Introduction
Materials and Methods
Inoculation Methods
Measurements
Results
Phytophthora Root Rot (Phytophthora cactorum)
White Root Rot (Rosellinia necatrix)
Southern Blight (Athelia rolfsii)
Woolly Apple Aphid [Eriosoma lanigerum (Huasm.)]
Discussion
Soil-borne Diseases
Woolly Apple Aphid (WAA)
Introduction
Phytophthora root rot, white root rot, and southern blight are destructive soil-borne diseases caused by Phytophthora cactorum, Rosellinia necatrix, and Athelia rolfsii, respectively, that occur in various crops (Hantula et al., 2000; Flores-Moctezuma et al., 2006; Kondo et al., 2013). Once they develop, it is difficult to prevent further outbreaks unless soil sterilization is performed completely, even if the fungus-infected tree is removed. These diseases can also be transmitted sequentially to healthy trees in the neighborhood, which could lead to more damage in orchards (Sewell and Wilson, 1959; Anselmi and Giorcelli, 1990). As the high-density orchard system has become more common in Korea since the late 1990s, the planting space between trees has become denser. Therefore, transmission to adjacent trees is expected to appear faster and wider than before.
Disease development is encouraged by several factors, including adequate soil moisture, moderate temperature, aerobic soil, tree-age, and rootstock susceptibility (Pérez-Jiménez, 2006). For practical management to control these pathogens, the use of resistant rootstocks with appropriate fungicide application, soil sterilization, and soil replacement around the host may be the best long-term option (Ellis et al., 1982; Utkhede and Smith, 1993; Carisse and Khanizadeh, 2006; Eguchi et al., 2008). Despite the few studies on the resistance of apple (Malus domestica Borkh.) rootstocks against P. cactorum, R. necatrix, and A. rolfsii, the resistance to soil-borne disease is reported to vary depending on the rootstock type (Utkhede and Quamme, 1988; Wilcox, 1993; Lee et al., 2000).
Woolly apple aphid (WAA) [Eriosoma lanigerum (Huasm.)] is a common pest in apple orchards. It can be observed year-round in the cracked bark of the mature trees or the rhizosphere, especially in trees affected by severe sucker. The WAA feed by sucking the sap, which results in galls on the roots and shoots of the apple trees, causing damage to the xylem vessel and eventually affecting the fruit quality by reducing the vigor of the trees (Klimstra and Rock, 1985; Brown et al., 1995). Although infestation to the canopy of apple trees by WAA can be controlled by pesticides and the WAA parasitoid Aphelinus mali (Haldeman), the colonies formed on the roots are difficult to control, so the use of resistant rootstocks is advantageous for controlling this pest (Sandanayaka et al., 2003). In addition, as more and more growers are implementing low pesticide control or adopting eco-friendly cultivation for environmental conservation and sustainable agriculture, the importance of WAA-resistant rootstocks is gradually increasing.
This study evaluated Cornell-Geneva apple rootstocks, with known resistance to fire blight (Erwinia amylovora), and standard rootstocks M26 and M9 for their susceptibility to soil-borne diseases and investigated how resistance to WAA varies depending on rootstock type.
Materials and Methods
This study was carried out in a greenhouse on the experimental fields of Kyungpook National University, Gun-Wi, Korea (36°06'N, 128°38'E) in 2019. Six types of the G and CG rootstock series from Cornell University and the industry-standard apple rootstocks M26 and M9 were investigated.
On February 27, 2019, 1-year-old plants of each rootstock type harvested from stool-beds were planted in 2-L pots filled with horticultural growing media (72% coco peat, 8.5% peat moss, 6% vermiculite, 9.5% perlite). After planting and managing 130 trees per rootstock type, 120 trees were selected, except for the dead or weak trees, and 30 trees were used for each of the four resistance tests against P. cactorum, R. necatrix, A. rolfsii, and WAA [E. lanigerum (Huasm.)]. The P. cactorum, R. necatrix, and A. rolfsii used in this study were obtained from the Korean Agricultural Culture Collection (KACC, Jeonju, Korea). Phytophthora cactorum (V8-agar medium) and A. rolfsii (potato dextrose agar medium) were incubated at 25°C for 2 weeks, and R. necatrix (potato dextrose agar medium) for 1 week in an incubator (MIR-154, Panasonic). Pathogens were inoculated on August 13, the 167th day after planting. After that, chemical control and nutrient fertilization were discontinued in all treatments.
