Introduction
Materials and Methods
Facility Specification for Plant Cultivation
Tree Cultivation under Different CO2 Concentration and Temperature Conditions
Shoot Growth, Fruit Quality, and Leaf Characteristics
Statistical Analysis
Results and Discussion
Changes in Shoot Growth and Fruit Production
Changes in Fruit Quality
Changes in Leaf Characteristics
Conclusion
Introduction
Since the start of the industrial revolution, climate change has rapidly progressed as fossil fuel usage has increased. The carbon dioxide from fossil fuels is released into the atmosphere, causing a greenhouse effect. The global mean surface temperature has risen by about 1.09°C in the 21st century compared to the 19th century (IPCC, 2021). In addition, the recent temperature rise has been more than twice as fast as that of the past hundred years. These climate changes have a significant influence not only on the human world but also on the natural world. Climate change affects the seasonal activity and populations of various organisms and generally reduces crop yields (Hur et al., 2015; Seo et al., 2021). In addition, Korea needs to have a careful management system as it shows a higher temperature increase than the global average (Lee et al., 2019). Therefore, the Korean government has selected representative concentration pathway (RCP) scenarios to reduce greenhouse gas emissions (KMA, 2020). The RCP scenario calculates the impact of human activity on the atmosphere and predicts how temperature and carbon dioxide will change (Chung, 2012). The two environmental factors in this scenario are essential because they are closely related to crop growth. Generally, elevated CO2 concentrations can promote photosynthesis, development, and yield in various crops (Prior et al., 2011; Dong et al., 2018; Jin et al., 2019; Kim et al., 2021). In contrast, crops exposed to high temperatures show physiological, morphological, and anatomical changes, and drastic productivity reduction appears (Moretti et al., 2010; Thomson et al., 2014). Therefore, it is necessary to predict crop production based on climate change.
Apples are representative fruit trees that are grown in Korea. In 2018, the cultivation area of apples was 33,234 ha, accounting for 21.2% of the total fruit tree cultivation area (Choi et al., 2018). The apple varieties were classified based on their harvest time. ‘Fuji’ apples (Malus domestica Borkh. ‘Fuji’), classified as late varieties, have the most extensive cultivation areas. The cultivation area of apples has continued to change according to price, consumer behavior, and climate change. Because apples have been cultivated in one place for over decades, it is difficult to move to cultivated areas due to climate change (Kim et al., 2009). Previous studies have described changes in the cultivation area and apple yield in the Mediterranean area (El Yaacoubi et al., 2019), Japan (Sugiura et al., 2005), China (Li et al., 2018), and India (Singh et al., 2016). However, there are insufficient cases in Korea that track the relationship between climate change and apple production for a long time and analyze its cause.
Apple cultivation is suitable for a relatively cold climate, with an average temperature of 15–22°C from April to October (Oh et al., 2004). New shoots of apple trees grew more vigorously as the temperature rose until June, after germination. After that, when July passes, the growth of shoots is almost stopped, and fruits begin to develop (Kim et al., 2008; Sagong et al., 2013). In general, photosynthesis increases when the CO2 concentration in the atmosphere increases; however, when the temperature increases, respiration becomes active, and the consumption of nutrients increases (Lee et al., 2009; Xu et al., 2015). In a previous study, when the CO2 concentration increased, the photosynthesis of apples was enhanced in the early stage of growth but decreased in the late stage.
On the other hand, an increase in temperature caused an opposite photosynthetic reaction to the rise in CO2 concentration (Kweon et al., 2013). For 6–8 weeks after flowering, more cell division occurs at higher temperatures, and fruit size increases more in ‘Fuji’ and ‘Delicious’ apple (Warrington et al., 1999). Fruit maturation is fastest at 27°C, and fruit weight and coloration depend on how much photosynthesis occurs. However, it was reported that fruit weight was different for each treatment with elevated CO2 concentrations, and fruit quality indicators were also different in many cases (Chae et al., 2006; Lee et al., 2006, Han et al., 2012). Therefore, it is necessary to observe the changes in apple trees in the long term according to an increase in CO2 concentration, an increase in temperature, and the combined effect of the two environmental factors.
The objective of this study was to analyze the growth, fruit quality, and leaf characteristics of apple trees grown under different elevated CO2 and temperature conditions over long periods.
Materials and Methods
Facility Specification for Plant Cultivation
A closed environmental control facility (37°18'N, 126°58'E) for plant cultivation was installed in the soil, with two rows of four experimental units each. Each experimental unit consisted of a soil compartment (3 × 3 × 3 m), transparent canopy enclosure (4 × 3 × 6 m), and a small utility room for the temperature control system located on the north side. A weather station measured air temperature, relative humidity, wind speed and direction, solar radiation, and rainfall. All environmental data were recorded using a data logger (21X, Campbell Scientific, Logan, UT, USA). An open-architecture distributed control system (POREX 6800, POSCON Institute, Pohang, Korea) was used to automatically collect sensor data and control the environment in the facility. The POREX 6800 distributed control system consists of two workstations (SPARCstation 20, Sun Microsystems, Menlo Park, CA, USA) based on the UNIX operating system and a process control station performing various real-time processing for field input/output points.
Each unit was designed to individually control the atmospheric CO2 concentration and air temperature. Compressed CO2 gas was mixed with fresh air flow according to the preset CO2 concentration of the bulk air in the canopy enclosure, and the mixture air was injected into each unit using an air blower. The purity of the injected CO2 gas was periodically inspected to determine whether it harmed the plant inside the unit. Depending on whether cooling or heating was required, chilled or heated water was supplied to the fan-coil unit. The conditioned air passed through the plant canopy with sufficient flux, causing slight leaf motion, and the air returned to the duct positioned near the soil level.
