Introduction
Materials and Methods
Plant materials
Low-light treatment
Growth response and photosynthetic activity
Statistical analysis
Results
Growth response under low-light conditions
Photosynthetic efficiency under low-light conditions
Discussion
Conclusion
Introduction
The United Nations, in its World Urbanization Prospects, points out that urban populations are expected to grow dramatically, with projections suggesting that 68% of the world population will reside in urban areas by 2050 (United Nations, 2018). This urban expansion is occurring at a faster rate than population growth, resulting in the fragmentation of landscapes that were previously dominated by agriculture and native vegetation (Williams et al., 2015; d’Amour et al., 2016). Such urbanization trends contribute to significant environmental changes, including alterations in light availability, which is a critical factor influencing plant survival, growth, and community dynamics (Canham et al., 1990; Valladares and Niinemets, 2008).
The construction of buildings and infrastructure in urban environments has resulted in reduced light availability, which has subsequently affected the quality or quantity of light available to plants (Wirz-Justice et al., 2021). In response to these altered light conditions, plants exhibit phenotypic plasticity, modifying their morphological and physiological traits to enhance their light capturing and utilization capabilities. Plants that are tolerant of shade demonstrate an enhanced capacity for photosynthesis and physiological adaptations in low-light conditions, including increased chlorophyll contents and specific leaf areas (Sultan, 2000; Delagrange et al., 2004).
Hosta plants, renowned for their tolerance of shaded conditions and adaptability to high soil moisture, are frequently selected as ornamental plants in gardens and urban green spaces (Lee et al., 2018). Hosta species are native to East Asia, with a variety of species predominantly found in Northeast Asia, including Korea (Lee et al., 2019). They offer the potential for ornamental and horticultural use (Lee and Maki, 2015).
Korean native hostas are classified into several taxa, with ongoing research focusing on their ecological characteristics and horticultural potential (Lee et al., 2018). Despite their popularity, detailed studies of the horticultural suitability of Korean wild Hosta species under varying light conditions remain scarce. To enhance urban ecosystems through the introduction of diverse plant resources, it is essential to integrate novel species that demonstrate resilience to urban environments and possess horticultural merit. A review of the urban shade tolerance of Korean wild hostas with horticultural value would be a valuable contribution to the advancement of urban horticulture. The two Korean Hosta species, H. minor and H. yingeri, are endemic to the Korean peninsula and are highly prized for their ornamental value, despite their different habitats and appearances. H. minor, which is diminutive in stature, is found across the entirety of the Korean Peninsula. Conversely, H. yingeri, characterized by fleshy, robust, and broad dark green leaves, has a markedly restricted habitat confined to Heuksando Island (Lee et al., 2018). It is therefore imperative to assess its environmental tolerance to ascertain its viability as a landscaping and gardening material. The aim of this study is to examine the growth persistence of two Korean wild Hosta species under low-light conditions, with a view to evaluating their potential for diverse horticultural applications. By examining parameters such as the leaf number, shoot biomass, and physiological responses, including their photosynthetic efficiency and chlorophyll fluorescence, this research aims to enhance our understanding of how these species adapt to urban garden environments characterized by reduced light availability.
Materials and Methods
Plant materials
Seedlings of H. minor and H. yingeri were provided by the Korea National Arboretum in May of 2020 and were cultivated in a greenhouse at the University of Seoul until November of 2021, followed by a two-month period of natural cold forcing outdoors. In February of 2022, the plants were transferred back to the greenhouse and transplanted into plastic rectangular pots (dimensions: 11.5 cm in width, 11.5 cm in length, and 10 cm in height) filled with artificial soil (Baroker, Seoul Bio, Eumseong, Korea). A granular fertilizer (15–9–12+2MgO+TE, Osmocote Exact, EVERRIS, Heerlen, Netherlands) was incorporated into the soil at a concentration of 1.8 g/L. Two weeks prior to the initiation of the experiment, one tiller per seedling was standardized to six leaves. Only individuals with healthy leaves and comparable leaf forms were selected for the study. Twenty plants per treatment were provided for destructive and non-destructive analyses.
