Introduction
Materials and Methods
Fruit Materials
Treatments and Storage Conditions
Fruit Quality Assessment
Determination of the Internal Ethylene Concentration (IEC) and Respiration Rates
Extraction and Determination of Cell Wall Materials
Cell Wall Hydrolase (CWH) Extraction and Activity Determination
Statistical Analysis
Results and Discussion
Fruit Qualities
IEC, Respiration Rates and Weight Loss
Fruit Peel Color Attributes
Uronic Acid Content (UAC) and Total Sugar Content (TSC)
Cell Wall Hydrolase (CWH) Activity
Introduction
Apples are a major fruit crop in Korea, and the demand and production of apples have steadily increased in recent years (KOSIS, 2020). Many new apple cultivars have been developed owing to increased consumption and market demand, including differently sized early- and mid-season apple cultivars (USDA, 2019). Among these, ‘Hwangok’ (‘Kogetsu’ × ‘Yataka Fuji’) is a small-medium size apple cultivar known for its firmness, sweetness, and acidity (Ban et al., 2014).
The ripening of apples is associated with increased ethylene production, which influences the fruits’ biochemical and physiological traits, including the texture, color, acidity, sweetness, and flavor (Lelievre et al., 1997). Changes in fruit texture are a significant indicator of fruit softening, which generally starts from the primary cell wall, and pectin plays a major role in the primary cell wall structure (Payasi et al., 2009; Win et al., 2019, 2021). Pectin typically becomes soluble and depolymerizes during fruit softening, which is induced by the activity of many cell wall hydrolysis enzymes (Brummell and Harpster, 2001; Brummell, 2006). These hydrolytic processes also reduce flesh firmness.
1-Methylcyclopropene (1-MCP), an ethylene perception inhibitor, is commonly used in many countries to improve storability and maintain the quality of apples, as it prevents the production and effects of ethylene during ripening (Fan et al., 1999; Sozzi and Beaudry, 2007; Park, 2012; Lwin and Lee, 2021, 2022; Win et al., 2021). Salicylic acid (SA) is commonly used to control plant diseases and pathogens (Luo et al., 2011); however, a SA treatment can also help maintain flesh firmness, titratable acidity (TA), and weight loss in peaches (Tareen et al., 2012), apples (Kazemi et al., 2011), and bananas (Khademi et al., 2019). In addition, SA can reduce ethylene levels in plants by preventing ethylene from forming enzymes and ACC synthase (Yang and Hoffman, 1984) and by triggering the biosynthesis of spermine, spermidine and putrescine from SAM (Freschi, 2013). Several previous studies also reported that SA reduces ethylene production in tomatoes (Li et al., 1992), pears (Leslie and Romani, 1998), and apples (Kazemi et al., 2011).
Among new Korean apple cultivars, ‘Hwangok’ apples exhibit a particular ripening mechanism with low internal ethylene concentrations (Yoo et al., 2016). In addition, firmness and other important quality characteristics of ‘Hwangok’ apples, including TA and the soluble solid content (SSC), are relatively stable for up to 40 days after harvesting (Yoo et al., 2016). However, the fruit quality of ‘Hwangok’ apples can be reduced after six months in cold storage (Win et al., 2021). Thus far, limited information about ‘Hwangok’ apples and storage optimization strategies is available. For this reason, we examined the effects of SA, 1-MCP, and a combined treatment with both compounds on fruit quality changes of ‘Hwangok’ apples under cold and shelf-life storage conditions. In addition, the degradation of cell wall materials in association with cell wall hydrolase (CWH) activity levels during softening was investigated.
Materials and Methods
Fruit Materials
‘Hwangok’ apples were harvested from a commercial orchard on September 11 in Gunwi, Gyeongsangbuk-do Province, Korea, The harvested fruits were sent to the quality crop management laboratory of Kyungpook National University in Daegu, Korea.
Treatments and Storage Conditions
Fruits free of visual damage and infection were selected and assigned to four treatment groups. These are referred to here as the control, SA, 1-MCP, and SA+1-MCP treatment groups (180 fruits each). The SA treatment was performed by dipping the fruits in a 3 mM SA solution (selected as a result of a previous experiment by the authors) for 5 min and then drying them for 3 h at 20°C. In our previous experiment, we evaluated the concentration rates (1, 2 or 3 mM) of a SA treatment in ‘Hongro’ apples (using the same application time and storage condition), finding that the 3 mM SA solution affected the fruit quality of apples most during storage (unpublished data). Therefore, the 3 mM SA solution was used as the recommended concentration rate in this study. The 1-MCP treatment was executed using fumigation with 1 µL·L-1 1-MCP for 18 h in an enclosed container at 20°C (Yoo et al., 2020). The combined treatment was performed by initially executing the SA treatment followed by the 1-MCP treatment after air-drying for 3 h at 20°C.
The storage conditions were 0 ± 1°C (90 ± 5% relative humidity) for cold storage and 20 ± 1°C (50 ± 5% relative humidity) for shelf-life storage. The fruits were stored for up to 180 and 180 + 7 days, and the fruit quality was assessed at 30 and 30 + 7 day intervals, respectively. Fifteen fruits per treatment for each interval were used for all assessments.
