Introduction
Materials and Methods
Plant Material
Light-quality Treatments and Growth Conditions
Determination of Morphological Characteristics
Pigment Content Measurements and Leaf Colour Analysis
Statistical Analysis
Results
Influence of Light Quality on Growth and Morphogenesis
Influence of Light Quality on Pigment Levels and Leaf Colour
Discussion
Light Quality Affects the Growth Development of Sedum rubrotinctum Tissue-culture-generated Plantlets
Different Light Quality Treatments Affect the Quality of Sedum rubrotinctum Tissue-culture-generated Plantlets
Conclusions
Introduction
The Sedum rubrotinctum plant is a perennial species that is a member of the Crassulaceae family, which is the third-largest family of succulent plant species. Gardening aficionados have a great deal of interest in S. rubrotinctum plants because they are resistant to drought, have tremendous vitality, are simple to handle, and have fleshy leaves that can change from green to vivid red (Ma et al., 2019). This species is used not only as an indoor potted plant but also for the landscaping of rock gardens, roof gardens or as a groundcover plant (Tuttolomondo et al., 2018). Thus, the market demand for S. rubrotinctum is increasing. To meet this demand, a large amount of S. rubrotinctum material must be rapidly grown and propagated in a short amount of time. However, traditional plant propagation methods such as seed propagation, stem-cutting propagules, and leaf-cutting propagules require long periods of time, especially during the winter (Aprile et al., 2020). Compared to conventional horticultural propagation methods, plant tissue culture can be used to generate a large number of plants under controlled conditions independent of the season, shortening breeding times (Jan et al., 2018; Osman and Awal, 2023). An essential environmental component that influences growth, morphogenesis, and physiology of the plants, is the light spectrum, especially considering its varying wavelengths. Plants have evolved a suite of photoreceptors (i.e., phytochromes, cryptochromes, phototropins, and UV-B receptors) that react to different wavelengths of light (Rai et al., 2021). Generally, red (R) and far-red (600–750 nm) light are detected by phytochromes, blue (B) and UV-A (320–500 nm) light are detected by cryptochromes and phototropins, and UV-B (290–320 nm) light is detected by UV-B receptors (Jones, 2018). Photoreceptors are activated by different wavelengths of light, and activated photoreceptors then transmit light signals through a cascade to modulate the expression of multiple genes that ultimately regulate several physiological processes (Yadav et al., 2020).
Many studies have shown that the quality of light can affect plant growth and development, even leaf colour and pigment contents, via the selective activation of different photoreceptors (Tian et al., 2019; Lin et al., 2021; Paradiso and Proietti, 2022). Thus, light quality can be used to manipulate the architecture of plants and induce beneficial characteristics. Plant responses to light quality depend on the species because various plant species have distinct light quality needs (Liu et al., 2018; Chiang et al., 2020), and it is important to identify the most beneficial light quality components and ratios for plant growth. Light-emitting diodes (LEDs) have the advantage of emitting specific wavelengths and thus allowing specific light quality combinations, and LEDs have been widely used to provide light quality combinations adjusted specifically to promote the growth and development of plants in controlled environments, such as plant tissue culture and factory seedling cultivation environments (Sipos et al., 2020). B (420–450 nm) and R (600–700 nm) light types are absorbed by chlorophyll a (Chl a), which has absorption peaks at 430 and 665 nm, and chlorophyll b (Chl b), which has absorption peaks at 453 nm and 642 nm. As such, wavelengths of R light and B light are efficiently absorbed by primary plant pigments (chlorophyll [Chl]); thus, the use of R and B LED lighting has been the primary choice of producers (Ouzounis et al., 2015).
Many experiments have been conducted to study the effects of various R/B LED light combinations on tissue-culture-generated plant species, such as Oryza sativa L. (Yu et al., 2020), Stevia rebaudiana Bertoni (Ramírez-Mosqueda et al., 2017), Rehmannia glutinosa (Manivannan et al., 2015), Dracocephalum forrestii (Weremczuk-Jeżyna et al., 2021), and Dendrobium nobile ‘Zixia’ (Guo et al., 2023). However, there have been few studies of the effects of R/B LED light combinations on the growth of succulent plants in vitro and on the colour changes of plant leaves.
