Introduction
Materials and Methods
Growing Conditions
Fish and Plants
Treatment
Water Quality Measurements
Measurement of Plant Growth Parameters
Statistical Analysis
Results and Discussion
EC and pH in Water
Nutrients in Water
Plant Growth Parameters
Conclusion
Introduction
Aquaponics is an emerging agricultural cultivation system that creates a symbiotic ecosystem for plants, fish, and microbes in a closed system (Yang and Kim, 2019). It is well known to be more efficient for water and nutrient utilization compared to conventional systems (Barbosa et al., 2015), but the challenge is management issues to maintain the water quality for both the fish and the plants (Badiola et al., 2012). Plants require a number of macro- and micro-nutrients to grow. Fortunately, in aquaponics systems, most of these nutrients are added to the water from the fish feed and byproducts produced by the fish component, leading to the provision of proper nutrients for plant growth (Yildiz et al., 2017). While the optimal growth of each organism requires a different water condition, it is suggested that the water quality parameters are a necessary compromise to promote nitrification to ensure the health of the fish. As well as, pH and EC in aquapinic water should be maintained based on types of plant and fish in this system (Tyson et al., 2007). Notably, the pH and strength of the EC of the solution act as key factors for a successful aquaponics system, influencing the physiology and yield of the plants (Wortman, 2015; Delaide et al., 2016; Jeong et al., 2020). A higher pH value (more than 7) inhibits plants from up taking essential nutrients, such as iron, from the solution (Rakocy, 2012).
Currently, aquaponic systems are also finding use as an interior design component, serving as both an aquarium and a ‘planterior’ item. Planterior, a popular pattern in interior design, refers to decorating the inside of a building with plants. Naturalistic and aesthetic indoor spaces are created using various functional living plants, and these promote healthy healing for residents and visitors. Water quality of aquaponic system in indoor spaces is important for plant growth, also making plant crops productive and profitable (Yang and Kim, 2019). It is crucial to produce healthy high-quality plants in aquaponics. Actively growing plants can act as a biological filter and improve water quality levels by removing nutrients from aquaponic water, which in turn contributes to maintaining good fish health (Yildiz et al., 2017).
The substrate for planting media is an important factor to maintain water quality levels in an aquaponics system. Different types of organic and inorganic substrates are used in this system. Inorganic substrates, such as orchid stone, synthetic sponge, hydroball, and volcanic rock have many advantages, including lower water holding capacities, better aeration for roots, and improved drainage and filtering in the aquaponic system (Lee et al., 2020). In an aquaponics system used for planterior purposes, inorganic substrates are also preferable, as such substrates are affordable, easy to find, lightweight, easy to maintain, and create a decorative appearance (Wu et al., 2022). Furthermore, inorganic substrates add ions and minerals to aquaponics water and stimulate plant growth (Rafiee and Saad, 2006). Limited research on different types of substrates has shown that inorganic substrates are preferable in aquaponics systems (Taofik et al., 2019).
Kalanchoe blossfeldiana, belonging to the Crassulaceae family, is a succulent perennial. It is dark green, with scallop-edged leaves, and its flower clusters are large umbels that are held above the foliage (Garcia-Sogo et al., 2010). It has been among the most commonly grown flowering pot plants in Europe in terms of total number of annual production (Castelblanque et al., 2010). It is also acts as a medicinal plant (Kolodziejczyk-Czepas and Stochmal, 2017). It is a CAM plant that characteristically closes its stomata during part of the day, and then at night, opens them, as contrasted with C3 and C4 plants (Gotoh et al., 2018). K. blossfeldiana is a very popular planterior plant owing to its attractive flowering and minimal need for care. Considerable interest in this ornamental plant has led to the development of its use in aquaponics systems for interior purposes.
Aquaponic systems are developed with different plant and fish combinations for interior purposes. Although the findings of different studies have been integrated into aquaponics systems (Asciuto et al., 2019; Greenfeld et al., 2021), information about inorganic substrates with regard to plant growth and nutrient concentrations in the water of these systems is still lacking. Therefore, the objective of this study was to investigate the effects of different inorganic planting media on the growth parameters of K. blossfeldiana and the nutrient concentration in water where goldfish are kept.