Fruit Quality Assessment
Flesh firmness (11 mm-diameter probe) was determined at three places in the equator region of each fruit using a firmness tester (Compac-100Ⅱ, Sun Scientific Co., Tokyo, Japan). The SSC was detected using a refractometer (PR-201α, Atago Co., Ltd., Tokyo, Japan). TA was determined through the titration of juice samples with NaOH to an endpoint of pH 8.1, according to Win et al. (2019). Fruit color indicators were determined on fruit peels using a chroma meter (CR-400, Konica Minolta Inc., Tokyo, Japan). Weight loss was determined based on the loss of water content of individual fruits before and after storage (Kim et al., 2018). During the experiment, flesh tissue was sampled, frozen, and stored at –80°C for the measurements of uronic acid (UAC), the total sugar content (TSC), and the CWH activity. Cell wall metabolism under each storage condition was measured at every 60 and 60 + 7 days intervals.
Determination of the Internal Ethylene Concentration (IEC) and Respiration Rates
For IEC, a 1-mL sample of gas was taken from the core cavity of each fruit and was injected into a gas chromatograph (GC 7820A, Agilent Technologies Inc., Santa Clara, CA, USA) (Yoo et al., 2021). During the IEC measurements, the oven temperature was set to 90°C, with 250°C and 100°C as the injector and detector temperatures, respectively. Regarding the respiration rates, the fruits were sealed in 1.6-L containers for 1 h and the gas (1 mL) was sampled using a syringe and was injected into a gas chromatograph (GC-2010; Shimadzu, Kyoto, Japan). During the measurements, the oven temperature was 90°C, with 100°C as both the injector and detector temperature. Internal standards are used for the calibration of the IEC and respiration rates.
Extraction and Determination of Cell Wall Materials
The cell wall composition, including UAC and TSC, was determined after extraction as described by Yoo et al. (2020). In this case, an amount of 10 g of frozen flesh samples was heated in 100 mL of boiling ethanol (95%) for 40 min and was homogenized. The homogenates were filtered through filter paper, and the residues were washed with ethanol (95%) and acetone (95%). The residues were dried for 24 h at 30°C and were used as alcohol-insoluble substances (AIS) to investigate the amounts of cell wall materials. Dried AIS powder (10 mg) was slowly extracted using sulfuric acid (2 mL) for 15 min. The extracted samples were diluted with distilled water (15 mL) and were filtered using filter paper. The final volume was adjusted to 50 mL with distilled water for the determination of UAC and TSC.
To determine UAC, a mixture of AIS (0.5 mL) and sulfuric acid (3 mL) was boiled for 20 min and was then cooled. After an addition of 0.1% carbazole (0.1 mL), the solution was allowed to react for 2 h at 30°C. The absorbance was measured at 490 nm using a UV-spectrophotometer with a blank. The amount of UAC was calculated using a D-galacturonic acid standard curve and was expressed as g per kg AIS.
To determine TSC, a mixture of AIS (0.5 mL) and 5% phenol (0.5 mL) was allowed to react with sulfuric acid (2.5 mL) and was incubated for 30 min at 25°C. The absorbance was measured at 490 nm using a UV-spectrophotometer with a blank. The amount of TSC was calculated using a glucose standard curve and was expressed as g per kg AIS.
Cell Wall Hydrolase (CWH) Extraction and Activity Determination
CWH was extracted and the activity was determined according to Yoo et al. (2020). All extraction steps were performed at 4°C. Frozen flesh samples (50 g) were homogenized with a 10 mM sodium phosphate buffer (100 mL, pH 7.0) containing polyvinylpolypyrrolidone (0.1 g). After an addition of NaCl (8.766 g), the homogenates were stirred for 3 h and were centrifuged (12,000 × g) for 1 h. The supernatants were collected, stirred for 12 h after adding ammonium sulfate, and were centrifuged (12,000 × g) for 1 h. The pellets were collected and dialyzed in a 10 mM sodium phosphate buffer for two days, after which the extract solutions were centrifuged (20,000 × g) for 1 h and the final sample extracts were collected.
The activities of the following six CWH enzymes were determined: β-galactosidase (β-gal), α-galactosidase (α-gal), β-glucosidase (β-glu), α-mannosidase (α-man), α-arabinosidase (α-ara), and β-xylosidase (β-xyl). A mixture of sample extract (250 µL) and a 10 mM sodium acetate buffer (200 µL, pH 4.0) was incubated at 30°C in a water bath for 1 h. After adding 2% p-nitrophenyl-pyranoside (125 µL) and 1 M sodium carbonate (1 mL) to the mixture, the absorbance was measured at 410 nm using a UV-spectrophotometer with a blank. The CWH activities were calculated using p-nitrophenyl standard curves and were expressed as units per kg fresh weight. One unit represents the amount of enzyme released from 1 mmol p-nitrophenol at 30°C for 1 h.
Statistical Analysis
Statistical analysis software (SPSS, IBM SPSS Statistics 26, IBM Co., Armonk, NY, USA) was used for all analyses. The data were subjected to an analysis of variance, and the means were compared among the treatments using Duncan’s multiple range test (p < 0.05).
Results and Discussion
Fruit Qualities
Fruit flesh firmness decreased in all treatments under both storage conditions. After 180 days of cold storage, the flesh firmness of the control and SA-treated fruits decreased from 71.7 N at harvest to 59.9 and 58.5 N; in the 1-MCP- and SA+1-MCP-treated fruits, firmness was maintained at 66.8 and 64.9 N, respectively (Fig. 1A). Similarly, during the cold storage plus shelf life condition, the 1-MCP and SA+1-MCP apples maintained flesh firmness at higher levels than the controls (Fig. 1B). However, the SA treatment alone did not help to maintain flesh firmness under either storage condition (Fig. 1A and 1B). Similarly, TA also decreased after all treatments under both storage conditions (Fig. 1C and 1D). Similar to the flesh firmness outcomes, the 1-MCP and SA+1-MCP apples maintained TA at higher levels compared to the control fruits and the specimens that underwent the SA treatments. SSC increased during early storage and then decreased during later storage. However, the overall SSC results did not differ significantly among the treatments under each storage condition (Fig. 1E and 1F).