The aim of the current study is to analyze how various lighting conditions affect the development, morphology, and leaf colour of S. rubrotinctum plantlets grown in tissue culture. The results of this research will be useful when determining which aspects and proportions of lighting are optimal for the development and production of vibrant colours in S. rubrotinctum. This research may be used as a standard for determining how much and what types of light S. rubrotinctum and similar succulents need to thrive, which is practically significant for the production of S. rubrotinctum.
Materials and Methods
Plant Material
This experiment was conducted at the School of Biological and Environmental Engineering of Jingdezhen University, China. Second-generation healthy and disease-free S. rubrotinctum plantlets generated via tissue culturing at the Plant Tissue Culture Laboratory at Jingdezhen University, China, were selected. Amounts of approximately 2 cm of the top portions of the selected tissue-culture-generated plantlets were removed with sterilized scissors on an ultraclean workbench. The size of the removed top portions of the S. rubrotinctum tissue culture-generated plantlets identical to that of the test material.
Light-quality Treatments and Growth Conditions
Light-quality experiments were conducted at the Plant Tissue Culture Laboratory at Jingdezhen University. Six composite LED light treatments, R (pure red), 4R1B (red: blue = 4:1), 3R1B (red: blue = 3:1), 2R4B (red: blue = 2:4), B (pure blue) and W (white) were used in this study. The LED light peaks are as follows: red 630–660 nm, blue 465–470 nm, and white 380–760nm. Light sources were purchased from Shenzhen Lingyun Technology Co., Ltd. Each shelf of the tissue culture rack utilized the same light intensity (PPFD of 400 ± 50 µmol·m-2·s-1) with different number of lamps, and the different shelves were separated by thick sheets of paper. S. rubrotinctum plantlets used as explants were incubated on Murashige and Skoog (MS) solid media supplemented with 0.5 mg·L-1 1-naphthaleneacetic acid (NAA) under the six LED light treatments. The removed tops of the tissue-culture-generated samples in each treatment included three replications with ten culture flasks per replicate, and each culture flask contained one explant. The environment had a relative humidity of 80 ± 5%, a photoperiod of 16 h light/8 h darkness, and daytime/nighttime temperatures of 25 ± 2/15 ± 2°C.
Determination of Morphological Characteristics
After 90 days of cultivation, ten tissue-culture-generated S. rubrotinctum plantlets were randomly selected from each treatment and subjected to a morphological characterization. The roots of the S. rubrotinctum tissue-culture-generated plantlets were carefully washed with deionized water to remove any adhering media. The shoots and roots were subsequently blotted dry and weighed separately with an electronic balance to determine their fresh weight (FW). Plantlet height, crown width, and stem diameter were measured with Vernier calipers, and the total number of leaves per plantlet was determined. The leaf length, leaf width, leaf thickness and leaf area were measured with a YMJ-C smart leaf area meter (Tuopu Instrument, China), and the root parameters of the total root length, root surface area, average diameter and root volume were analyzed via WinRHIZO 2015 (Regent Instruments, Canada).
Pigment Content Measurements and Leaf Colour Analysis
Five tissue-culture-generated plantlets were randomly selected from each light-quality treatment, and five leaves were randomly selected from each plantlet to determine the pigment levels and chromaticity of the leaves. The photosynthetic pigments (Chl a, Chl b, total Chl and carotenoids) were extracted from fresh leaves (0.1 g) with acetone according to the method of El-Saadony et al. (2021). To determine the anthocyanin concentrations in the leaves, fresh leaves were extracted in 1 mL of acidic (1% HCl) methanol and the optical density was measured at 530 nm (OD530) and 657 nm (OD657) for each sample. Anthocyanin contents were calculated according to Yao et al. (2017). The levels of Chl a, Chl b, total Chl, and carotenoids were calculated according to Fadeel (1962), the formulas in which are as follows:
Chl a content (mg·g-1) = (9.78 × A662–0.99A644)V/(W × 1000);
Chl b content (mg·g-1) = (21.4 × A644–4.65 × A662)V/(W × 1000);
Total Chl content (mg·g-1) = Chl a content + Chl b content;
Carotenoid content (mg·g-1) = (4.69 × A440–total Chl content × 0.268)V/(W × 1000);
Anthocyanin content (mg·g-1) = (A530–0.25A 657)V/(W × 1000).