Prior to releasing WAA, acaricides were applied twice to control the mass emergence of Panonychus ulmi and Tetranychus urticae (Acari: Tetranychidae) in the WAA experimental block. A screen was installed with white silk to prevent the infestation of the natural enemy A. mali, and the trees were cultivated inside.
Inoculation Methods
P. cactorum was inoculated directly in the stem cambium. The stem bark was peeled off at a height of about 2 cm above-ground with a scalpel, and the mycelia on the V8-agar medium were extracted using a cork borer (8.0 mm in diameter). A media disk was inserted into the bark. The inoculation site was wrapped with sterilized wet gauze that was overlaid with a black cloth to provide dark conditions, followed by polyethylene grafting tape to prevent evaporation.
R. necatrix infection wasinduced in the soil using mulberry (Morus alba L.) branches (about 1 cm in diameter and 5 cm in length) as host plants. After incubating the pathogen for 1 week in the incubator, host infection was induced in the dark by placing sterilized (autoclaved at 120°C, 20 min) mulberry branches on the potato dextrose agar medium of Petri dishes filled with mycelia. Five days later, the host plants covered with mycelia were inserted into the soil at a distance of 5 cm from the trunk (one per pot).
A. rolfsii was inoculated on the ground surface around the trunk using the sclerotia formed on the medium. A scalpel was used to cut two parts of the trunk on the ground surface, and then five sclerotia were inoculated per pot. The sclerotia were covered with wet gauze to prevent drying and the gauze overlaid with a black cloth to provide dark conditions.
On June 6, sufficient colonies were collected in WAA-infested apple orchards and used for resistance testing. On June 7, highly active individuals, isolated from the collected WAA colonies using a desktop magnifying glass with a light, were released to the ground surface around the trunk (10 WAA per pot).
Measurements
Forty days after P. cactorum inoculation, the stem bark was peeled off with a scalpel, and the length of the developed lesion was measured with a digital Vernier caliper (CD-20CPX, Mitutoyo). An additional 20 days later, the roots were removed, the below-ground stem bark was peeled off, and the infection was examined as in the above-ground investigation. After 40 days of R. necatrix inoculation, the roots were removed, the stem bark was scraped off with a razor blade, and the length of the lesion distribution was measured using the digital Vernier caliper. The lesion index was expressed by dividing the infected area into five levels (1: 1–20%, 2: 21–40%, 3: 41–60%, 4: 61–80%, 5: 81–100%) and compared with the healthy area within the lesion distribution range. After 10 days of A. rolfsii inoculation, the length of the stem infection was measured. Twenty days later, further diffusion was examined. Forty days after inoculation, roots were removed, and the length of the below-ground stem infection was measured. Dead trees were examined at the end of the experiment.
The infestation of WAA into the roots and shoots was investigated on January 10, after defoliation. WAA settlement rate and the number of colonies formed on shoots were recorded. Colony size was measured using the digital Vernier caliper. The infestation of WAA into the roots was expressed as a percentage through visual inspection.
Data were analyzed by analysis of variance (ANOVA) using SAS 9.4 software (SAS Institute, Inc., Cary, NC, USA). Significant differences among the rootstocks were evaluated by Duncan's multiple range test at p < 0.05.
Results
Phytophthora Root Rot (Phytophthora cactorum)
There was a marked difference in the length of lesions developed after 40 days of P. cactorum inoculation among rootstock types (Fig. 1). M series rootstocks showed relatively high resistance. After 40 days of inoculation, the length of the lesion developed from the initial infection site toward the upper stem was the longest for CG5087 (55.8 mm), followed by G935 (55.6 mm), CG4814 (49.1 mm), G202 (42.2 mm), and G11 (28.6 mm). M9 had the shortest infection length of 13.2 mm. After 60 days of inoculation, the below-ground stem lesions were less developed than the above-ground stem lesions. The below-ground lesion length was 17.9 mm for CG4814 and 1.6 mm for M9.
White Root Rot (Rosellinia necatrix)
Unlike the trend of resistance to P. cactorum, M series rootstocks tended to be slightly more sensitive to R. necatrix disease than the G or CG rootstocks (Fig. 2). M9 and M26 exhibited lesion lengths of 65.3 and 58.6 mm, respectively, followed by CG5087 (58.2 mm), G214 (47.3 mm), G202 (45.3 mm), G935 (42.0 mm), CG4814 (38.0 mm), and G11 (37.1 mm). The lesion index was 3.6 for M26, which had the largest stem infection area, and 3.3 for M9 and CG5087, separately. G202 and CG4814 recorded 2.1 and 2.0, respectively, showing differences among rootstocks in the rate of disease development.