Flesh firmness, SSC, and TA are important characteristics that are directly related to consumer preference. Flesh firmness is a standard indicator of the freshness and textural quality of fruits (Musacchi and Serra, 2018). 1-MCP can help to maintain fruit texture, as reported previously (Dal Cin et al., 2006; Zhang et al., 2020). SA is a natural phenolic compound that can reduce ethylene production and help maintain fruit quality (Kazemi et al., 2011; Khademi and Ershadi, 2013; Khedr, 2016). However, in the current study, firmness was not maintained in SA-treated apples under either storage condition, possibly due to the low SA concentration or to cultivar-specific differences. In addition, the firmness results after all treatments did not differ markedly between the two storage conditions, possibly due to the slow ripening of ‘Hwangok’ cultivar apples.
The 1-MCP and SA+1-MCP-treated fruits showed a delayed reduction of TA under both storage conditions. Compared to cold storage, TA was slightly lower when the fruits were transferred to shelf-life storage, especially in the controls and SA-treated fruits. Therefore, TA may be further reduced in untreated fruits when they are transferred from cold to shelf-life storage. 1-MCP can help retain flesh firmness and TA in ‘Green Ball’ and ‘Summer King’ apples during cold storage (Win et al., 2019). Similarly, 1-MCP can help maintain the TA content at different storage temperatures (Mir et al., 2001). In addition, a SA treatment can help maintain the TA content in mangos (Khedr, 2016). However, in the current study, the SA treatment did not affect TA, though this may be related to the use of a lower concentration of SA than those in previous studies or to different cultivars or storage conditions.
SSC was not affected by any of the treatments in either storage condition (Fig. 1). No difference in SSC has been observed between 1-MCP-treated and control fruits under cold and shelf-life storage (Pre-Aymard et al., 2005). Moreover, no effect of SSC in 1-MCP-treated ‘Ginger Gold’, ‘Gala’, and ‘Jonagold’ apples was previously observed during shelf-life storage (Fan et al., 1999). Although 1 and 2 mM SA treatments can reduce SSC in peaches, SA at 4 mM does not affect peach SSC levels during storage (Khademi and Ershadi, 2013). However, SSC is reduced through 3 mM SA in ‘Jonagold’ apples (Kazemi et al., 2011). Therefore, the effects of the SA and 1-MCP treatment on SSC may vary depending on the cultivar or treatment concentration.
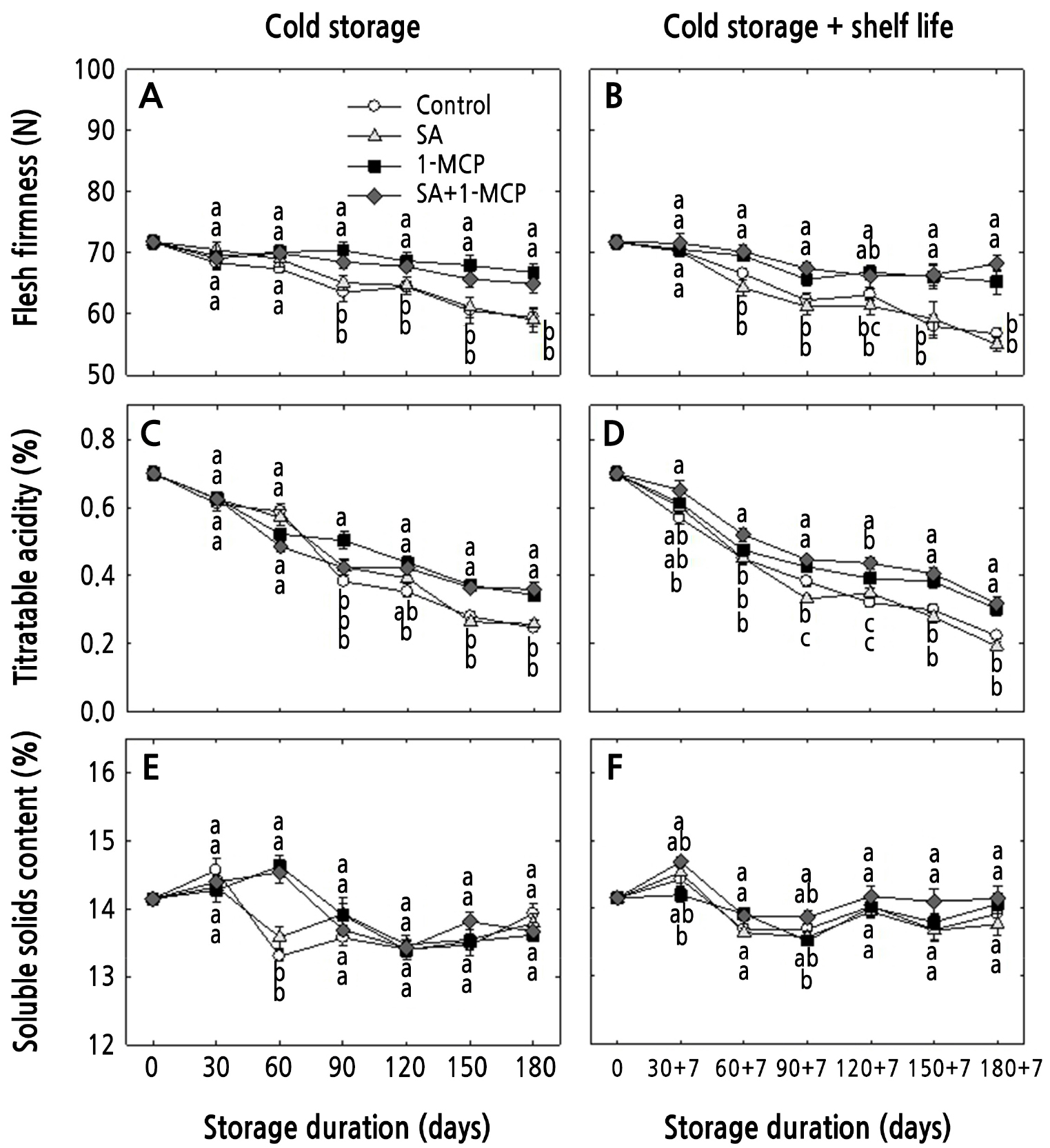
Fig. 1.