Low-light treatment
The selected plant materials were transferred to an indoor growth room with 12 open chambers and subjected to low-light conditions for 12 weeks (from 15 March to 31 May 2022) without prior acclimatization to observe their adaptation. The light intensity levels were set to 10, 30, and 50 µmol·m-2·s-1 PPFD, designated as L10, L30, and L50, respectively. During the experiment, the growth room was maintained at an air temperature (AT) of 23 ± 2°C and at 40 ± 10% relative humidity (RH), with a 14-hour photoperiod, as utilized in a previous report (Yang et al., 2022). Plants were irrigated with 0.2 L of tap water per pot twice per week.
Growth response and photosynthetic activity
The number of fully developed leaves was counted on a weekly basis for a period of 12 weeks, with the exclusion of etiolated leaves. The leaf area (LA) and shoot weight (fresh weight and dry weight; FW and DW) were measured after the 12-week light intensity treatment. The samples were then repacked and dried for 72 hours, after which the shoot dry weight in each case was measured. The specific leaf area (SLA) was calculated by dividing LA by DW.
To detect the chlorophyll content, leaves were washed with distilled water and cut into 2 mm sections. Forty milligrams of plant samples were placed in 10 mL of 100% dimethyl sulfoxide (DMSO) and heated at 65°C in a constant-temperature water bath for six hours. After adequate cooling, the absorbance of the resulting extracts was measured using spectrophotometry (UV-3100, Shimadzu, Kyoto, Japan). Results were calculated according to Barnes et al. (1992).
Photosynthetic values were obtained from fully developed leaves using a portable photosynthesis system (LI-6800, LI-COR, Lincoln, NE, USA) at 400 µmol·m-2·s-1 photosynthetic photon flux density (PPFD). The net photosynthetic rate was measured under the following conditions: 23°C AT, 50% RH, 5000 rpm in flow rate, and 400 ppm CO2. To measure chlorophyll fluorescence, plants were kept in the dark for 15 minutes, after which the chlorophyll fluorescence values were recorded using a portable photosynthesis system (LI-6800, LI-COR, Lincoln, NE, USA). An intense light beam of 8000 µmol·m-2·s-1 was projected onto the dark-treated leaves and the dark phase values (Fo, Fm, Fv, Fv/Fm, and OJIP curve) were measured. Non-photochemical quenching (NPQ) was determined after exposing the plant materials to a saturated light intensity level of 800 µmol·m-2·s-1 for the two wild Hosta genotypes.
Statistical analysis
An analysis of variance (ANOVA) was performed using the SAS statistical software package (ver. 9.4, SAS Institute Inc., Cary NC, USA). The difference between the means was evaluated using Duncan’s multiple range test (DMRT, p = 0.05).
Results
Growth response under low-light conditions
The two Hosta species displayed divergent growth responses when subjected to low-light conditions of L10, L30, and L50 (Table 1, Figs. 1, 2, and 3). As illustrated in Fig. 1, the visual biomass of the initial plant materials of both species exhibited an increased at L50, whereas a reduction was observed at the lowest light intensity value of 10 µmol·m-2·s-1 (L10). Meanwhile, leaf tip drying symptoms were noted, as shown in Fig. 1. This symptom was only observed on existing leaves in the first week of treatment and not on newly emerging leaves in all treatments. As there was no acclimatization period at the time of treatment, this is likely to be an adaptive response to the difference in humidity between the nursery greenhouse and the indoor treatment environment, and there was no effect at the treatment level.
Table 1.