In these equations, V represents the extraction volume (mL) and W represents the weight of a tissue sample (g).
The objective colour of the plantlet leaves (the third leaf from the top of the shoots) was evaluated using a colorimeter (Bangyes3NH, Shanghai China); the colour measurements included three elements (L*, a*, and b*). L* corresponds to the lightness, and a* indicates the R/green chromaticity, with ‘+’ values indicating ‘redness’ and ‘–’ values indicating ‘greenness’. b* indicates yellow/B chromaticity, with ‘+’ values indicating ‘yellowness’ and ‘–’ indicating ‘blueness’.
Statistical Analysis
The experimental data were analyzed via a standard analysis of variance (ANOVA) followed by Duncan multiple comparison tests (p < 0.05) with SPSS version 20.0, and SigmaPlot (v12.5, SYSTAT) software was used for mapping. The principal component analysis (PCA) and correlation analysis conducted in this study were done with Origin 2021.
Results
Influence of Light Quality on Growth and Morphogenesis
Fresh weight (FW) and select morphological characteristics
After the plantlets were cultured for 90 days, significant differences in plantlet morphologies were observed among the six different light-quality treatments. The tissue-culture-generated S. rubrotinctum plantlets grown under the 4R1B treatment presented the greatest values of the shoot fresh weight (FW), root FW, total FW and shoot/root FW, while the plants grown under the R LED light presented the lowest values of these parameters. The plantlet height was 51.61 mm per plantlet, which was highest under the R LED light, followed by the 2R4B and B treatments, which were 38.35 mm and 37.86 mm per plantlet, respectively. The plantlets under the 3R1B, 4R1B and W LED light treatments were significantly shorter. The greatest values of the stem diameter and crown width were obtained under the 4R1B treatment and the 2R4B treatment, respectively, and the lowest values were observed under the R treatment. Compared to the plantlets grown under the monochromatic light treatments (pure R, pure B) and pure white [W], the plantlets grown under the different combinations of R/B light presented greater shoot FW, root FW, total FW, stem diameter and crown width values (Table 1).
Table 1.
Effects of different light-quality treatments on the growth parameters of Sedum rubrotinctum tissue-culture-generated plantlets
Treatmentsz |
Shoot FW (g) |
Root FW (g) |
Total FW (g) |
Shoot/ root FW |
Plantlet height (mm) |
Stem diameter (mm) |
Crown width (mm) |
R | 1.41 ± 0.03ydz | 0.22 ± 0.01 d | 1.63 ± 0.04 d | 6.34 ± 0.07 c | 51.61 ± 1.46 a | 1.51 ± 0.04 d | 19.03 ± 1.00 d |
4R1B | 3.24 ± 0.10 a | 0.40 ± 0.01 a | 3.64 ± 0.97 a | 8.12 ± 0.19 a | 33.51 ± 0.67 c | 2.50 ± 0.06 a | 29.46 ± 0.46 ab |
3R1B | 2.54 ± 0.08 b | 0.37 ± 0.02 ab | 2.91 ± 0.10 b | 6.98 ± 0.37 bc | 31.48 ± 1.05 c | 2.15 ± 0.05 b | 29.40 ± 0.69 ab |
2R4B | 2.66 ± 0.06 b | 0.35 ± 0.01 b | 3.01 ± 0.06 b | 7.59 ± 0.32 ab | 38.35 ± 0.64 b | 2.39 ± 0.21 a | 31.28 ± 0.46 a |
B | 1.78 ± 0.05 c | 0.28 ± 0.01 c | 2.06 ± 0.06 c | 6.48 ± 0.21 c | 37.86 ± 1.35 b | 1.63 ± 0.05 d | 26.85 ± 0.57 c |
W | 1.84 ± 0.08 c | 0.27 ± 0.01 c | 2.11 ± 0.08 c | 6.87 ± 0.22 bc | 34.10 ± 0.70 c | 1.96 ± 0.05 c | 27.33 ± 1.09 bc |
Morphological characteristics of leaves
The ANOVA results showed that there were differences in the morphological characteristics of the leaves of the plantlets among the different light treatments (Table 2). The R and B treatments resulted in fewer leaves per plant compared to the other LED light treatments; these outcomes were 19.4 and 21.20 per plantlet, respectively, and the 4R1B treatment resulted in the greatest number of leaves per plant at 30.90. The leaf length, leaf thickness, leaf area and number of leaves per plantlet were greatest under the 4R1B treatment. Although the leaf width value was highest under the 2R4B treatment, the leaf width did not differ significantly under the 2R4B treatment and the 4R1B treatment (Table 2).