The atmospheric CO2 concentration and inside temperature of each unit were controlled separately at ± 1 Pa and ± 0.5°C of the preset CO2 concentration and temperature value, respectively. Referencing values for the ambient environment were obtained from meteorological stations in real-time. The solar radiation, air temperature, and air relative humidity inside the unit were measured and recorded using a 21X data logger. The atmospheric CO2 concentrations in each unit were measured using infrared CO2 gas analyzers (ZRH, Fuji Electric, Tokyo, Japan). Thus, the injected CO2 concentration was controlled at the inlet where the CO2 gas was sprayed. The unit of internal air temperature was set to 5°C higher than the ambient environment for the high-temperature condition. Four time-domain reflectometry profile probes were installed at depths of 0.15 to 1.20 m horizontally to plant roots at 0.15 m intervals. Three tensiometers were also installed at depths of 0.15, 0.45, and 0.75 m. Soil temperatures were measured using calibrated resistance–temperature detectors.
Tree Cultivation under Different CO2 Concentration and Temperature Conditions
‘Fuji’ apple trees (Malus domestica Borkh. ‘Fuji’), which account for a high percentage of South Korean apple production, was selected and used for the experiment. Each soil compartment was filled with sandy loam soil and stabilized for two years. Eight nursery apple trees grafted onto M.9 rootstocks were transplanted into each soil compartment during late fall. Four different CO2 concentrations and temperature treatments for the cultivation of apple trees were applied as follows: ambient CO2 concentration and temperature (CON), elevated CO2 concentration and ambient temperature (ECAT), ambient CO2 concentration and elevated temperature (ACET), and elevated CO2 concentration and elevated temperature (ECET). The CO2 concentrations of the ECAT and ECET units were maintained at 650 µmol CO2 mol-1, while those of the CON and ACET units were maintained at 360 µmol CO2 mol-1. The internal air temperature of the CON and ECAT units mimicked the ambient temperature variation (‒15.0–35.9°C), whereas the ACET and ECET units were maintained at an elevated temperature of 5°C over the ambient temperature variation (‒13.0–40.9°C). CO2 concentration and temperature conditioning were initiated after transplantation and lasted eight consecutive years. The relative humidity inside the units was maintained between 60 and 80%. The soil moisture content was automatically controlled by a drip irrigation system maintained at ‒50 kPa of soil moisture tension, the current practical method for cultivated apple trees (Ro and Park, 2000). During experiments, the leaf water potential of the apple trees ranged between ‒0.1 and ‒0.4 MPa. The currently recommended N-P-K fertilization rates for apple trees were chosen to maintain the optimum foliar nutrient concentrations.
Shoot Growth, Fruit Quality, and Leaf Characteristics
Tree shoot growth was measured using four trees per treatment. The total shoot length was determined as the cumulative length of all shoots. Fruit fresh weight and the number of fruits per tree were measured. The fruit fresh weights measured annually in each treatment were continuously accumulated, and the cumulative fruit fresh weight was calculated. Measurements were repeated annually.
The soluble solids content (SSC), titratable acidity (TA), and hardness of fruits harvested in the ninth year of the experiment were measured using the procedures described by Drake et al. (1981). Hunter ‘a’ value was measured using a chroma meter (CR-200, Minolta, Osaka, Japan). Fruits were placed in a 1.0 L sealed container and left at room temperature for two hours. Air samples (1 mL air) were collected, and the amounts of ethylene and CO2 generated were investigated using Gas Chromotography (HP6890, Hewlett-Packard, Huston, TX, USA).
The leaf photosynthetic rates of 8-year-old apple trees were measured using a portable photosynthesis measuring device (LI-6400, LI-COR Inc., Lincoln, NE, USA) with an LED light source chamber. Three fully expanded leaves (number 12 counted from each shoot apex) from each tree were used for photosynthesis measurements. Light intensities were set to 0, 15, 50, 100, 200, 500, 1,000, and 1,300 µmol·m-2·s-1, and the CO2 concentrations in the chamber were the same for each unit. The measured photosynthesis data were regression-analyzed using a rectangular hyperbola model widely used to express the saturation curve (Jung et al., 2015). The light compensation point and maximum photosynthetic rate were calculated using the regression coefficient of the rectangular hyperbolic model.
Ultrathin sections obtained from fruit skin harvested from trees grown under different CO2 concentrations and temperatures were stained and examined using a transmission electron microscope (LEO-906E TEM, Zeiss, Oberkochen, Germany). Stomatal density was counted on scanning electron microscope images (200×) taken with an S-2460N SEM (Hitachi, Tokyo, Japan) on the same portion of the abaxial surfaces of three randomly sampled leaves.
Statistical Analysis
The measured data were evaluated using SPSS statistical package (IBM, New York, NY, USA). Data were analyzed using Duncan’s new multiple range test after ANOVA for a completely randomized design to compare the significance between elevated temperature and CO2 concentration, as well as the effects of their interaction at a significance level of 0.05. Regression analysis was performed on a rectangular hyperbola model using the SPSS statistical package.