Plant growth responses of two Korean endemic Hosta species grown under low-light conditions for 12 weeks
Species | Light intensity | Leaf number | Leaf area (mm2) | SPAD | FW (g) | DW (g) | SLA (mm2·g-1) |
H. minor | Initial value | 6.4 bcz | 118.6 a | 35.4 ab | 2.88 a | 0.45 a | 272.3 b |
L10 | 4.4 c | 85.0 a | 22.5 b | 2.11 a | 0.24 a | 341.0 a | |
L30 | 9.9 ab | 205.1 a | 42.9 a | 5.78 a | 0.79 a | 269.0 b | |
L50 | 11.4 a | 197.0 a | 40.1 a | 5.01 a | 0.76 a | 257.3 b | |
H. yingeri | Initial value | 6.0 b | 126.4 c | 37.8 b | 3.62 c | 0.55 b | 223.2 b |
L10 | 3.4 c | 101.0 c | 37.3 b | 3.04 c | 0.32 b | 317.7 a | |
L30 | 9.2 a | 222.3 b | 46.3 ab | 7.30 b | 1.06 a | 211.2 b | |
L50 | 10.9 a | 352.3 a | 52.9 a | 10.45 a | 1.42 a | 249.0 ab | |
Significance | |||||||
Species (A) | ns | ns | * | * | * | * | |
Light treatment (B) | *** | *** | ** | * | * | * | |
A × B | ns | ns | ns | ns | ns | ns |
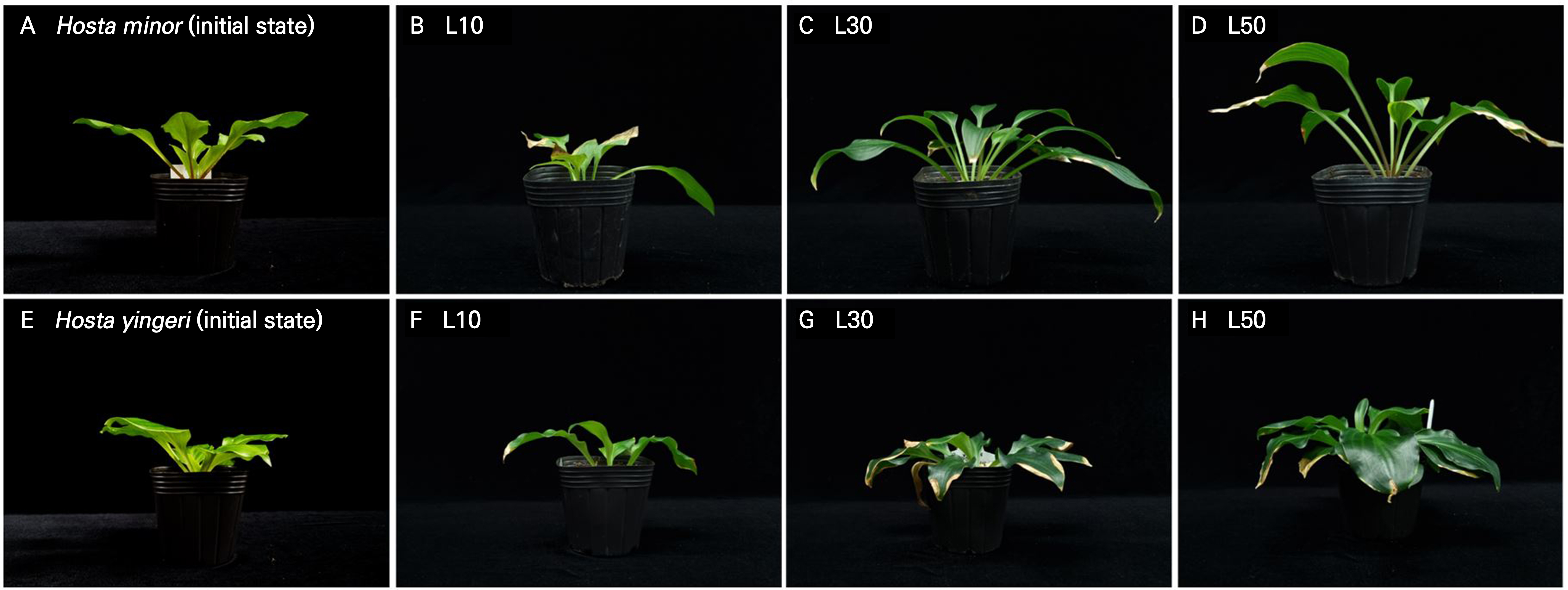
Fig. 1.
Visual growth status of two Korean endemic Hosta species grown under low-light conditions for 12 weeks. A, B, C, and D: Hosta minor; E, F, G, and H: Hosta yingeri. A and B: initial state. L10, L30, and L50 represent 10, 30, and 50 µmol·m-2·s-1 PPFD, respectively. The pot size is 8 cm × 7 cm (upper diameter × height).