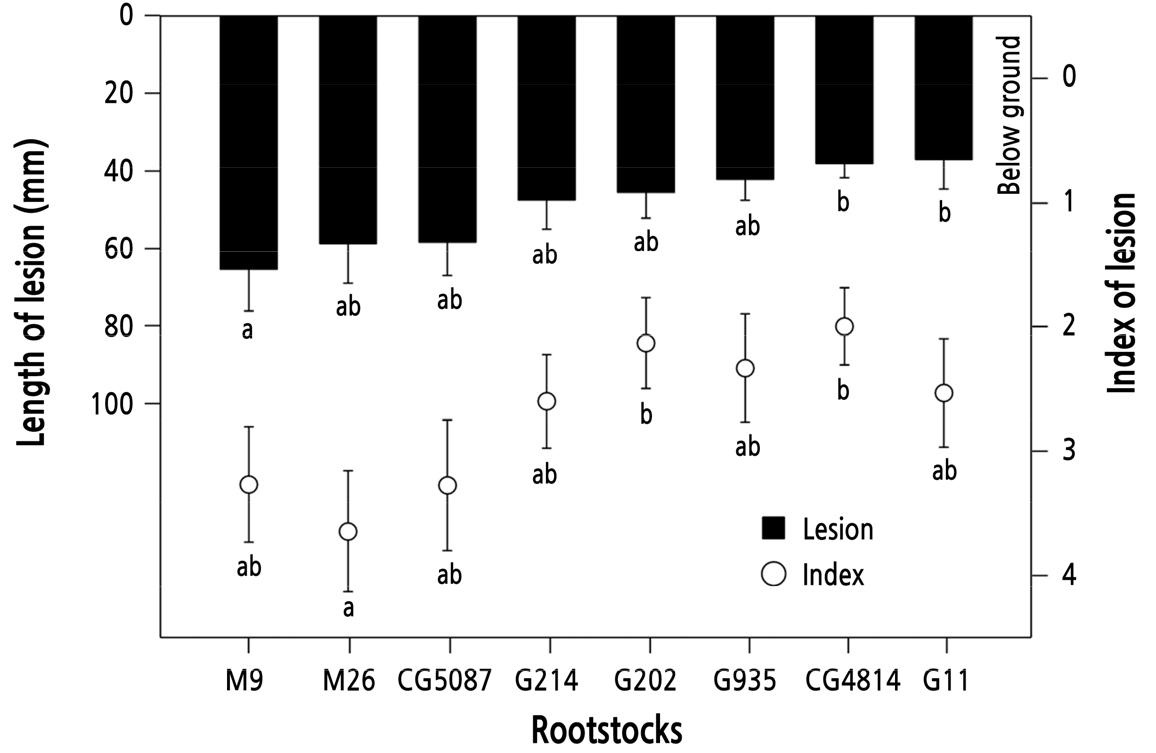
Fig. 2.
Length of spot-shaped lesions spread on the below-ground stem of 1-year-old rootstocks after soil inoculation with mulberry host plants covered with Rosellinia necatrix mycelium, and the lesion index. Infected area classification was 1: 1–20%, 2: 21–40%, 3: 41–60%, 4: 61–80%, 5:81–100%. Vertical bars represent standard errors of the means. Bars with different letters are significantly different at p < 0.05 by Duncan's multiple range test.
Southern Blight (Athelia rolfsii)
A. rolfsii disease was more severe in M series than in G or CG series (Fig. 3). After 10 days of inoculation, the lesion lengths were 6, 9, 20, and 22 mm for G935, CG5087, M26, and M9, respectively. After 30 days of inoculation, there were some differences among rootstocks in the rate of infection development. Lesion lengths among the rootstocks increased in the order of G214 (2 mm), CG5087 (3 mm), G935 (3 mm), G202 (4 mm), CG4814 (7 mm), and M26 (8 mm). Values of 13 and 23 mm were recorded for G11 and M9, respectively, and their spread of pathogens was relatively larger than that of other rootstocks. After 40 days of inoculation, M9 and M26 had 108 and 127 mm of below-ground stem infection, respectively, resulting in a markedly faster infection rate than other rootstocks. The lesion lengths of G935, G11, G202, CG5087, G214, and CG4814 were 10, 11, 23, 24, 35, and 36, mm, respectively, but were not statistically significant. Rootstock mortality was 80% for M9 and 53% for M26, followed by CG5087 (25%), G202 (23%), CG4814 (20%), G214 (17%), G11 (10%), and G935 (7%).