Results and Discussion
Changes in Shoot Growth and Fruit Production
Shoot growth showed a linear or exponential increase in all treatments, including the CON treatment (Fig. 1). CON and ACET showed similar growth patterns, and the total shoot length increased linearly to approximately 1,500 cm in the 9th year. On the other hand, the growth of ECAT and ECET stopped from the 2nd to 4th years, but in the 9th year, they grew more than the other two treatments and showed a total shoot length of over 2,000 cm. Therefore, elevated CO2 concentration treatment inhibits shoot growth in the early stages of apple tree growth but promotes growth in the late stages. The elevated CO2 concentration treatment was more effective than the elevated temperature treatment for shoot growth. This result was consistent with previous research on elevated CO2 concentrations and temperature treatment for three years (Ro et al., 2001). However, this was inconsistent with the results that elevated temperatures helped shoot growth for four years (Kweon et al., 2013). Although results show that the increase in temperature mitigates the photosynthetic decline caused by elevated CO2 concentrations (Kim and Lee, 2001), it does not match the shoot growth in apple cultivation over a long period.
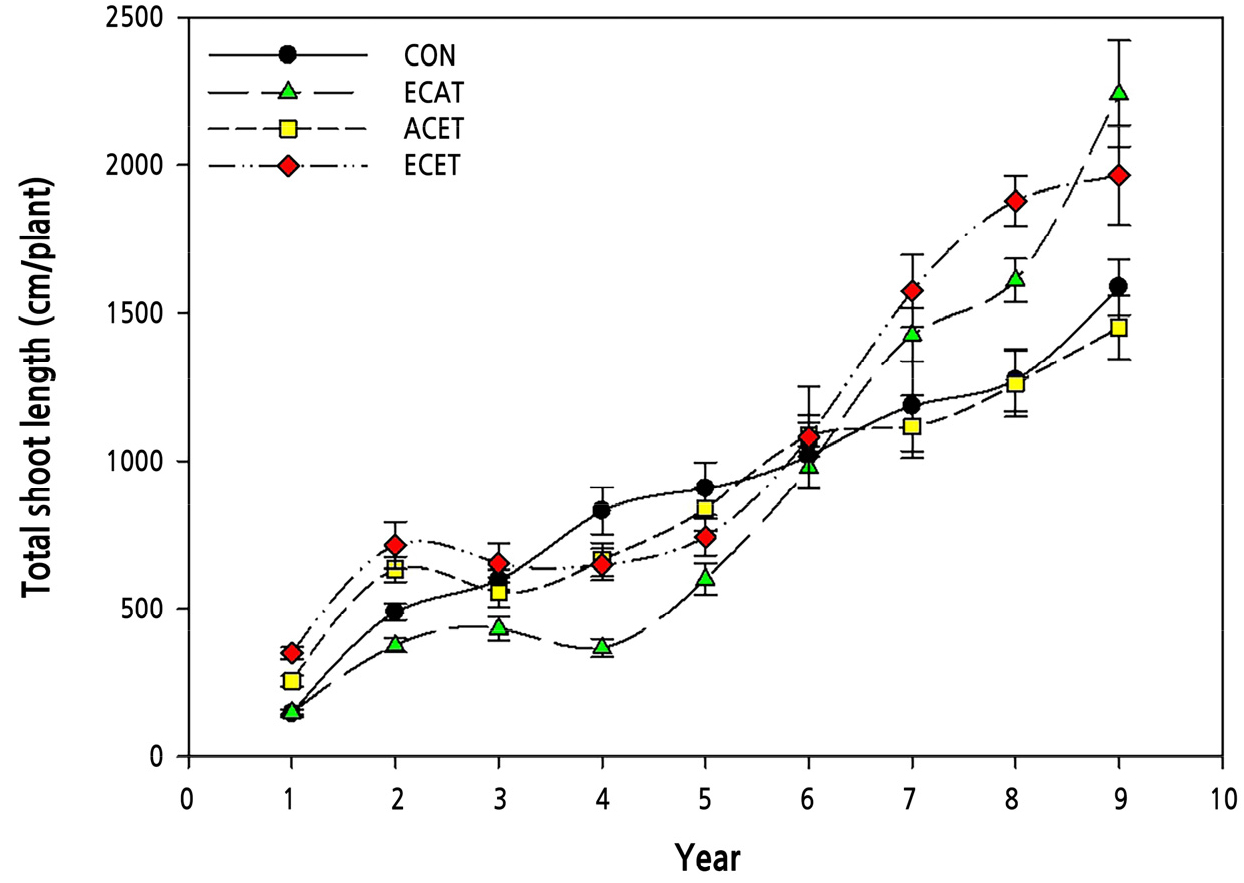
Fig. 1.
Changes in total shoot length of ‘Fuji’ apple trees grown under different CO2 concentrations and temperature conditions measured over eight consecutive years. The black circle, green triangle, yellow square, and red diamond means ambient CO2 concentration and temperature (CON), elevated CO2 concentration and ambient temperature (ECAT), ambient CO2 concentration and elevated temperature (ACET), and elevated CO2 and elevated temperature (ECET) conditions, respectively. Vertical bars represent the mean ± SD (n = 3).
The average fruit fresh weight tended to decrease in all treatment groups, except for ECAT (Fig. 2). In the CON, ACET, and ECET treatment groups, the average fruit fresh weight increased up to the 5th year but gradually decreased until the 9th year. In contrast, the average fruit fresh weight continued to increase with ECAT. However, CON had the highest cumulative fruit fresh weight during all experimental periods (Fig. 3). The average fruit fresh weight was the highest in ECAT, but the cumulative fruit fresh weight was the lowest in ECAT until the 6th year, and after the 8th year, it recovered to a level similar to that of CON and ECET. Therefore, if only the CO2 concentration increases, the size of the fruit increases in the early stage of growth, but the yield decreases, and it takes more than eight years to recover. The cumulative fruit fresh weight decreased in ACET because of increased fruit respiration at night (Oh et al., 2004; Sagong et al., 2013). Apples require a certain level of low temperature for flowering, but elevated temperature reduces the number of blooms, affecting the yield of apples (Guédon and Legave, 2008; Kweon et al., 2013).