H. minor showed the best overall growth under the L30 and L50 conditions, with significant increases in the leaf number, leaf area, SPAD, FW, and DW compared to the initial values and L10. H. yingeri showed a similar trend, with the most significant growth parameters observed under the L50 condition, followed by L30 (Table 1). Overall, the low light intensity of 10 µmol·m-2·s-1 (L10) resulted in reduced or limited growth compared to the initial values, whereas increased light intensity levels (L30 and L50) had a positive effect on the growth of both species. The initial leaf number, leaf area, and shoot biomass (DW) were typically reduced by an average of 31.3%, 28.3%, and 46.7% in H. minor and by 43.3%, 20%, and 41.8% in H. yingeri at L10 during the 12-week experiment (Fig. 2). Furthermore, a decline in the leaf number was evident from week 4 onwards under the L10 condition, with reductions of ‒31.3% in H. minor and ‒43.3% in H. yingeri in comparison to the initial values (Fig. 3). The leaf area (LA) and specific leaf area (SLA) were also significantly reduced in the L10 case compared to baseline, i.e., the initial value (Table 1). This indicates that L10 is not sufficient to support optimal growth for these species, highlighting their need for higher light intensity levels to thrive. Based on the observations above, growth persistence, as measured by the growth responses in both species, was not achieved at the lowest light intensity (L10). It can be inferred that the threshold for growth persistence for both species lies between L10 and L30 for both H. minor and H. yingeri. Specifically, L10 is below the threshold, as this condition led to reduced growth, whereas L30 is above the threshold, leading to enhanced growth.