Table 2.
Effects of different light-quality treatments on the parameters of leaves of Sedum rubrotinctum tissue-culture-generated plantlets
Treatmentsz |
Number of leaves per plantlet |
Leaf length (mm) |
Leaf width (mm) |
Leaf thickness (mm) |
Leaf area (mm2) |
R | 19.40 ± 0.90ycx | 9.92 ± 0.28 d | 5.17 ± 0.04 d | 3.08 ± 0.09 c | 44.01 ± 0.83 e |
4R1B | 30.90 ± 0.48 a | 14.41 ± 0.28 a | 5.59 ± 0.48 ab | 4.07 ± 0.09 a | 76.68 ± 1.67 a |
3R1B | 25.20 ± 0.47 b | 13.09 ± 0.23 b | 5.34 ± 0.69 cd | 3.67 ± 0.13 b | 64.36 ± 1.52 bc |
2R4B | 25.00 ± 0.49 b | 13.82 ± 0.24 ab | 5.72 ± 0.48 a | 4.01 ± 0.04 a | 67.26 ± 1.06 b |
B | 21.20 ± 0.84 c | 11.66 ± 0.19 c | 5.26 ± 0.13 cd | 3.35 ± 0.05 b | 52.62 ± 1.26 d |
W | 25.30 ± 0.80 b | 12.90 ± 0.43 b | 5.42 ± 0.11 bc | 3.62 ± 0.12 b | 62.89 ± 1.32 c |
Morphological characteristics of the roots
As shown in Table 3, the different light quality treatments had significant effects on the root development of the S. rubrotinctum tissue-culture-generated plantlets (p < 0.05). The total root length, root surface area and root volume were greatest under the 4R1B and 3R1B combined conditions compared to any of the single monochromatic light condition. Maximum values of 6.74 cm, 8.14 cm2, 5.18 mm, and 1.66 cm for the total root length, root surface area, average root diameter, and root volume, respectively, were recorded under the 4R1B treatment, correspondingly greater by 49.40%, 36.86%, 5.41%, and 60.24% than those under the R treatment group (for which the lowest values were recorded).
Table 3.
Effects of different light-quality treatments on the parameters of roots of Sedum rubrotinctum tissue-culture-generated plantlets
Treatmentsz |
Total root length (cm) |
Root surface area (cm2) | Average root diameter (mm) |
Root volume (cm3) |
R | 3.41 ± 0.48yez | 5.14 ± 0.42 d | 4.90 ± 0.24 ab | 0.66 ± 0.07 d |
4R1B | 6.74 ± 0.37 a | 8.14 ± 0.21 a | 5.18 ± 0.23 a | 1.66 ± 0.07 a |
3R1B | 6.19 ± 0.37 ab | 7.42 ± 0.17 ab | 4.70 ± 0.23 ab | 1.38 ± 0.09 ab |
2R4B | 5.33 ± 0.39 bc | 7.07 ± 0.22 b | 4.44 ± 0.12 b | 1.16 ± 0.11 bc |
B | 3.88 ± 0.38 ed | 5.78 ± 0.44 cd | 4.79 ± 0.40 ab | 0.79 ± 0.07 cd |
W | 4.61 ± 0.32 cd | 6.53 ± 0.30 bc | 4.68 ± 0.27 ab | 0.96 ± 0.12 cd |
Influence of Light Quality on Pigment Levels and Leaf Colour
The present study also included an evaluation of the effects of the various LED light treatments on the pigment levels in S. rubrotinctum leaves and on the leaf colour. As shown in Fig. 1, significant differences in the levels of pigments, in this case the levels of Chl a, Chl b, total Chl, and anthocyanins, and the Chl a/b ratio were observed among the plantlets under the six different light-quality treatments, while there were no significant differences in the carotenoid content (Fig. 1A to 1F). The 4R1B treatment resulted in the greatest Chl a, Chl b, total Chl and carotenoid levels at 0.52 mg·g-1, 0.35 mg·g-1, 0.87 mg·g-1, and 0.29 mg·g-1, respectively, followed by the 2R4B treatment. The R and B treatments yielded lower Chl a, Chl b, total Chl and anthocyanin contents, while the maximum Chl a/b ratios were found under the R LED light treatments.