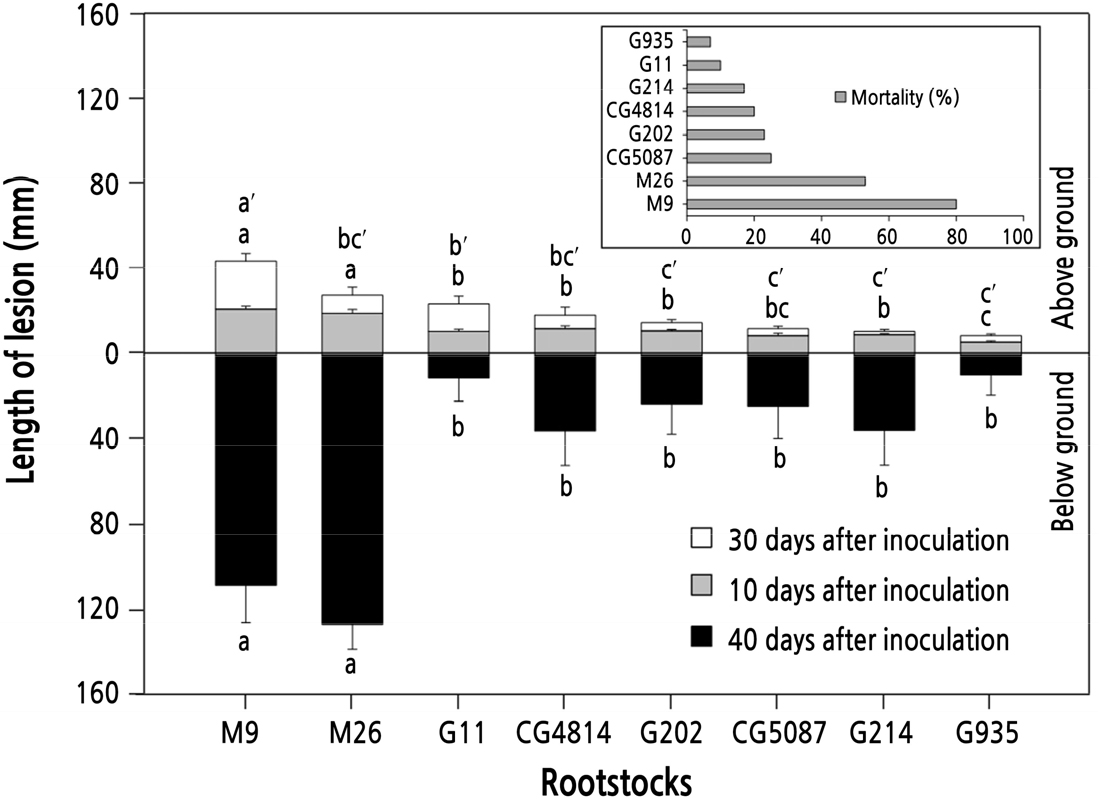
Fig. 3.
Length of the lesions developed on the stem of 1-year-old rootstocks after inoculation with Athelia rolfsii on the ground surface, and rootstock mortality. Vertical bars represent standard errors of the means. Bars with different letters are significantly different at p < 0.05 by Duncan's multiple range test.
Woolly Apple Aphid [Eriosoma lanigerum (Huasm.)]
WAA susceptibility was dramatically different by rootstock type (Table 1). CG4814 had the highest proportion of settled WAA of 57%, followed by M26 (53%), M9 (47%), and G935 (40%). No colony formation was observed on G11, G202, G214, and CG5087, but the number of colonies formed on M9, CG4814, M26, and G935 was 8.6, 7.4, 7.2, and 6.5, respectively. The size per colony formed on M9 was 32.6 mm, which showed a large difference compared with other rootstocks. Root infestation by WAA was most prevalent in CG4814, with 47% infested, followed by M9 (40%), M26 (33%), and G935 (23%).
Table 1.