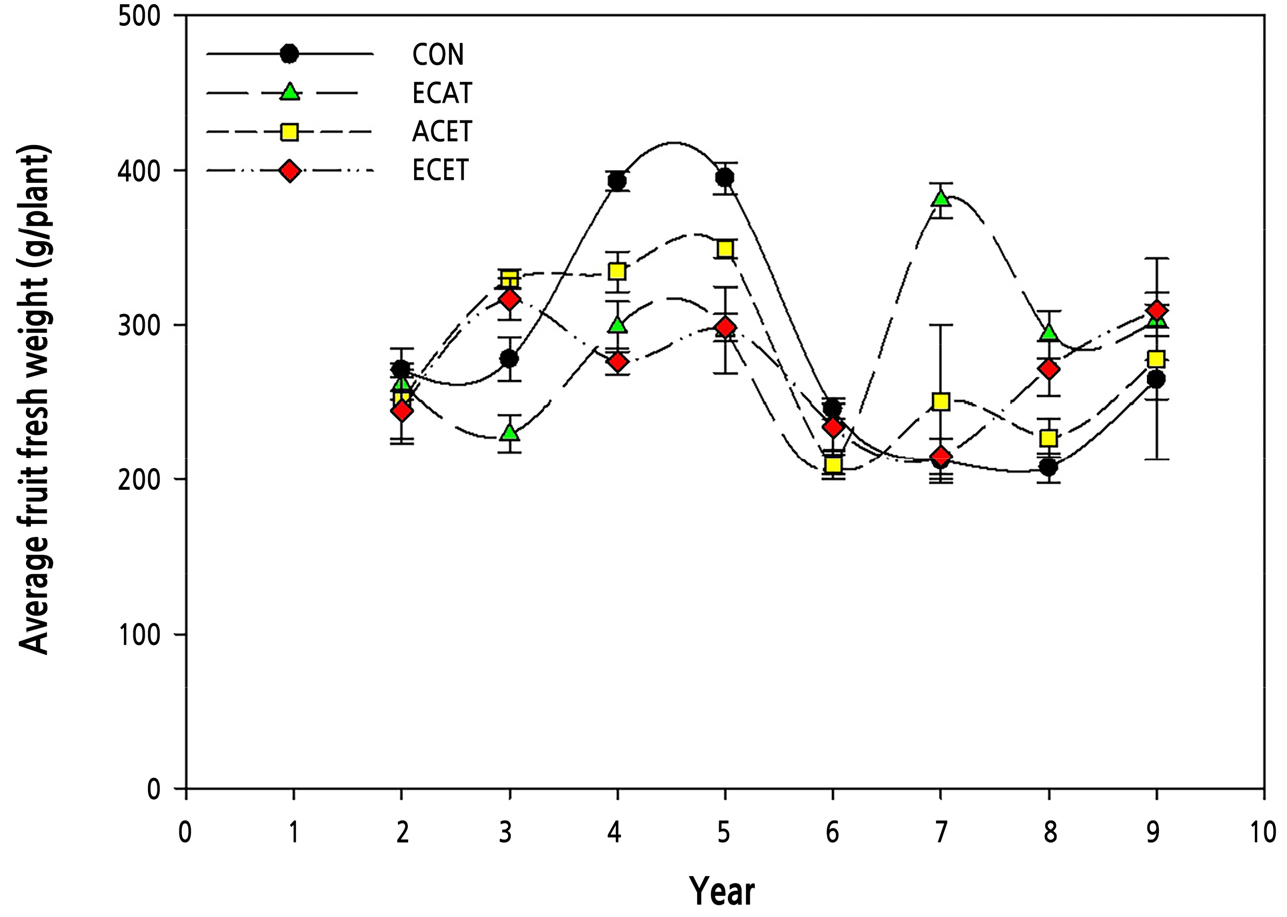
Fig. 2.
Changes in fruit fresh weight of ‘Fuji’ apple trees grown under different CO2 concentrations and temperature conditions measured over eight consecutive years. The black circle, green triangle, yellow square, and red diamond means ambient CO2 concentration and temperature (CON), elevated CO2 concentration and ambient temperature (ECAT), ambient CO2 concentration and elevated temperature (ACET), and elevated CO2 and elevated temperature (ECET) conditions, respectively. Vertical bars represent the mean ± SD (n = 3).