Materials and Methods
Growing Conditions
This experiment was conducted in an environmentally controlled room located at Kangwon National University, Chuncheon, South Korea (latitude, 38°06' N; longitude, 128°17' E) from May 23, 2022 to July 26, 2022. A recycling deep flow system and suitably sized tank (46 × 32 × 24 cm; KSP−1000, Gahwa tech, Korea) for home interior spaces were used. The tank was divided into two sections: an upper section and a lower section (Fig. 1). The upper section was for growing plant and the lower section was for keeping fish. The total amount of water used in the system is 15 L, and the water is circulated from the lower section to the upper section of the tank by an underwater motor. Furthermore, the water is drained from the upper section of the tank to the lower section. For filtration, 800 mL of ceramic rings (Siporax, Sera Co., Ltd. USA) and filtering cotton (20 × 15 cm) were placed in the upper section of the tank. These materials help to ensure clean and colorless water in the lower section of the tank compared to that in the upper section.
The temperature of the room was maintained at 23°C ± 3 and the relative humidity was 60% ± 10. White LEDs (light-emitting diodes) were used, and the intensity of light for the plants was set to 100 µmol·m2·s-1. From the beginning of the experiment to June 15, 2022 the light/dark cycle was 14:10. Light was turned on for 15 min, and then turned off for 15 min, repeatedly. From June 16th to the end of the last day, the light/dark cycle was 15:9, with the light turned on for 30 min and then turned off for 15 min, repeatedly. We set this experiment to that developers may use the results here in the future for planterior purposes when designing indoor systems. Moreover, we attempt to provide a long photoperiod for plants with low light irradiance. In addition, to avoid light stress on plants by continuous lighting, we use a number of short breaks during the light period.
Fish and Plants
Five goldfish (Carassius auratus auratus, (51.5 ± 3) g per fish) were used in every treatment. Every day, 0.25 g fish feed (Grovita, Korea Feed Co., Korea) was supplied into each tank. The feed ingredients were crude protein, crude flour, crude fiber, crude fat, phosphorus, and calcium (36.0, 13.0, 5.0, 4.0, 1.80, and 0.40%, respectively). Before starting the experiment, the fish were grown in tanks for eight weeks to prepare the aquaponics water (pH and EC) in which to grow the plants. Then, Kalanchoe blossfeldiana ‘Peperu’ seedlings were transplanted into the tank on May 23, 2022 to start growing. Six plants were planted in every tank. Each seedling had approximately seven leaves with a height of 17.5 cm and stem diameter of 6 cm.
Treatment
Four different inorganic substrates, sponge type (30 PPI; Filson filter, Henan, China), mixed orchid stone 0.3 and 1.5 cm in size at a 1:3 ratio (Hyugato, Hugato, Japan), hydroball (0.5 cm; Better loess ball, Potted World, Korea), and volcanic rock (1.5 cm; Safflower rock, Bando Sangsa, Korea), were used as the growing substrates in the upper section of the tank. These are indicated as SS, SO, SH, and SV, respectively. During the treatment period, the aquaponic water of the tank was slightly reduced due to evapotranspiration. For this reason, at regular intervals of seven days, the tank water was adjusted to 15 L by adding fresh water at the required amount. Notably, after 22 days of treatment, an obstacle formed in the water circulation system of the SV treatment, and 5 L aquaponic water overflowed from the tank. At that time, for the SV treatment, we added a further 5 L of fresh water into the tank and continued our experiment.
Water Quality Measurements
The pH and EC of aquaponic water were checked daily using a multipurpose water quality meter (HI9813-6, Hann Instruments Inc., Romania). The pH level in the water, when decreased to 5.0 or less due to the nitrification process and nutrient absorption by plants, was balanced by adding 5 mL 1 N KOH. NO3‒ and Ca2+ were analyzed at five-day intervals with a portable reflectometer (RQflex 20, Merck Co., Germany). The NH4+, HPO42‒, and Mg2+ levels were analyzed at five-day intervals using an ion detector (Multi-ion probe, CleanGrow, UK).