Infestation level on shoots and roots by rootstock type, about 7 months after release of woolly apple aphid on the ground surface of the pots
Rootstock | On a whole-canopy basis | Root infestation (%) | ||
Settlementz (%) | Number of colonies | Colony size (mm) | ||
G11 | 0 | 0.0 by | 0.0 c | 0 |
G202 | 0 | 0.0 b | 0.0 c | 0 |
G214 | 0 | 0.0 b | 0.0 c | 0 |
G935 | 40 | 6.5 a | 11.9 b | 23 |
CG4814 | 57 | 7.4 a | 11.1 b | 47 |
CG5087 | 0 | 0.0 b | 0.0 c | 0 |
M26 | 53 | 7.2 a | 9.4 b | 33 |
M9 | 47 | 8.6 a | 32.6 a | 40 |
Discussion
Soil-borne Diseases
P. cactorum, R. necatrix,and A. rolfsii are soil-borne pathogens that attack roots and crowns (Freeman et al., 1990; Tidball and Linderman, 1990; Mahadevakumar et al., 2018). Phytophthora root rot, white root rot, and southern blight caused by these organisms are difficult to control completely chemically, and if the disease prevails in orchards, trees with low resistance may lose their viability, resulting in economic losses. In this study, infection symptoms appeared in various forms according to the pathogen, and the relative resistance among rootstocks was evaluated by measuring the lesion lengths (Utkhede and Quamme, 1988). P. cactorum is encouraged in situations where soil drainage is poor and can spread to other areas by nursery apple trees (Campbell, 1969; Jeffers and Aldwinckle, 1988). After inoculation of P. cactorum into the cambium, the infection progressed to the upper part of the trunk, forming a black band along the transpiration stream (Fig. 1). Marked differences in resistance among the rootstocks were observed, and, as reported by Browne and Mircetich (1993), M9 exhibited relatively high resistance. Among G or CG rootstocks, G11 showed disease resistance comparable to M9. Other rootstocks, such as CG5087, G935, CG4814, G202, and G214, could be classified as relatively sensitive.
R. necatrix infects various plants either as a diffuse mycelium or in the form of hyphal strands (Sztejnberg et al., 1987). Roots infected with R. necatrix in apple trees are covered with fan-shaped mycelia, and the mycelia penetrate the cortical parenchyma under the epidermis, causing tissue decay (Schena et al., 2008). Proper moisture and temperature in the soil are factors that promote fungal growth and provide environment conditions in which fungi can parasitize if dead vegetable residues, such as roots and branches, remain in the soil (Pérez-Jiménez, 2006). This study confirmed that rhizosphere could easily be infected by R. necatrix via a host plant. The susceptibility to R. necatrix was slightly higher in M9 and M26 than in G or CG rootstocks (p < 0.05), indicating that the inherent resistance of rootstocks may vary depending on the type of disease (Fig. 2).
A. rolfsii disease is a destructive pathogen that infects a wide range of plant species and forms a sclerotium, which allows it to survive for a long time, even in soil (Punja, 1988). There are few reports of A. rolfsii in apples, but the incidence increases when tree residues remain in the soil (Conway et al., 1996). Regarding the susceptibility of rootstocksto A. rolfsii, the pathogen distribution tended to be relatively suppressed in the G or CG rootstocks, unlike the high sensitivity of M rootstocks, thereby suggesting that there was a difference in resistance among the rootstocks (Fig. 3). Specifically, it is thought that there is a significant difference (p < 0.05) in resistance between G935 and M26 or M9.
All rootstocks showed incomplete resistance to three of these diseases, and even rootstocks with relatively high resistance did not seem to imply that they had immunity. In conclusion, the resistance of rootstocks may vary depending on the pathogen, and resistant rootstocks are somewhat expected to be able to inhibit infection or spread in trees. G11 was evaluated as a rootstock that showed common resistance to all three pathogens.
Woolly Apple Aphid (WAA)
During the growth of apple trees, WAA infests shoots and roots to form colonies and has a life cycle that overwinters in trunk cracks and the rhizosphere (Gontijo, 2011). Therefore, the susceptibility of rootstocks can be estimated by examining WAA activity according to size and number of colonies formed on the trunk, as well as the root infestation (Ateyyat and Al-Antary, 2009). Trees where the WAA settled were clearly distinguished among the rootstocks, and the colony size was remarkable in M9 (Table 1). As already known, M9 and M26 showed relatively high susceptibility to WAA (Webster and Wertheim, 2003) in this study. Among the promising elite rootstocks, G935 (Robinson et al., 2014) and CG4814 were highly susceptible. This outcome can be attributed to the vulnerability of 'Ottawa 3' to WAA, one parent of the G935 and CG4814 rootstocks (Spangelo et al., 1974). In conclusion, the resistance to WAA differed noticeably among the rootstock types. G11, G202, G214, and CG5087 rootstocks seemed to have immunity against WAA.