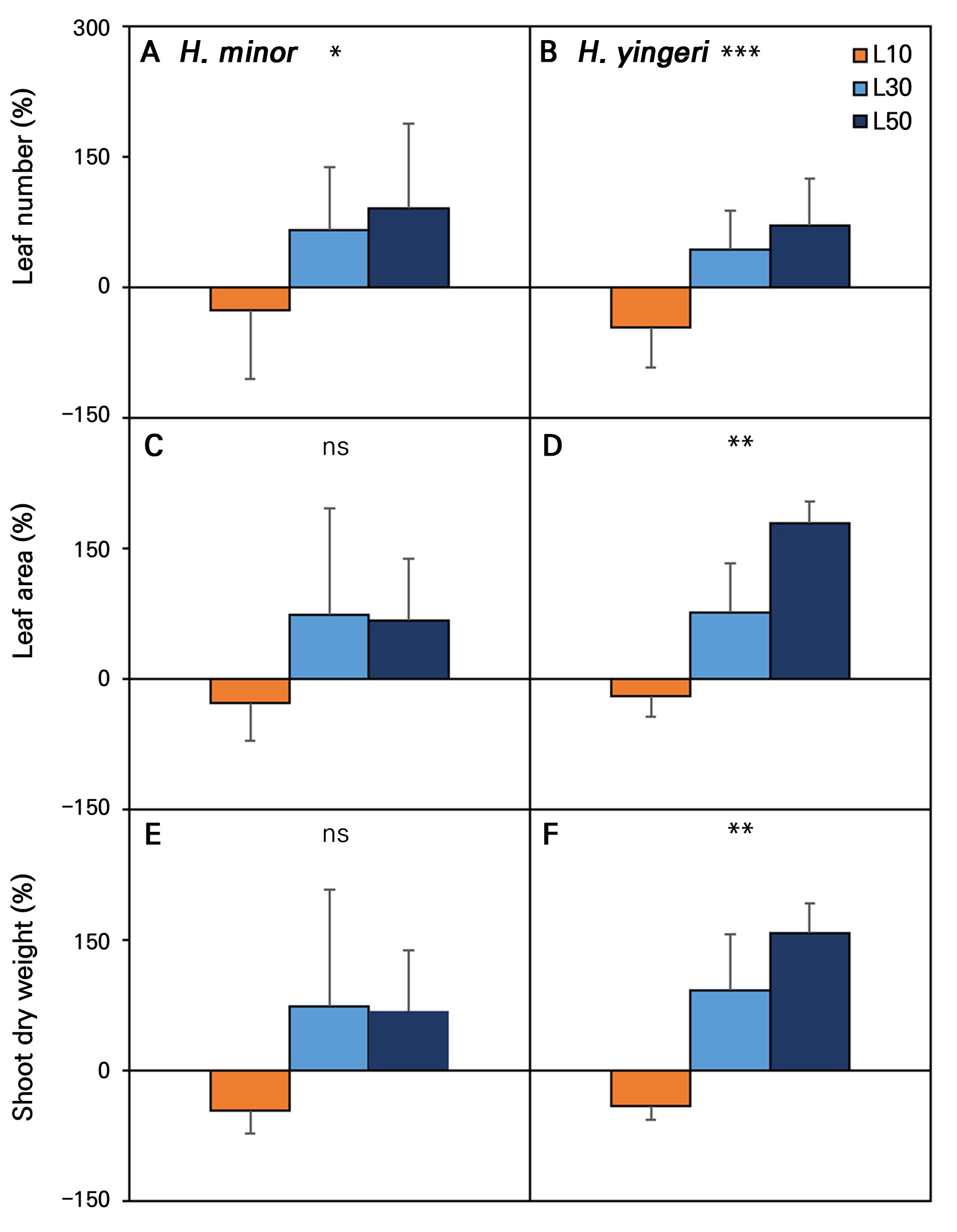
Fig. 2.
Relative comparison of growth persistence levels of two Korean endemic Hosta species grown under low-light conditions for 12 weeks with their initial value (0%). A, C, and E represent H. minor, and B, D, and F denote H. yingeri. L10, L30, and L50 indicate 10, 30, and 50 µmol·m-2·s-1 PPFD, respectively. Vertical bars denote the standard error (n = 10). Ns, *, **, and *** indicate non-significant and significant differences at p < 0.05, 0.01, and 0.001, respectively, according to an ANOVA.
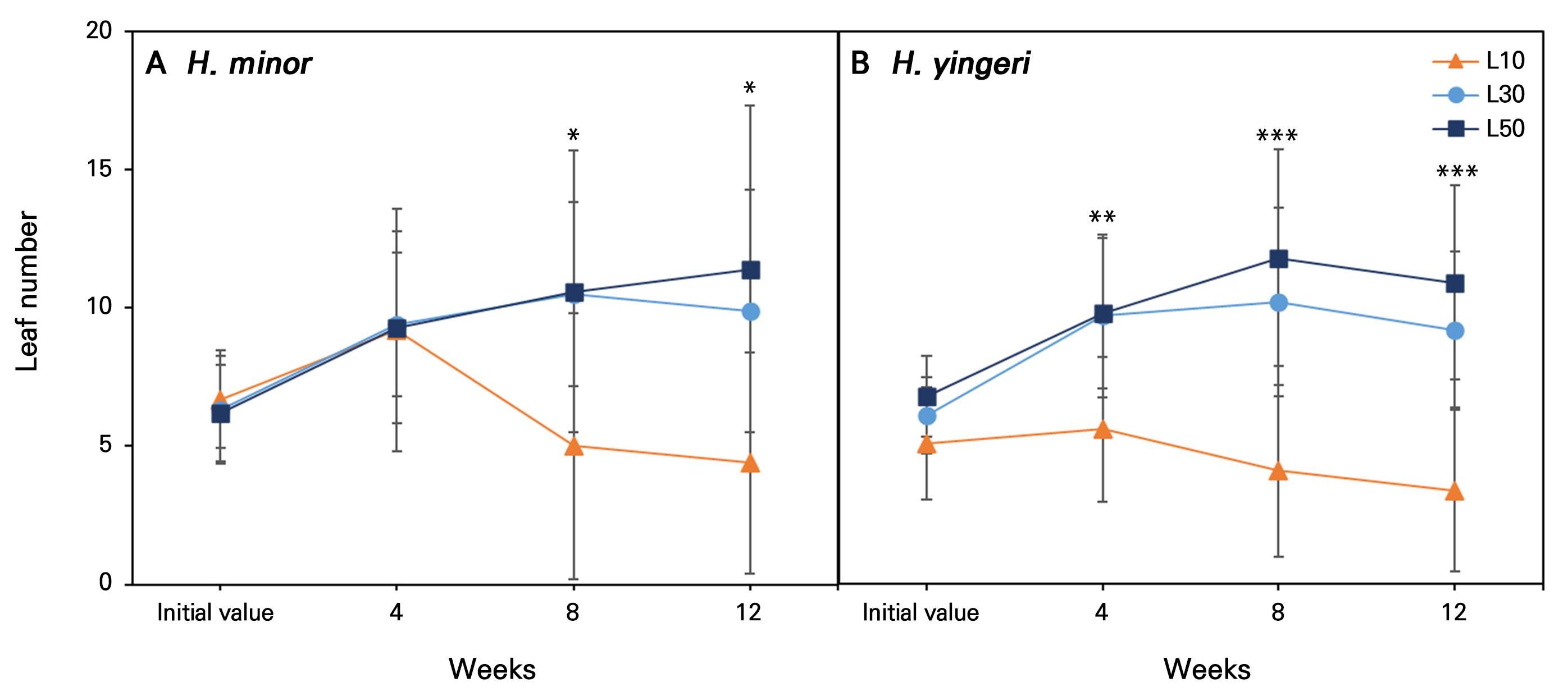
Fig. 3.
Changes in the leaf number of two Korean endemic Hosta species grown under low-light conditions for 12 weeks. L10, L30, and L50 denote 10, 30, and 50 µmol·m-2·s-1 PPFD, respectively. Vertical bars indicate the standard error (n = 10). *, **, and *** indicate significant differences at p < 0.05, 0.01, and 0.001, respectively, according to an ANOVA.
Photosynthetic efficiency under low-light conditions
As shown in Table 1, low-light conditions deepened the color of the leaves (SPAD). The total chlorophyll content (a + b) also increased dramatically, up to 6.6-fold in H. minor (L30) and by 13-fold in H. yingeri (L50) under the 12-week low-light condition compared to the initial values (Table 2). This considerable increase in the chlorophyll content was primarily due to an increase in chlorophyll a, which increased 7.8-fold in H. minor under L30 and 17-fold in H. yingeri with the L50 condition. The a/b ratio of the chlorophyll content increased maximally by 2.2-fold in H. minor (L10) and by 2.7-fold in H. yingeri (L50).