Changes in flesh firmness, titratable acidity, and soluble solids content of ‘Hwangok’ apples treated with SA, 1-MCP, and SA+1-MCP and stored in cold storage (0°C) for 180 days or in cold storage (0°C) plus shelf life (20°C) for 180 + 7 days. Different lower-case letters for each storage day indicate a significant difference at p < 0.05. Shown are the means ± standard error (n = 15).
IEC, Respiration Rates and Weight Loss
In the controls and SA-treated fruits, IEC increased to 29.8 and 26.8 µL·L-1 under cold storage; the levels were 90.0 and 86.0 µL·L-1 under the cold storage plus shelf-life storage condition, respectively (Fig. 2A and 2B). However, compared to the controls, lower IECs were observed in SA-treated fruits until 150 + 7 days of shelf life (Fig. 2B). The 1-MCP and SA+1-MCP treatment reduced IECs in apples under both storage conditions (Fig. 2A and 2B). In addition, respiration rates were higher in the controls and the SA-treated fruits and lower in the 1-MCP- and SA+1-MCP-treated fruits under both storage conditions (Fig. 2C and 2D). Compared to the controls, a lower respiration rate was observed in SA-treated fruits from 90 + 7 to 180 +7 days of shelf life (Fig. 2D). The higher IEC observed under cold storage plus shelf life may be due to the transfer of the fruits to the shelf life conditions (Saletnik et al., 2022), as observed in the current study. Park et al. (2016) observed that 1-MCP reduced the IEC of ‘Jonathan’ apples but did not affect the respiration rates. However, Argenta et al. (2007) observed that 1-MCP reduced both the IEC and respiration rates in ‘Delicious’ apples. In addition, reduced respiration rates and ethylene levels by 1-MCP have been reported in many other fruits (Zhang et al., 2020), in line with the results of the current study. Khedr (2016) found a slight inhibition of ethylene production in SA-treated bananas. Similarly, in the current study, slightly lower IECs and respiration rates were observed in SA-treated apples, especially when they were transferred to shelf-life storage; however, this was not sufficient for maintaining fruit quality consistently.
Weight loss progressively increased under both storage conditions, especially in cold storage plus shelf storage (Fig. 2E and 2F). Under cold storage, the weight losses of the controls and SA-treated apples were 3.5% and 3.4%, respectively, whereas, it was only 3.0% in 1-MCP-treated fruits after 180 days of cold storage. In addition, weight loss was reduced in 1-MCP-treated fruits from 90 to 180 days, especially compared to those of the controls and the SA-treated fruits (Fig. 2E). Under cold storage plus shelf storage, the weight loss in all treatments was higher than that in all cold storage treatments (Fig. 2F). In addition, the weight loss was reduced in 1-MCP-treated fruits from 60 + 7 days to 150 + 7 days, especially compared to the corresponding outcomes of the controls and SA-treated fruits. However, the weight loss did not differ among the treatments at 180 + 7 days (Fig. 2F). Increased weight loss is associated with fruit transpiration (Ghafir et al., 2009; Hung et al., 2011; Konarska, 2013). In the current study, the weight loss was higher during cold storage plus shelf life, which may be due to the higher transpiration rates when the apples were removed to shelf-life storage. 1-MCP and SA treatments delay fruit weight loss in plums (Martinez-Romero et al., 2003) and strawberries (Babalar et al., 2007); however, the fruit weight loss induced by 1-MCP may differ among different cultivars (Win et al., 2019). In the current study, the 1-MCP treatment reduced weight loss, whereas the SA treatment did not have any effect on fruit weight loss outcomes.
