower enzymatic activities of PG and cellulase. PG activity is substantially suppressed by postharvest 1-MCP treatment during fruit ripening (Jeong et al., 2002; Choi and Huber, 2008). In fact, the effectiveness of 1-MCP treatment in controlling the PG activity in tomato fruit is more profound at lower storage temperatures than at higher storage temperatures (Mostofi et al., 2003). Furthermore, cellulase activity was also suppressed by postharvest 1-MCP treatment in avocado fruit during shelf life (Jeong et al., 2002). Thus, a decrease in fruit firmness can be delayed by postharvest 1-MCP treatment during storage (Jeong et al., 2002; Mostofi et al., 2003). Overall, the results of the current study showed that the combined 1-MCP and PP MAP treatment controlled the PG and cellulase activities, thereby delaying the deterioration of pericarp tissue firmness and retaining the detachment force in cold-stored tomato fruit.
In conclusion, to retain fruit quality during cold storage and distribution of the cluster-type tomato fruit, a 1-MCP concentration of 1 µL·L-1 is highly effective. Furthermore, among several MAP technologies, PP MAP was the most effective in retaining fruit quality attributes, especially SSC and pericarp tissue firmness in the fruit of cluster-type tomato cultivars during cold storage. In addition, combined 1-MCP and PP MAP treatment was highly effective in extending fruit quality in terms of SSC, pericarp tissue firmness, and detachment force. The extension of fruit quality attributes by the combined 1-MCP and PP MAP treatment was mainly attributed to the suppression of cell wall-degrading enzyme activities, such as PG and cellulase. Therefore, the results indicated that combined 1-MCP and PP MAP treatment could play a substantial role in controlling fruit ripening and maintaining quality during cold storage.