Measurement of Plant Growth Parameters
At 25 and 50 days after treatment, we measured the plant growth parameters. The plant height, width, leaf length, and leaf width were measured using a ruler. The plant width is the distance between the tip of the largest leaf of the plant and the tip of its opposite largest leaf. The number of leaves was counted manually. We measured the SPAD value from the upper leaves of the plant (three fully opened, healthy leaves were selected from a plant to formulate an average value, and each leaf obtained was the average of three readings from the upper side of the leaf blade) with a chlorophyll meter (SPAD 502, Minolta, Japan). The stem diameter was measured at a distance of 1 cm from the base of the plant using a digital caliper (CD-20APX; Mitutoyo Corp., Kanagawa, Japan). The numbers of nodes, branches, and flowers of plants were counted.
Statistical Analysis
The experiment was conducted using three replications per treatment. The effects of the treatments were analyzed using IBM SPSS Statistics for Windows, version 26 (IBM Corp., Armonk, NY, USA). Significant differences among the means were examined by means of an analysis of variance (ANOVA), followed by Duncan’s multiple range test (DMRT) at p < 0.05.
Results and Discussion
EC and pH in Water
The electrical conductivity (EC) of the water in all treatments showed an increasing trend from the beginning of treatment to the days after. The highest EC in water was always found in the SH treatment, and the range was 0.58 to 0.85 (Fig. 2A). At 22 days after commencing the treatments, the lowest EC was observed in the SV treatment compared to the others. Newly added fresh water of 5 L at 22 days of treatment was the cause of the reduced EC in the SV treatment compared to the other outcomes. However, before this, the EC in the SV treatment was always higher than those in the SS and SO treatments. Furthermore, the range of EC of the water in the SO treatment was 0.45 − 0.65, and that in the SS treatment was 0.50 − 0.76. In this study, we found that the EC in all treatments was less than 1 dS·m-1, an outcome supported by other research findings. Wortman (2015) noted that plants in aquaponic systems prefer an EC of less than 1 dS·m-1, as they are fertilized by the aquaculture effluent, which becomes active at this level of EC. However, Noh et al. (2011) found that an EC of 1.5 is better than an EC of 2.0 and 3.0 for kalanchoe plants in a hydroponic system, but this range is not preferable for aquaponic fish.
The EC value increased in all treatment solutions (Fig. 2A) due to the input fish feed and the increasing nutrient accumulation in the water as the treatment period grew longer. Delaide et al. (2017) also found an increasing EC level with the treatment period in water of an aquaponics system involving lettuce and tilapia. They reported faster calcium and nitrate accumulation rates compared to those of other nutrients, which affected the EC of the water. On the initial day of the treatment, the EC levels were not the same in the SS, SO, SH, and SV treatments, but these differences were small (Fig. 2A). This arose because before we transplanted the plants at around eight weeks, the fish were kept and fed to prepare the water for growing the plants, resulting in the EC not being identical in the different tanks. Buzby and Lin (2014) reported that after transplanting plants in an aquaponic system, a nutrient cycle was created by the fish output and plant uptake. Yang and Kim (2020) also noted that the EC of water in an aquaponic system is slowly affected by the released nutrients from fish output at the initial stage, but this led to an increase in the EC level over time in the aquaponic system. Other studies found that nutrient concentrations in aquaponics water also influenced the EC levels (Nicoletto et al., 2018; Yang and Kim, 2019). In addition, the physical properties of the substrate also factor into the EC levels in aquaponics water (Yep et al., 2020).
The pH of the nutrient solution plays an important role in fish health due to the oxidation of NH4 and NO2 in the aquaponic system (Chen and Ma, 2006). Differences in the solution pH affect plant nutrient uptake outcomes (Wortman, 2015). During the treatment period here, the pH of the water fluctuated in all treatments in the range of 6.9 to 4.0 (Fig. 2B). However, the fluctuation range differed depending on the treatment. Greater fluctuation of the pH was observed in the SS treatment. In contrast, SO and SH showed less fluctuation of the pH (6.8-4.5). Schreier et al. (2010) reported that in an aquaponic system, fish feed and excreta by fish add ammonia to the solution, which is then converted into nitrates by nitrifying bacteria, causing the release of H+. This phenomenon is assumed to be the main reason for low pH occurrence in water, and causing the water to acidify during the treatment (Fig. 2B). Notably, when the pH of the water was decreased to less than pH 5.0, we added 1 N of KOH to balance the pH. Tyson et al. (2007) and Delaide et al. (2016) noted that pH values in an aquaponic system are managed at 6.0 − 7.0 to ensure sufficient rates of nitrification and so as not to hamper plant uptake of essential nutrients from the water. In another study, the pH level was maintained in the range of 4.0 to 7.1 for an aquaponic system (Lee et al., 2022). Hence, we attempted to maintain the lowest range of pH (approx. 5.0) in this study.