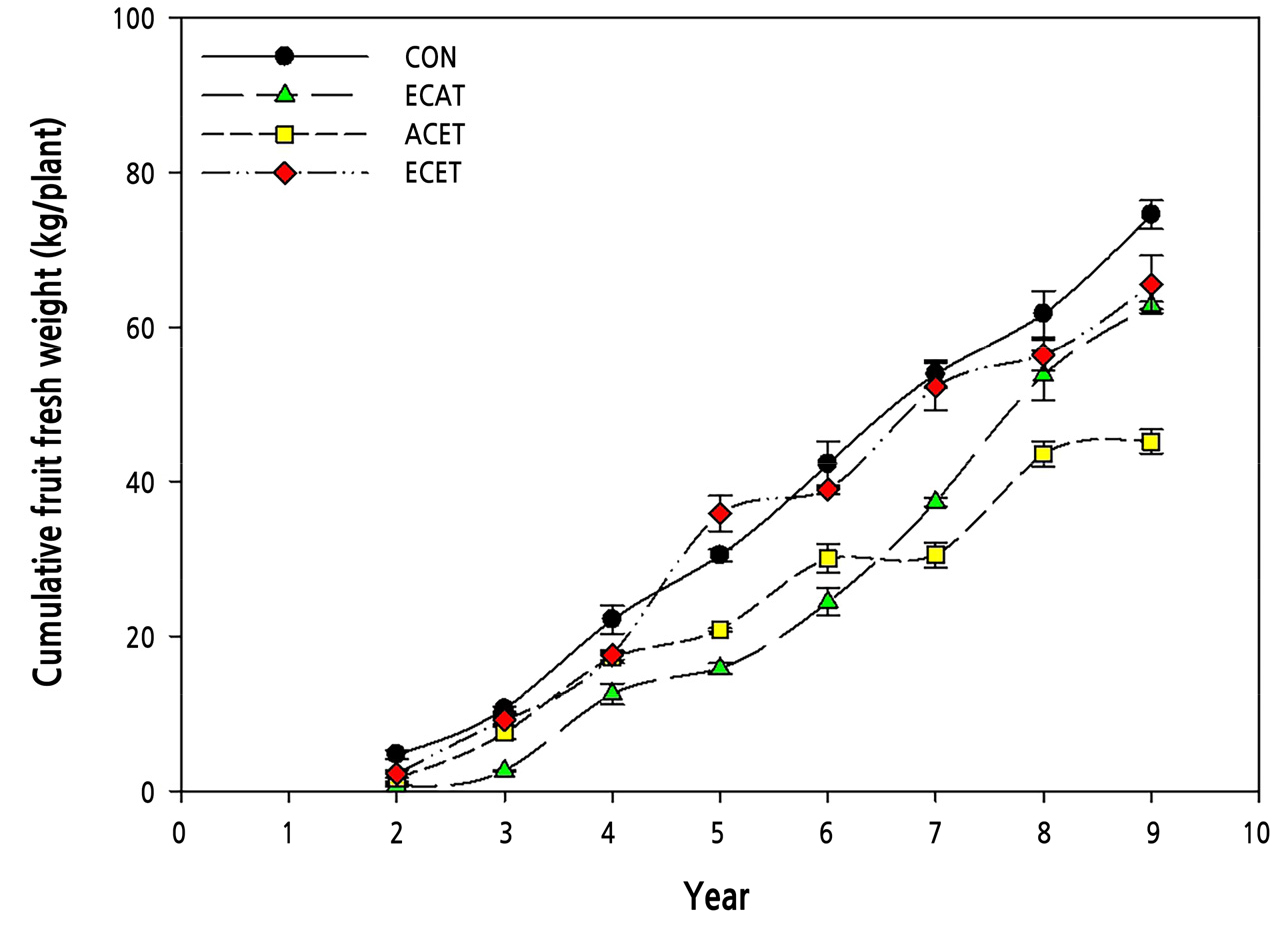
Fig. 3.
Changes in cumulative fruit fresh weight of ‘Fuji’ apple trees grown under different CO2 concentrations and temperature conditions measured over eight consecutive years. The black circle, green triangle, yellow square, and red diamond means ambient CO2 concentration and temperature (CON), elevated CO2 concentration and ambient temperature (ECAT), ambient CO2 concentration and elevated temperature (ACET), and elevated CO2 and elevated temperature (ECET) conditions, respectively. Vertical bars represent the mean ± SD (n = 3).
Changes in Fruit Quality
The effect of elevated CO2 concentration and temperature was apparent, even when observing the appearance of apples with the naked eye (Fig. 4). In the 5th and 8th years of the experiment, the apples of CON showed the typical appearance of a red ‘Fuji’ apple (Fig. 4a), but the coloration was not appropriately performed in the other treatments (Fig. 4b, c, and d). The ECAT apples were confirmed to have decreased in size in the 5th year and recovered in the 8th year. This shows some changes in the average fruit fresh weight of the apples (Fig. 2). The soluble solids content of apples decreased in all treatment groups except CON, and titratable acidity increased inversely (Table 1). The Hunter ‘a’ value was 7.8 in CON, but other treatments ranged from 0.8 to ‒5.3, so the fruit skin color did not appear correctly. These results indicate that the quality of apples produced under climate change has reduced. The color of apples is based on the anthocyanins produced in the fruit skin, which are synthesized from primary photosynthetic products (Honda and Moriya, 2018). This result is consistent with a previous study in which the color of apples did not appear appropriately because the production of anthocyanins was suppressed by high temperatures (Lin-Wang et al., 2011). Studies have shown that elevated CO2 affects fruit color during storing harvested apples (Rudell et al., 2002), but there are no studies on its effect during growth.