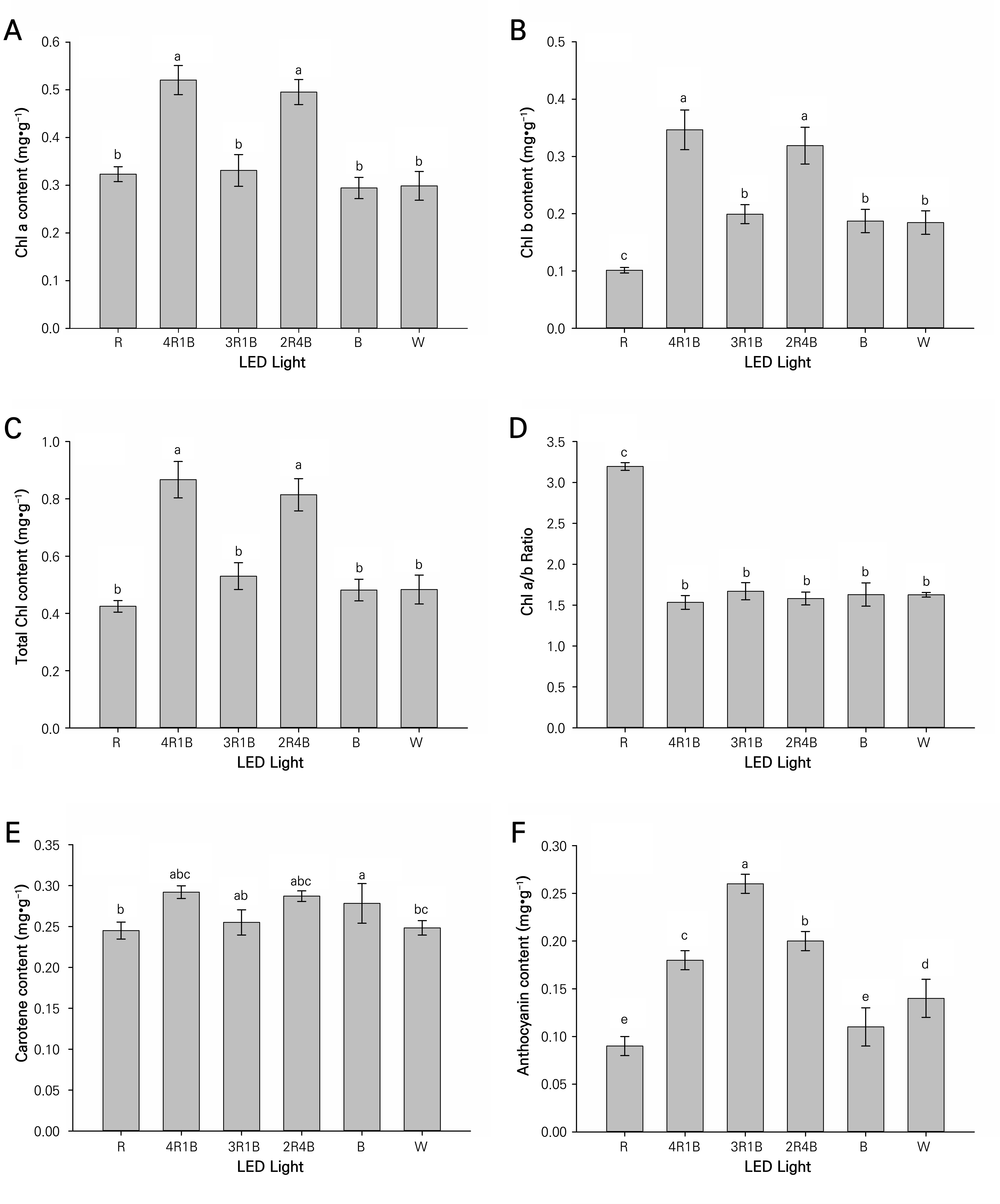
Fig. 1.