Nutrients in Water
Plants take up their essential nutrients in an ionic form. Usually, the nutrients are present in the soil in solid or other compound forms. However, in aquaponics systems, water nutrients from fish feed and fish waste are added, and these are solubilized and converted into an ion form by the activities of different microorganisms (Rakocy et al., 2004). Plants easily acquire the ionic form of nutrients in an aquaponic system compared to when they are grown in soil. Notably, regular monitoring of the ion concentration is necessary to prevent toxicity in aquaponics water (Graber and Junge, 2009; Endut et al., 2014). Researchers have noted that a balanced concentration of nutrient ions in aquaponic water is an important factor for plant growth (Goddek et al., 2015).
In a recirculating aquaponic system, nutrient accumulation is an indicator of the balance between plant nutrient use and fish waste production (Lennard, 2021). The ammonium ion is a nitrogen source and preferential for most plants at low external concentrations in aquaponics systems, but at higher concentrations, this ion causes phytotoxicity (Kiraly et al., 2013). At the initial stage of the aquaponics system, nitrifying bacteria were typically existed at insufficient levels. For this reason, ammonia levels build up, and after a period, ammonia becomes stable when it is converted into nitrates through nitrifying bacteria (Tyson et al., 2011). In addition, after the condition stabilizes, the cycled system is ready to grow plants. In this study, the ammonia concentration in water was increased in all treatments, and the increase was rapid between 15 and 20 days of treatment. Later, the ammonium ion concentration in water showed a more stable condition compared to the initial days of the treatment in all treated tanks (Fig. 3A). From 30 to 50 days of treatment, the ammonium ion concentration was around 1 mg·L-1 higher in the SO treatment than in the SV treatment. After 50 days of treatment, NH4+ was increased in all treatments compared to the initial stages. In addition, it was higher in the SS and SH treatments than in the SO and SV treatments. Continuously using the same water in a tank with the cycled process adds fish feed and fish by-products, resulting in increased ammonia in the aquaponic water.
Nitrification is a biological process in aquaponic systems that maintains the water quality by converting a toxic form of nitrogen (ammonia–nitrogen; NH3–N) into a non-toxic form, nitrate, through nitrite-oxidizing bacteria (NOB) (Yang and Kim, 2019; Su et al., 2020). Nitrate is an easily assimilated form of nitrogen for plants, which is an essential nutrient for plant growth in an aquaponic system that is also non-toxic for both fish and plants (Su et al., 2020). The concentration of NO3‒ in the water increased with the treatment period in the SS, SO, and SH treatments (Fig. 3B). At 50 days after treatment, it was increased by approximately twofold in the SS and SO treatments, and was increased by 37% in the SH treatment. However, NO3‒ fluctuated in the SV treatment, and from 23 to 26 days, it decreased sharply. Water of 5 L newly added at 22 days of treatment resulted in diluted concentrations of NH4+ and NO3‒ ions in the water of the SV treatment. The use of inorganic substrates is also a reason for the increased NH4+ and NO3‒ levels in the different treatments, as the inorganic substrates added nitrogen ions to the water without inhibiting microbial biomass buildup (Fauci and Dick, 1994).
The Mg2+ concentration in water increased up to 20 days of treatment in the SS, SO, SH, and SV treatments, and the rate of increase was rapid from 15 to 20 days (Fig. 3C). During these five days, the Mg2+ concentration was increased by approximately 70% in the SO, SH, and SV treatments and by around 1.5 times in the SS treatment. From 35 days of the treatment on, the concentration of Mg2+ remained stable at around 5 mg·L-1 in the SS and SV treatments, but it gradually decreased from 10 to 6.5 mg·L-1 in the SO treatment. The magnesium ion trend is similar to that of nitrogen ions. Pineda-Pineda et al. (2015) noted that Mg2+ and NO3‒ ions increased at a similar rate in the days after a treatment in an aquaponic system containing lettuce (Lactuca sativa L.) and tilapia (Rocky Mountain strain). Notably, food provided to the fish contains optimal amounts of Mg that is added to the water, playing a positive role in the growth and development of the plants.