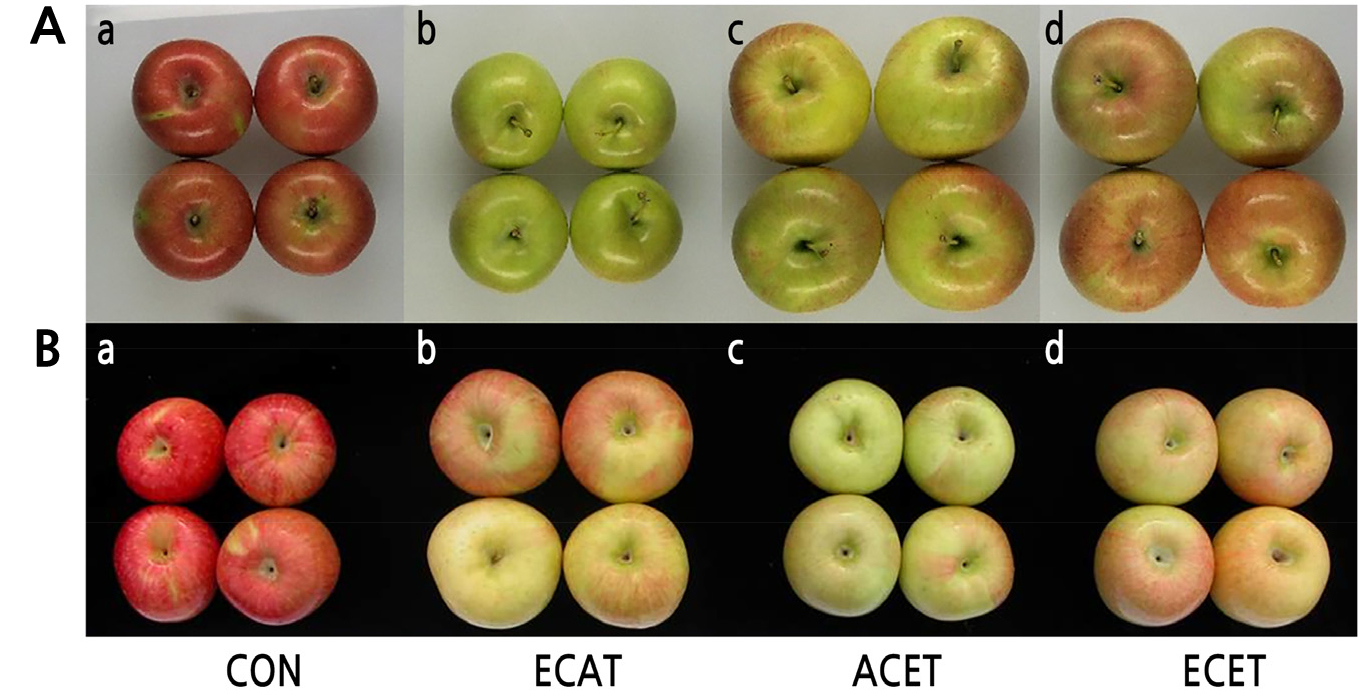
Fig. 4.
Changes in characteristics of ‘Fuji’ apple fruit harvested from 3-year-old (A) and 5-year-old (B) trees grown under ambient CO2 concentration and temperature (a; CON), elevated CO2 concentration and ambient temperature (b; ECAT), ambient CO2 concentration and elevated temperature (c; ACET), and elevated CO2 and elevated temperature (d; ECET) conditions.
Table 1.
Fruit fresh weight, number of fruits, soluble solids content (SSC), titratable acidity, hardness, and Hunter ‘a’ value of ‘Fuji’ apple fruits harvested from 8-year-old trees grown under different CO2 concentrations and temperature conditions
Treatmentz | SSC (°Brix) | Titratable acidity (%) | Hardness (kgf, 5 mm) | Hunter ‘a’ value |
CON | 15.1y ax | 0.17 b | 3.2 b | 7.8 a |
ECAT | 13.8 ab | 0.22 a | 3.1 b | 0.8 ab |
ACET | 13.1 ab | 0.22 a | 3.3 b | ‒1.9 ab |
ECET | 12.2 b | 0.23 a | 3.8 a | ‒5.3 b |
The results of the fruit skin images obtained using TEM also showed that the skin thickness of the three treatments was thinner than that of CON (Fig. 5). In CON, the epidermis cells were multilayered and thick (Fig. 5A), but this was not the case in other treatments. Anthocyanins are synthesized in this epidermal cell layer and play an essential role in determining fruit color (Lancaster et al., 1994). The amount of ethylene emitted from the fruit was higher in the treatment group with elevated CO2 concentrations (Table 2). However, the amount of CO2 emitted by fruit respiration was low, at a level similar to that of CON in ECET. It was judged that the respiration rate did not increase in the fruit adapted to the elevated temperature. In addition, the amount of respiratory gas emitted may be related to the thickness of the fruit skin, and additional research should be conducted.