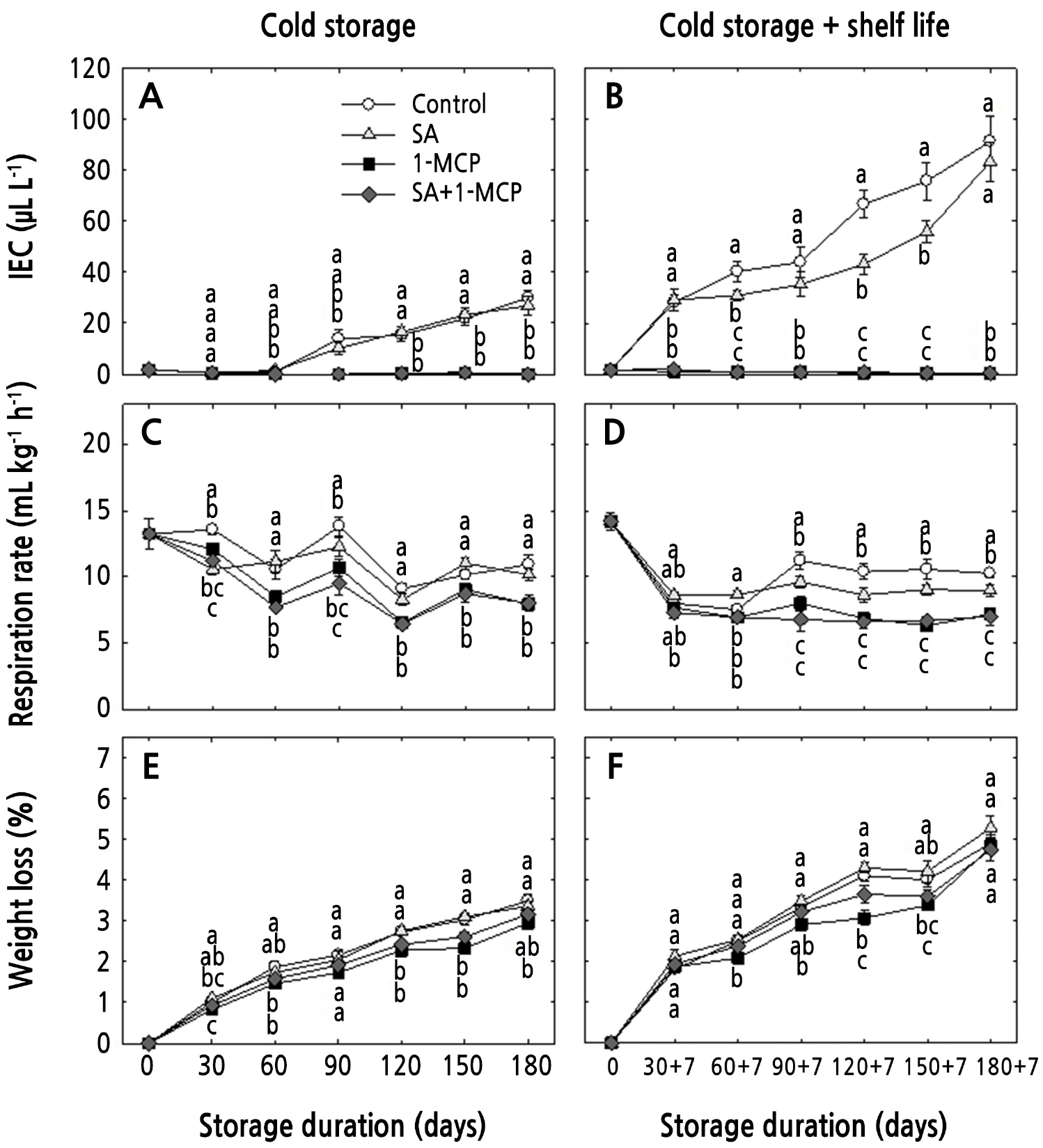
Fig. 2.
Changes in the internal ethylene concentration (IEC), respiration rate, and weight loss of ‘Hwangok’ apples with SA, 1-MCP, and SA+1-MCP and stored in cold storage (0°C) for 180 days or in cold storage (0°C) plus shelf life (20°C) for 180 + 7 days. Different lower-case letters for each storage day indicate a significant difference at p < 0.05. Shown are the means ± standard error (n = 15).
Fruit Peel Color Attributes
The fruit peel color index L* of ‘Hwangok’ apples did not differ statistically among the treatments in both storage conditions (Fig. 3A and 3B). The a* and b* values increased during storage, with this change delayed by 1-MCP and SA+1-MCP (Fig. 3C–3F). Changes in apple peel color generally occur due to the accumulation of carotenoid and anthocyanin compounds and the degradation of chlorophyll compounds in the ripening peel (Butkeviciute et al., 2021). However, these processes may be delayed by 1-MCP and SA+1-MCP treatments according to the present results. A similar result was observed in 1-MCP-treated fruits (Zucoloto et al., 2017; Yoo et al., 2019a, 2019b). However, SA did not delay pigment changes during ripening.