The concentrations of Ca2+ in the SH, SO, and SS treatments were stable during 20−50 days of treatment (Fig. 3D). However, at 50 days after treatment, these levels were increased by two times in the SO treatment, and decreased by 19% in the SV treatment from their initial concentrations. The Ca2+ ion concentration in aquaponic water depends on the density of the fish and the amount of food; the variation in the pH also possibly affects the solubility of Ca. Mineralization of the food and absorption by plants are other factors that influence the variation of this nutrient in aquaponic water (Pineda-Pineda et al., 2015). This study also found that during the treatment period, the pH was sometimes highest in the SS treatment and lowest in the SH treatment, whereas the Ca2+ ion level was highest in the SH treatment but lowest in the SS treatment after 20 days (Fig. 3D).
From the initial day of the treatment to 30 days, the content of HPO42‒ in the water fluctuated (Fig. 3E). However, at 30 days after the commencement of the treatment, it was found to have increased in every case. After 50 days of treatment, the highest HPO42‒ level was observed in the SS treatment compared to the others. The lower concentration of HPO42‒ in the water was a consequence of the higher amount of Ca2+ ions in the system. Other researchers have reported that calcium is able to bind phosphorus, leaving fewer free phosphate ions available for the plants in the aquaponics water (Cerozi and Fitzsimmons, 2016; Maucieri et al., 2019). A similar inverse relationship between calcium ions and the phosphate ion concentration in water was observed in an aquaponic system that included common carp (Cyprinus carpio L.) and lettuce (Lactuca sativa L.) (Maucieri et al., 2020). In this study, similar to other studies, SS, SH, SO, and SV showed inverse patterns based on their concentration chronologies of the HPO42‒ and Ca2+ ions in water. The newly added 5 L of water is also a reason for the reduced concentrations of Mg2+, Ca2+, and HPO42‒ ions in the water of the SV treatment (Fig. 3E). Noh et al. (2011) also noted that Mg2+, Ca2+ and HPO42‒ ion concentrations were more stable in nutrient water after the initial stage in an ebb-and-flow hydroponic system that grew kalanchoe plants. Other studies have also shown that the concentrations of Mg2+, Ca2+, and HPO42‒ ions increased when the EC of the nutrient water was increased in an ebb-and-flow hydroponic system (Lu and Son, 2006; Noh and Son, 2010).
Plant Growth Parameters
At 25 and 50 days after treatment, the plant height and width were not significantly different between plants grown in the SS, SO, SH, and SV treatments (Figs. 4, 5A, and 5B). During this period, similar pH fluctuations and the increasing patterns of EC in the aquaponic water of all treatments are possible reasons for the non-significant variation of the plant heights and widths. Differences in nutrient concentrations in a close range are also significant factor. Furthermore, the quality and intensity of the light received by a plant are important factors that influence plant growth and enlargement (Bian et al., 2015). Other studies also showed that variations in light availability among different plants influenced their enlargement when grown in soil (Shawon et al., 2021; Kim et al., 2022). Kim et al. (2021) showed that the height of apple seedlings was statistically not different when they were grown in a substrate medium with the same light intensity provided. In this study, we used light-emitting diodes (LEDs) emitting white light and the same light intensity for all treatments under controlled environmental conditions. These are other reasons for the plant height being statistically similar in all substrates that were used as growing media.