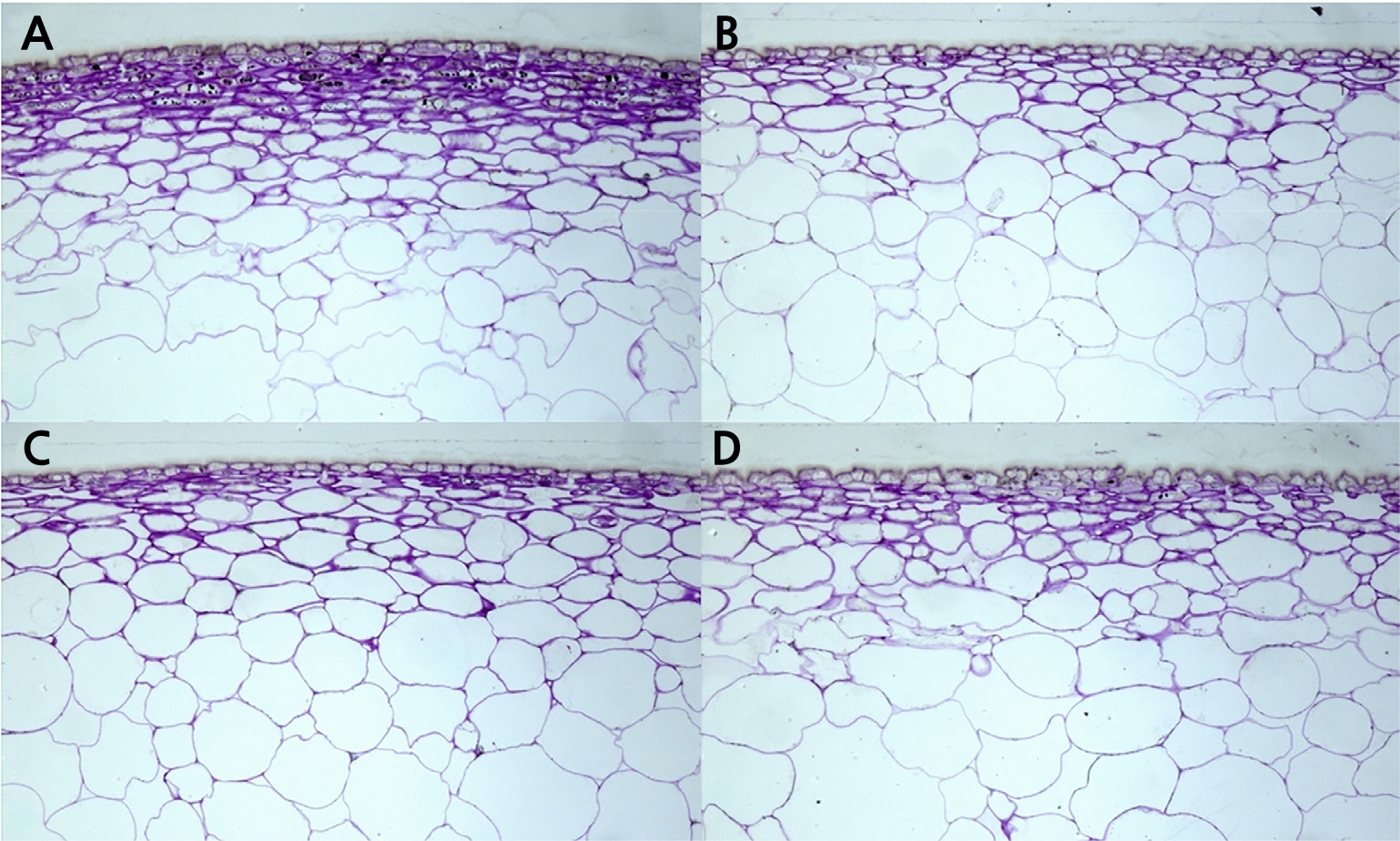
Fig. 5.
Transmission electron microscope (TEM) images of fruit skin cells of 8-year-old ‘Fuji’ apple trees grown under ambient CO2 concentration and temperature (A; CON), elevated CO2 concentration and ambient temperature (B; ECAT), ambient CO2 concentration and elevated temperature (C; ACET), and elevated CO2 and elevated temperature (D; ECET) conditions.
Table 2.
Comparison of C2H4 and CO2 production of ‘Fuji’ apple fruit harvested from 8-year-old trees grown under different CO2 concentrations and temperature conditions
Treatmentz | C2H4 (mL·kg-1·h-1) | CO2 (mL·kg-1·h-1) |
CON | 0.48y abx | 192 b |
ECAT | 0.88 a | 352 a |
ACET | 0.25 b | 224 ab |
ECET | 0.98 a | 172 b |
Changes in Leaf Characteristics
SEM images were obtained to confirm the number of stomata in the leaves, and the position of the stomata was marked with a red cross (Fig. 6). The SEM images did not show any morphological characteristics that could be distinguished, except for the number of stomata. The stomatal density was high in ECAT and ACET, and CON and ECET showed similar levels (Table 3). In leaves subjected to drying or high temperature, stomatal density decreases, which reduces photosynthesis (Bai et al., 2013). As a result of measuring photosynthesis and analyzing it in the rectangular hyperbola model, the light compensation point was 1.88 to 6.01 µmol·m-2·s-1, all of which were lower than the general level of apple leaves (Tartachnyk and Blanke, 2004). Since the maximum leaf photosynthetic rate (Amax) was the highest in ECAT and lowest in ACET, it was confirmed that photosynthesis was lowered under high-temperature conditions. The maximum photosynthetic rate in all treatment groups, except for ECAT, was similar to that reported in a previous study (Jeong et al., 2017). When the CO2 concentration and temperature were increased simultaneously, the maximum leaf photosynthetic rate was similar to that of CON. This result is shown in Fig. 2, which indicates that an increase in photosynthesis leads to a rise in the average fruit fresh weight. In conclusion, elevated CO2 concentration increases the leaf photosynthetic rate, elevated temperature decreases the photosynthetic rate, and the downregulation effect of temperature increase appears to be greater than that of CO2 concentration. The increase in photosynthesis positively affects the growth of shoots and average fruit weight, but climate change could decrease the quality of ‘Fuji’ apple fruits.