Pigment levels in leaves of Sedum rubrotinctum under different light-quality treatments. (A) Chl a content, (B) Chl b content, (C) total Chl content, (D) Chl a/b ratio, (E) carotenoid content, and (F) anthocyanin content. The different letters indicate significant differences between the different treatments. Data are means ± standard errors (n = 5).
The R/B LED light combination resulted in relatively high levels of anthocyanins, a finding in contrast to the low level obtained under monochromatic light. The greatest anthocyanin content was observed under the 3R1B treatment, showing corresponding increases of 65.38%, 57.69%, and 46.15% compared to those under the R, B and W treatments. Moreover, the different light-quality treatments significantly affected the L* and a* values of the colour readings (L* value: F = 3.64, p < 0.05; a* value: F = 53.97, p < 0.001). The S. rubrotinctum leaves under the W treatment presented the greatest L* values, followed by those under the 4R1B, 3R1B, and R treatments, and the minimum value was recorded under the 2R4B treatment. The leaves under the 3R1B treatment presented the greatest a* values, which were ranked as follows: 3R1B > 2R4B > 4R1B > W > B > R. No significant effects of the light quality on b* values were found (b* value: F = 2.34, p > 0.05) (Fig. 2A). The leaves of S. rubrotinctum grown under the 3R1B, 2R4B and 4R1B treatments were obviously red, with the colour encompassing the whole blade or the edge of the blade, and the leaves were the reddest under the 3R1B treatment. The leaves under monochromatic light (R, B) were green, and no visible red colour in these leaves was observed (Fig. 2B).
PCA
Figure 3 shows a classification model of the growth of S. rubrotinctum plantlets grown from tissue culture under different light-quality conditions. This model is based on the results of a principal component analysis (PCA) to give a better visual representation. Two principal components explained 86.3% of the total variance, with PC1 and PC2 accounting for 70.5% and 15.8%, respectively. Additionally, the loading plots revealed that the leaf thickness, leaf area, and the total FW and Chl b contents were the main contributors to the first principal component, while the shoot/root FW and crown diameter were the main contributors to the second principal component. Therefore, these parameters were used as proxies for the quality of S. rubrotinctum tissue-culture-generated plantlets in response to the different light-quality treatments. Moreover, the R, B, 2R4B, 3R1B, 4R1B and W treatment groups were clearly separated on the basis of PC1 and PC2. In the PCA plots, both the R and B treatment groups were located in the second quadrant, and both the 2R4B and 4R1B treatment groups were located in the first quadrant, indicating that these treatments had similar effects on the quality of S. rubrotinctum tissue-culture-generated plantlets. The W treatment group was located in the third quadrant, and the 3R1B treatment group was located in the fourth quadrant.
To compare the differences in the growth of S. rubrotinctum tissue-culture-generated plantlets under different light-quality conditions further, according to the notion that the cumulative contribution percentage exceeds 85%, two principal components were obtained after a dimensionality reduction of each index. Thus, the comprehensive scores were weighted by the variance contribution of each component and calculated as follows: comprehensive score (F) = X1 × 0.705 + X2 × 0.158, where X1 is the score of the first principal component and X2 is the score of the second principal component. Fig. 4 shows the comprehensive scores of the growth of S. rubrotinctum tissue-culture-generated plantlets under the six different light-quality treatments. When an overall score of 0 is used as a benchmark, positive values indicate improved quality and negative values indicate poorer quality. The six treatments were ranked as follows: 4R1B > 2R4B > 3R1B > W > B > R. Consequently, the light quality associated with the 4R1B treatment was considered to be the best for the growth of S. rubrotinctum tissue-culture-generated plantlets.