The number of leaves, the leaf length, and the leaf width of the plants did not show statistical differences among the treatments (Table 1). Generally, photosynthesis of a plant depends on the chlorophyll content in its leaves (Porcar-Castell et al., 2014). In this study, the chlorophyll content was determined by SPAD readings. From Table 1, at 50 days after treatment, the SPAD value in the leaves was not significantly different in the SS, SO or SV treatments. Similar leaf features (length, width) are possible reasons for the statistically similar SPAD values in the leaves of plants among these treatments. Jifon et al. (2005) and Marenco et al. (2009) also showed that the SPAD value of a plant depends on its leaf shape. However, at 50 days after treatment, the SPAD value in the SH-treated plants was significantly higher compared to those grown under the other treatments (Table 1). A higher EC and higher concentrations of the Ca2+, NH4+, NO3‒ and Mg2+ in the water of the SH treatment may be reasons for the high SPAD value in the corresponding plants. Khayyat et al. (2009) noted that calcium ions increased the chlorophyll concentration in leaves. As well, it can enhance the electron transport capacity in the photosystem of a plant (He et al., 2018). Furthermore, decreased pattern of SPAD value in 50 days-treated plants compared to 25 days-treated plants in all treatments (Table 1) may be the reason of combinations of the plant age, treatment period and water quality (i.e., nutrient concentration, EC and pH).
Table 1.
Effects of inorganic substrates of sponge (SS), orchid (SO), hydroball (SH), and volcanic rock (SV) on the plant growth characteristics of K. blossfeldiana in an aquaponics system (n = 5)
DATz | Treatment | Plant growth characteristics per plant | |||
Leaf |
SPAD (values) | ||||
Length (cm) | Width (cm) | Number (no.) | |||
25 | SS | 4.7 ay | 3.3 a | 19.4 a | 34.5 b |
SO | 5.5 a | 3.7 a | 19.0 a | 43.1 a | |
SH | 5.1 a | 3.5 a | 19.4 a | 44.1 a | |
SV | 5.1 a | 3.5 a | 19.0 a | 41.8 ab | |
50 | SS | 6.0 a | 4.2 a | 20.2 a | 31.6 b |
SO | 6.6 a | 4.4 a | 20.2 a | 35.5 b | |
SH | 6.8 a | 4.5 a | 21.0 a | 42.9 a | |
SV | 6.4 a | 4.3 a | 21.4 a | 36.8 b |
Furthermore, the stem diameter, number of branches, and number of nodes for a plant were not significantly different among the SS, SO, SH, and SV treatments (Fig. 5C, 5D, and 5E). The similar number of leaves and the similar SPAD value in the leaves indicate that the photosynthesis rates and physiological activities of the plants were similar in all treated plants. For this reason, the stem diameter, number of branches, and number of nodes of the plants in all treatments did not show statistical differences. In nature, kalanchoe is compact in habit, and a succulent plant (Currey and Erwin, 2010). Furthermore, 50 days is a very short period for this plant growth. This is another reason why all treatments showed no significant effects on the plant growth parameters.