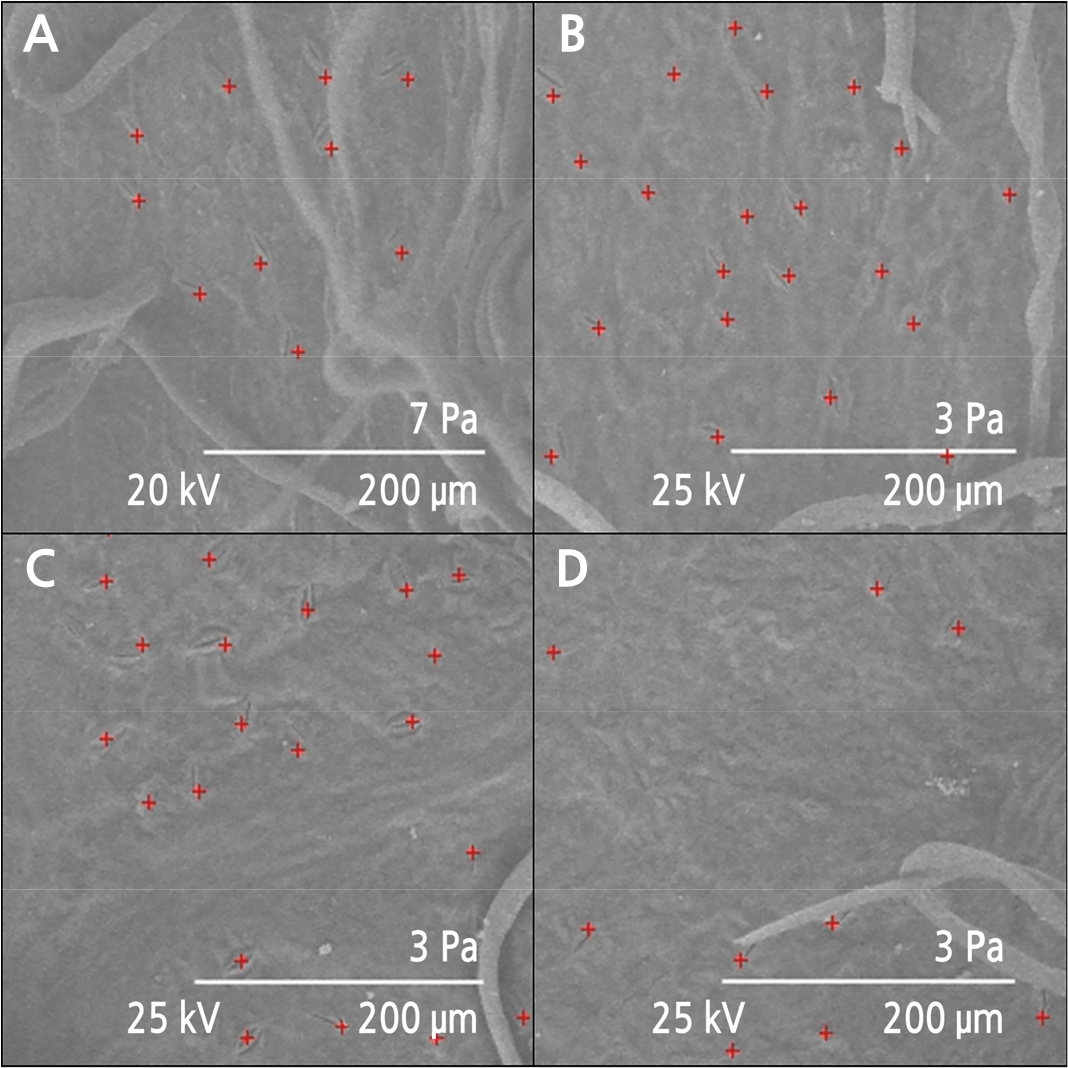
Fig. 6.
Scanning electron microscope (SEM) images of leaves of 8-year-old ‘Fuji’ apple trees grown under ambient CO2 concentration and temperature (a; CON), elevated CO2 concentration and ambient temperature (b; ECAT), ambient CO2 concentration and high temperature (c; ACET), and elevated CO2 and elevated temperature (d; ECET) conditions. The red cross marks the location of the stomata.
Table 3.
Comparison of stomatal density, light compensation point, and maximum leaf photosynthetic rate (Amax) of ‘Fuji’ apple leaf harvested from 8-year-old trees grown under different CO2 concentration and temperature conditions
Treatmentz |
Stomatal density (ea cm-2) |
Light compensation point (µmol·m-2·s-1) | Amax (µmol CO2·m-2·s-1) |
CON | 86y bx | 1.88 b | 13.49 b |
ECAT | 138 ab | 2.50 ab | 28.45 a |
ACET | 189 a | 4.35 ab | 10.98 b |
ECET | 69 b | 6.01 a | 14.24 b |
Conclusion
This study investigated shoot growth, fruit quality, and leaf characteristics by cultivating apples under elevated CO2 concentrations and temperatures for nine consecutive years. Elevated CO2 concentrations promoted shoot growth and average fruit fresh weight but reduced fruit yield at the early stage of development, with more than eight years of recovery. Elevated temperature decreased shoot growth, average fruit fresh weight, and fruit yield, as it caused a decrease in the leaf photosynthetic rate. However, when CO2 concentration and temperature elevated simultaneously, shoot growth, fruit yield, and leaf photosynthetic rate were maintained at elevated CO2 and temperature levels. However, under all climate change conditions, it was predicted that the the fruit quality would decrease, and thus the marketability of apples would decrease. In the future, as climate change continues, this study can identify the type of cultivation and management practices needed to maintain the fruit quality of apples.