Correlation analysis
The correlations between different morphological traits, pigment levels and between pigment levels and leaf colour attributes of S. rubrotinctum tissue-culture-generated plantlets grown under different light quality combinations are shown in Fig. 5, which indicates that the both the shoot FW and total FW were significantly positively correlated with most morphological traits and pigment levels, in this case the stem diameter, leaf width, root surface area, the Chl a/b ratio, the carotenoid content and anthocyanin content, but not the shoot/root FW. However, according to these analyses, there were several significant positive correlations between the morphological traits and Chl content, specifically the shoot FW, total FW, shoot/root FW, stem diameter, leaf width, and leaf thickness, all of which were significantly positively correlated with the Chl a, Chl b and total Chl contents. Among these traits, a highly significant positive correlation was detected between the shoot/root FW and Chl b content (r = 0.98), and strong correlations between the leaf thickness and Chl b content were also detected (r = 0.97). However, most of the growth attributes were negatively correlated with the Chl a/b ratio, and no significant correlations were detected between any morphological trait and the Chl content (Fig. 5). However, a highly significant positive correlation was also found between the anthocyanin content and the a* value (r = 0.96), and Chl a content, Chl b content, total Chl content, the Chl a/b ratio were not significantly correlated with the L* value or a* value (Fig. 6).
Discussion
Light Quality Affects the Growth Development of Sedum rubrotinctum Tissue-culture-generated Plantlets
Light is not only a source of energy but also an essential signal for plant growth and development. Plant detection and regulation of light signals are controlled by a network of photoreceptors, in particular phytochromes (red/far-red receptors, which absorb wavelengths of 600–750 nm), cryptochromes and phototropins (blue/UV-A light receptors, which absorb wavelengths of 320–500 nm) and UVR8 (a UV-B light receptor, which absorb wavelengths of 280–320 nm) (Paik and Huq, 2019). Thus, different light quality combinations induce different morphogenetic responses in plants.
With respect to plant biomass, our results showed that shoot FW, total FW and shoot/root FW were similarly affected by the different light treatments. The treatments were ranked as follows: 4R1B > 2R4B > 3R1B > W > B > R. In addition, the root FW was affected by the light treatments in the order of 4R1B > 3R1B > 2R4B > W > B > R, demonstrating that B light had a stronger effect on biomass accumulation and that biomass exhibited a clear increasing trend under the R/B light combination compared to monochromatic light. Moreover, the increase in shoot biomass of S. rubrotinctum was greater than the increase in root biomass. Similar results were reported in studies of the succulent plant species Mesembryanthemum crystallinum and tomato; the results showed that seedling biomass clearly increased under the combined R/B LED light treatment compared to monochromic R and B light (He et al., 2019; Li et al., 2021b). This phenomenon could be attributed to plants growing under R/B light having the greatest photosynthetic efficiency, as this wavelength range coincides closely with the absorption peaks of Chl. Owing to the greater phytochrome content under R light, which promotes plant cell division and elongation, R light plays an important role in shoot elongation (Yang et al., 2017). Previous studies have demonstrated that R light hastens the elongation of the stems of several plant species, such as Phalaenopsis, tobacco, and tomato (Yang et al., 2017; Shin et al., 2008; Izzo et al., 2020). Here, we also observed that S. rubrotinctum tissue-culture-generated plantlets under monochromatic R light were the tallest. R light has also been shown to promote the elongation and extension of tobacco leaves (Izzo et al., 2020), but this phenomenon was not observed in our experiment. In contrast, the leaf length, leaf width and leaf area of the S. rubrotinctum plantlets were lowest under R light, whereas these values were comparatively high for the plants grown under B light and the other light treatments in our study. This discrepancy could be caused by the different plant species and experimental environments used. Related studies have shown that R light promotes polar auxin (indoleacetic acid [IAA]) transport from the apical shoot to basipetal regions and increases the length of the roots of Solanum lycopersicum plantlets (Wu et al., 2014). Similar results were observed in studies of tobacco plantlets; researchers reported that R light promotes lateral root formation and auxin polar transport from the shoots to the roots (Meng et al., 2015). In our study, except for the average root diameter, all of the other relevant parameters of the roots were lowest under monochromatic R light. In addition, the thinnest leaves were observed under R light in the present study, and supplemental B light resulted in thicker leaves. Notably, thicker leaves ordinarily lead to increased absorbance and therefore increased photosynthetic yields (Zheng and Van Labeke, 2017).