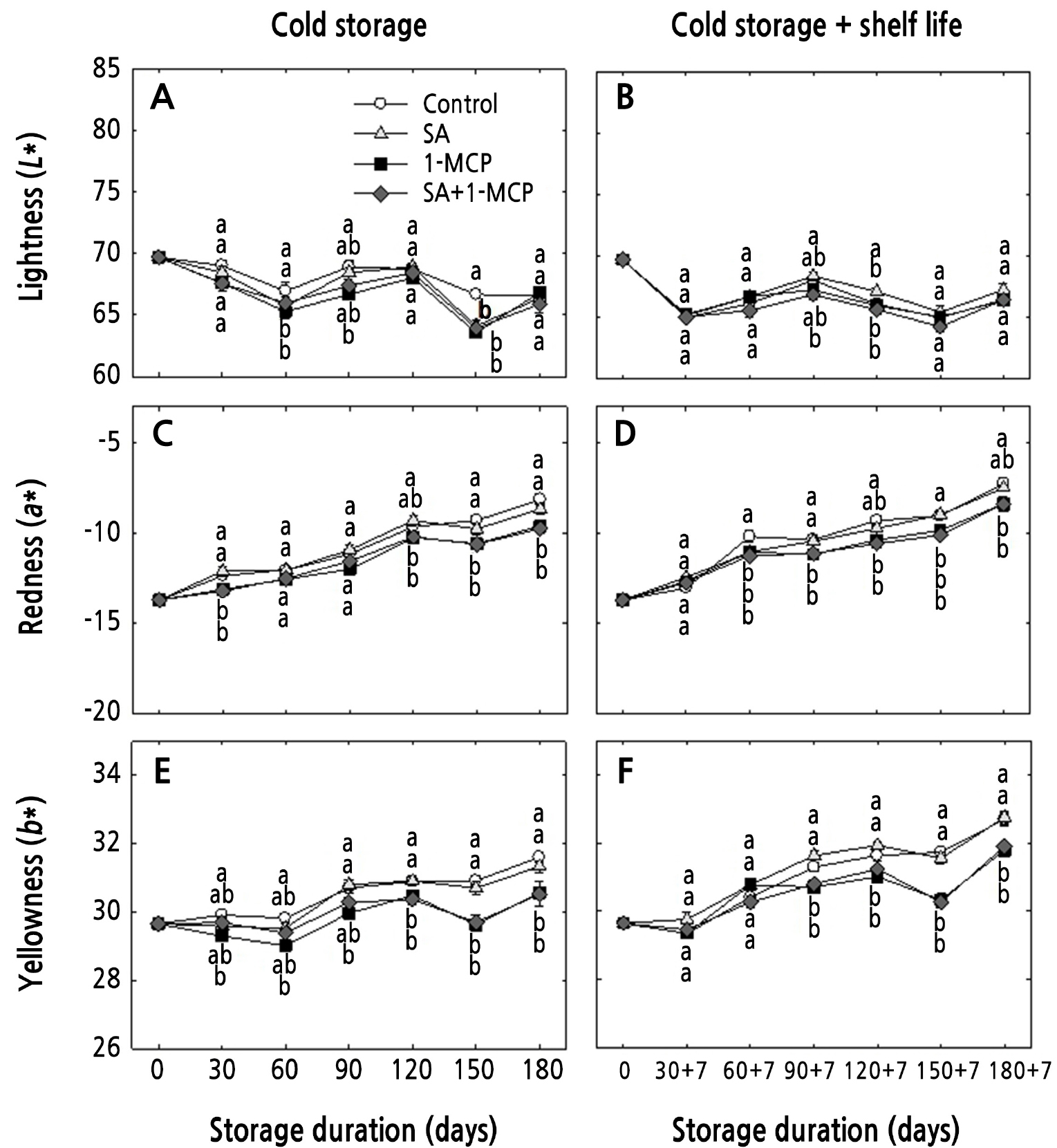
Fig. 3.
Changes in peel color (L*, a*, and b*) measured on the equatorial region of ‘Hwangok’ apples with SA, 1-MCP, and SA+1-MCP and stored in cold storage (0°C) for 180 days or in cold storage (0°C) plus shelf life (20°C) for 180 + 7 days. Different lower-case letters for each storage day indicate a significant difference at p < 0.05. Shown are the means ± standard error (n = 15).
Uronic Acid Content (UAC) and Total Sugar Content (TSC)
UAC decreased during cold storage, especially in the controls and SA-treated fruits (Fig. 4A and 4B). The UAC of 1-MCP- and SA+1-MCP-treated apples was higher than the control until 120 days, but it did not differ among the treatments after 180 days of cold storage (Fig. 4A). However, under cold storage plus shelf life, the UAC of SA+1-MCP-treated apples was higher than those of the controls throughout the storage period (Fig. 4B). TSC decreased during all treatments under both storage conditions (Fig. 4C and 4D). However, 1-MCP and SA+1-MCP decelerated total sugar solubilization under both storage conditions. The TSC outcomes of the control and SA-treated fruits gradually decreased to 126.0 and 131.5 mg g-1 AIS after 180 days in cold storage and to 116.5 and 121.8 mg g-1 AIS after 180 + 7 days in cold plus shelf-life storage, respectively (Fig. 4C and 4D).