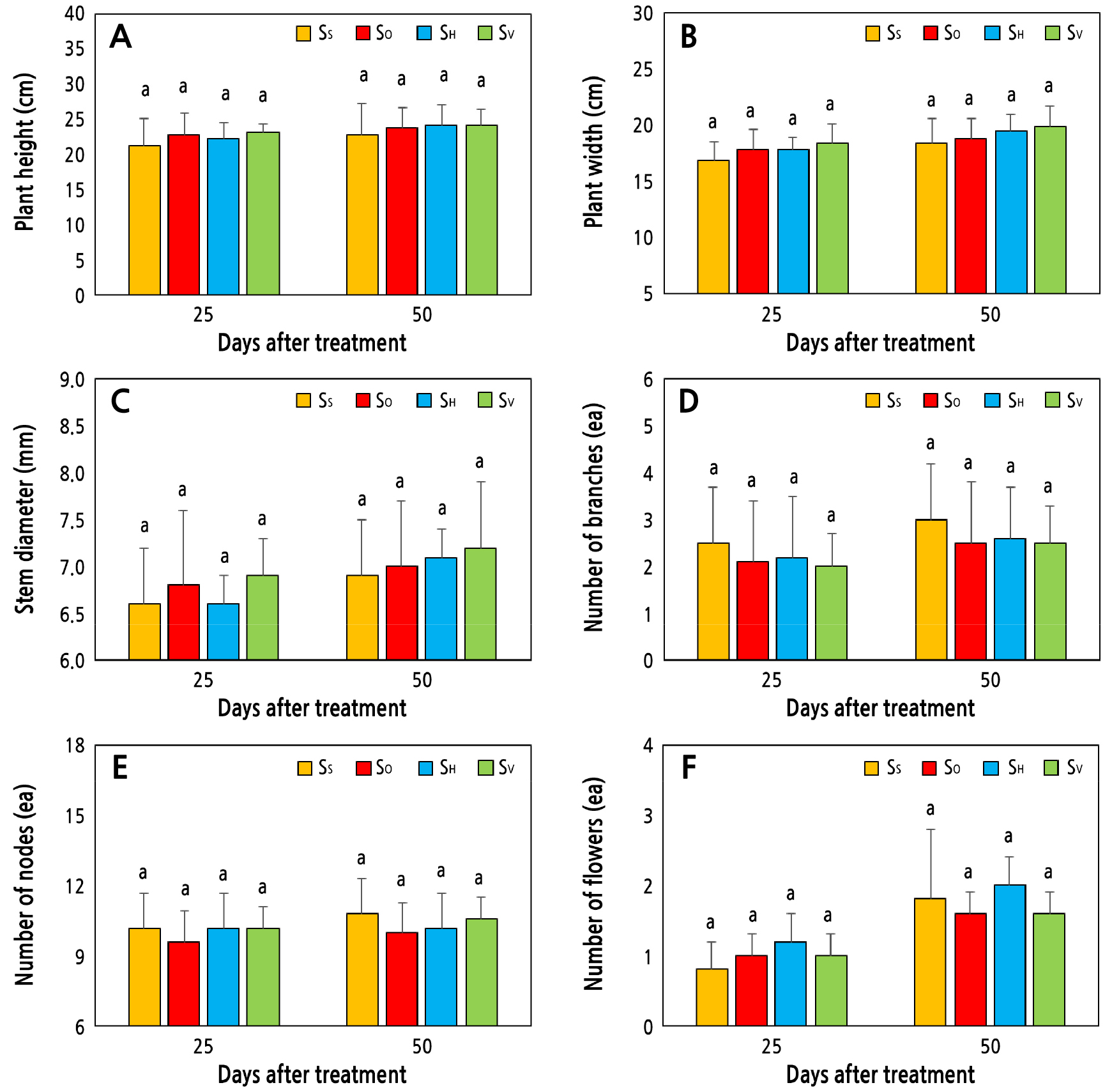
Fig. 5.
Effects of inorganic substrates of sponge (SS), orchid (SO), hydroball (SH), and volcanic rock (SV) on the (A) plant height, (B) plant width, (C) stem diameter, (D) number of branches, (E) number of nodes, and (F) number of flowers of K. blossfeldiana plants. The same lowercase letters above the bars within the figure indicate non-significant differences based on Duncan’s multiple range test at p < 0.05.
The flower is an important organ of plants, containing several phytochemicals (Bachheti et al., 2020), and the development or production of flowers greatly depends on the growing environment and health conditions of a plant (Borghi et al., 2019; Lim et al., 2020). The numbers of flowers in this study were not significantly different in the SS, SO, SH, or SV treatments (Figs. 5F and 6). Although the strength of EC was different among the treatments, its increasing pattern was similar during the treatment period (Fig. 2A). Furthermore, the fluctuations of the pH range were very similar among the treatments (Fig. 2B). In addition, other plant growth parameters such as the plant height and width and the numbers of leaves, branches and nodes were similar in all treatments at 25 and 50 days (Table 1 and Fig. 5). These factors may be cumulatively responsible for the non-significant variation in the number of flowers in the plants among the treatments (Figs. 5F and 6).
Conclusion
The strength of EC was greater in the water used with the hydroball substrate, and its pH was also more stable than the other cases. The nutrient concentrations of the aquaponics water that was circulated through the sponge, orchid stone, hydroball, and volcanic rock substrates differed. However, these treatments did not show significant variations in the growth of K. blossfeldiana owing to the compact and succulent characteristics of this plant as well as the short treatment period. Therefore, among the inorganic substrates, any of the four would be suitable for an aquaponic system that utilizes K. blossfeldiana and Carassius auratus auratus. Furthermore, the period of the first 20 days of this system is important for stabilizing the ion concentrations in the water. In future research, we will investigate the factors of the fish density and feed dose on the growth of plants and fish in the aquaponic system as a planterior component including the impacts of these factors on the physiological response and bioactive compounds in the plants.