Different Light Quality Treatments Affect the Quality of Sedum rubrotinctum Tissue-culture-generated Plantlets
Light absorption is also dependent on the Chl concentration. B light was shown to increase the expressions of different enzymes that regulate the synthesis of Chl, meaning that B light is important for Chl biosynthesis (Wang et al., 2009). Several studies have shown that Chl levels increase in plants under B light (Fan et al., 2013; Izzo et al., 2020; Weremczuk-Jeżyna et al., 2021). However, in our experiment, there was no significant difference in the Chl content between the plantlets under the R treatment and the B treatment, and the treatments involving combinations of R and B light (especially 2R4B and 4R1B) yielded greater Chl levels than did the monochromatic R and B treatments. These findings suggest that a combination of R/B light is more conducive to light absorption and the photosynthetic efficiency of S. rubrotinctum leaves. B light is also generally considered to promote the formation of Chl a, and higher Chl a/b ratios under B light and lower Chl a/b ratios under R light have been observed (Fan et al., 2013). In contrast, in the present study, the R light treatment resulted in the highest Chl a/b ratio.
With respect to the L*, a*, and b* colour values in our study, the L* value was highest under W light, in agreement with the results of Tian et al. (2019), who showed that the L* values (lightness) of Huangjinya leaves under R, R/B and B light treatments clearly decreased compared with those under a W light treatment. With respect to a* and b* values, Tian et al. (2019) reported that, compared to a W light control sample, R light caused obvious decreases in these values. However, the results of our experiment showed that the combination of R/B light clearly resulted in increased a* values, but different light quality treatments had no significant effect on the b* values. Here, a* represents the colour from green to red, and we also observed that the leaves of the S. rubrotinctum plantlets were obviously red under the 3R1B, 2R4B and 4R1B treatments. Therefore, the R/B combination was found to be most effective at promoting the colour development of S. rubrotinctum. Leaf colour formation is complex and determined by the contents, distributions and types of intracellular pigments such as anthocyanins, Chl and carotenoids (Li et al., 2021a). Our results showed that the a* value was closely related to the anthocyanin content (Cant); thus, the R/B combination can promote an increased content of anthocyanins in the leaves of S. rubrotinctum plants, resulting in redder leaves, and this colour change adds to the ornamental value of succulent plants.
PCA is a useful tool that can be used to manage multiparametric data matrices and identify treatments responsible for maximum variation but also to rank factors comprehensively. PCA is widely used for comprehensive evaluations of vegetable, fruit, and food quality levels (Nie et al., 2019; Jin et al., 2022; Lv et al., 2022). In the present study, the results of a comprehensive ranking via PCA based on 25 select raw indicators of S. rubrotinctum tissue-culture-generated plantlets were as follows: 4R1B > 2R4B > 3R1B > W > B > R. Taken together, our results demonstrate that the R/B combination is best by far for promoting S. rubrotinctum growth and leaf colour and that the use of monochromatic light can induce severe changes in photomorphogenesis. These results are consistent with the findings of other authors (Izzo et al., 2020).
Conclusions
Our results indicated that light quality significantly influenced the growth, morphology, and leaf colour of tissue-culture-generated S. rubrotinctum plantlets. R light hastened the elongation of the stems of S. rubrotinctum, whereas B light had a stronger effect on biomass accumulation and the growth of the leaves and roots. Compared to monochromatic light and pure W light, the R/B light combination was significantly better at promoting S. rubrotinctum growth and leaf colour. The 4R1B treatment was most appropriate for promoting growth and inducing the production of attractive colours in S. rubrotinctum tissue-culture-generated plantlets. This study provides a practically significant and optimal solution for growth regulation via light quality for the production of S. rubrotinctum and other succulent plant species. To clarify the exact biological functions and action mechanisms through which light quality affects the growth, morphology, and leaf colour of S. rubrotinctum, more physiological parameters and related genes should be analyzed in the future.