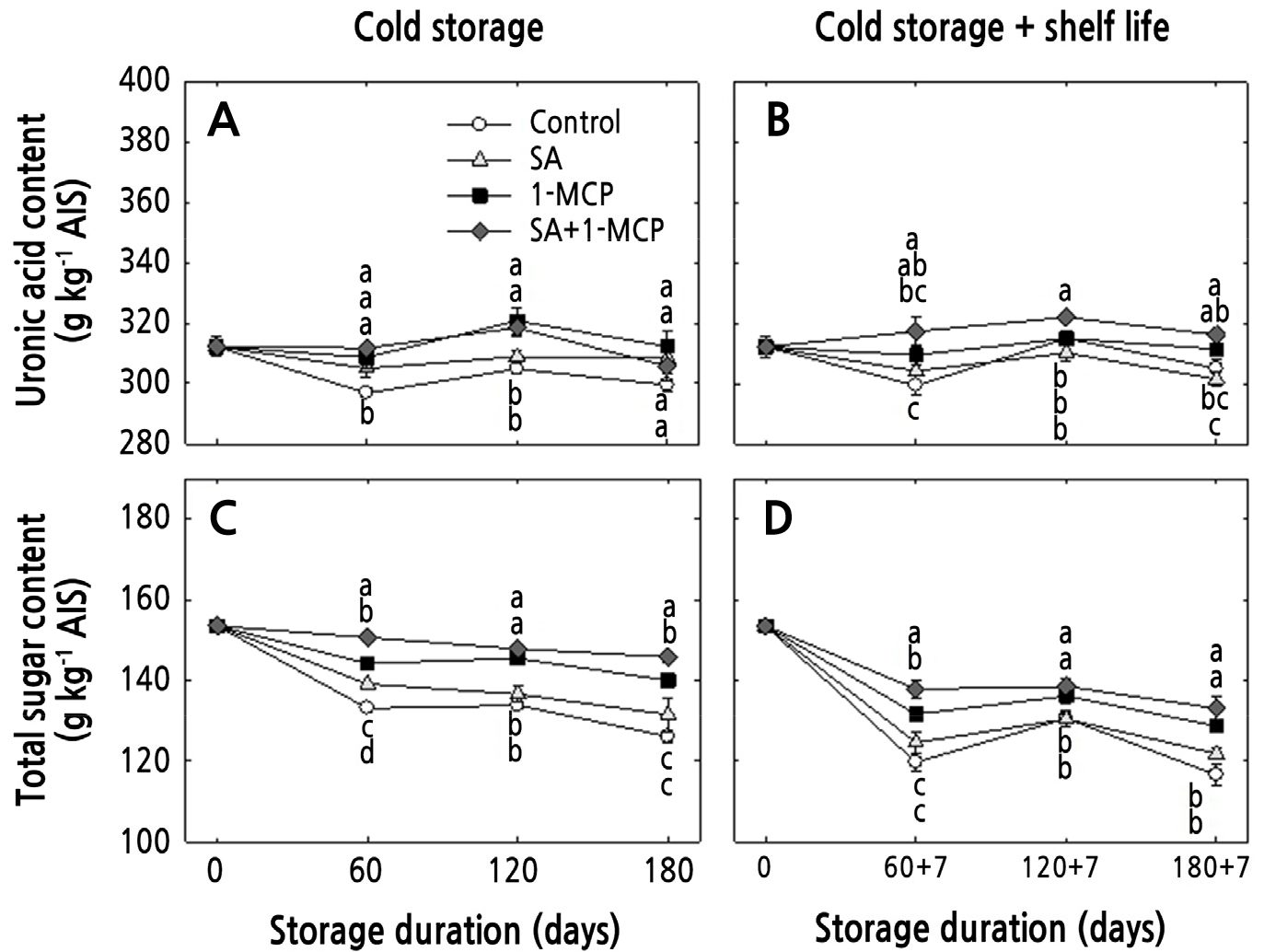
Fig. 4.
Changes in uronic acid levels and the total sugar content of ‘Hwangok’ apples with SA, 1-MCP, and SA+1-MCP and stored in cold storage (0°C) for 180 days or in cold storage (0°C) plus shelf life (20°C) for 180 + 7 days. Different lower-case letters for each storage day indicate a significant difference at p < 0.05. Shown are the means ± standard error (n = 3).
The cell wall material is important for maintaining fruit flesh firmness. Fruit cell walls are primarily composed of polyuronides and soluble sugars, which are solubilized during softening, thus reducing the firmness (Brummell and Harpster, 2001). Therefore, the degradation of UAC and TSC is an important indicator of cell wall solubilization, which is mediated by CWH activity levels (Yoo et al., 2020, 2021; Kwon et al., 2022). In the current study, 1-MCP and SA+1-MCP showed consistent UAC outcomes in ‘Hwangok’ apples for up to 120 days in cold storage and for up to 180 days in cold plus shelf-life storage. In addition, 1-MCP and SA+1-MCP helped to maintain TSC levels under both storage conditions. Therefore, 1-MCP helped to maintain the cell wall materials, in line with the results of previous studies involving peaches and apples (Yoo et al., 2019b, 2020, 2021). However, the SA treatment did not prevent the solubilization of the cell wall materials in the current study, likely due to the use of a lower concentration, as previously reported in a study of mandarin fruit (Baswal et al., 2020).
Cell Wall Hydrolase (CWH) Activity
The activities of all CWHs, except β-xyl, gradually increased in the controls and SA-treated fruits under both storage conditions. However, the increase in the CWH activity was delayed by the 1-MCP and SA+1-MCP treatments under both storage conditions. The activities of CWHs, including β-gal, β-glu, and α-man, were highest in the controls after 180 days of cold storage, and those of β-gal, β-glu, and α-ara were highest after 180 + 7 days of cold storage and shelf-life storage (Fig. 5). In the cell wall, β-gal and α-ara are associated with the polymerization of pectin side chains, which are importantly related to fruit-texture-related cell wall structures (Brummell and Harpster, 2001; Payasi et al., 2009). Brummell (2006) also reported that β-gal and α-ara activities increase when the cell wall loses galactosyl and arabinosyl residues, markedly contributing to reduced firmness levels. In addition, β-glu and β-xyl are responsible for the degradation of cellulose and hemicellulose networks in the cell wall (Guolao and Oliveira, 2008). Moreover, α-man and α-gal are associated with a group of glycosidases that is also responsible for producing cell wall oligosaccharides (Vicente et al., 2007). Yoo et al. (2019a, 2019b and 2021) observed that the CWH activities in peaches, persimmons and apples were decelerated by 1-MCP. However, Baswal et al. (2020) found that the effects of SA on cell wall enzymes depended on the concentration. Therefore, the lack of effects on CWH by SA in the current study may be due to the cultivar tested or the SA concentration used.
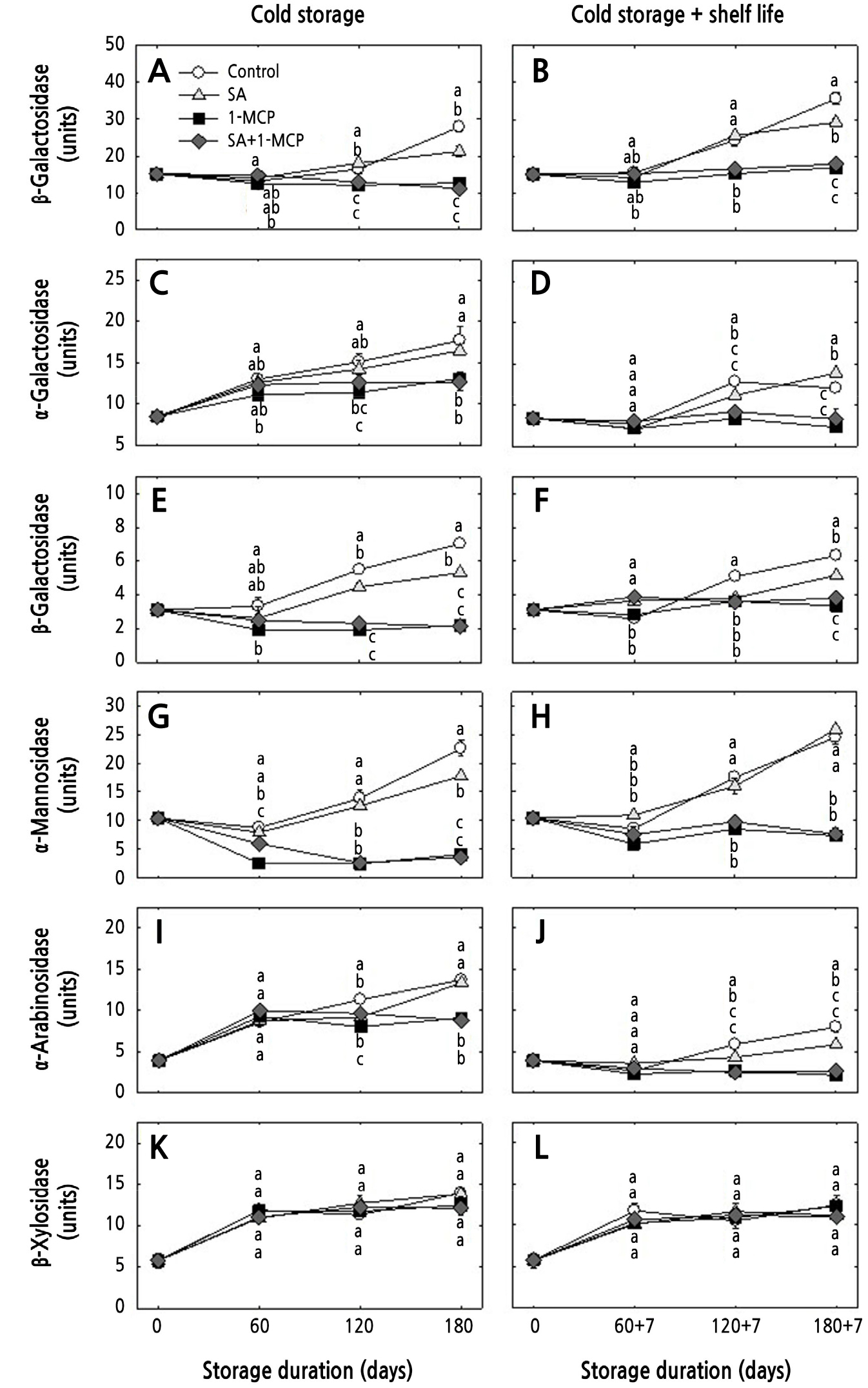
Fig. 5.
Changes in cell wall hydrolases activities (β-galactosidase, α-galactosidase, β-glucosidase, β-arabinosidase, β-xylosidase, and α-mannosidase) of ‘Hwangok’ apples with SA, 1-MCP, and SA+1-MCP and when stored in cold storage (0°C) for 180 days or in cold storage (0°C) plus shelf life (20°C) for 180 + 7 days. Different lower-case letters for each storage day indicate a significant difference at p < 0.05. Shown are the means ± standard error (n = 3). One unit represents the amount of enzyme released from 1 mmol of p-nitrophenol per kg (fresh weight) at 30°C for 1 h.
In conclusion, the 1-MCP treatment alone helped to maintain the fruit quality of ‘Hwangok’ apples through reduced IEC and respiration levels, which in turn delayed fruit softening. In contrast, the SA treatment alone did not affect the fruit quality of ‘Hwangok’ apples under either storage condition. The combined SA+1-MCP treatment affected fruit quality outcomes, similar to the effects of the 1-MCP treatment. Therefore, the findings here suggest that 1-MCP is effective for maintaining the fruit quality of ‘Hwangok’ apples under cold and shelf-life storage conditions. Further studies are necessary to understand the effects of SA on apple quality outcomes.