Table 2.
Chlorophyll contents of two Korean endemic Hosta species grown under low-light conditions for 12 weeks
Species | Light treatment | Chl a | Chl b | Chl a+b | Chl a/b ratio |
H. minor | Initial value | 1.44 cz | 0.64 d | 2.08 c | 2.25 b |
L10 | 6.52 b | 1.31 c | 7.83 b | 4.97 a | |
L30 | 11.32 a | 2.44 a | 13.76 a | 4.64 a | |
L50 | 8.85 ab | 1.98 b | 10.83 ab | 4.47 a | |
H. yingeri | Initial value | 0.75 c | 0.44 c | 1.19 c | 1.73 b |
L10 | 7.37 b | 1.50 b | 8.87 b | 4.93 a | |
L30 | 12.81 a | 2.69 a | 15.50 a | 4.77 a | |
L50 | 10.69 ab | 2.28 a | 13.00 ab | 4.69 a | |
Significance | |||||
Species (A) | ns | ns | ns | ns | |
Light treatment (B) | *** | *** | *** | *** | |
A × B | ns | ns | ns | ns |
To determine the relative photosynthetic efficiency under low-light conditions, the photosynthetic rate was measured at four-week intervals and plotted against an initial value of 100% (Fig. 4). Compared to the initial value (100%), the photosynthetic activity of both species demonstrated a notable decline over the 12-week period, particularly at lower light intensities. The reduction in photosynthesis was more pronounced in H. yingeri than in H. minor. Over time, photosynthesis exhibited a tendency to converge towards the initial value at L50 in H. minor under the L50 condition.
Conversely, the chlorophyll fluorescence indicators (Fo, Fm, Fv, Fv/Fm, and NPQ) in both species exhibited less variability than the photosynthetic reactions under low-light conditions (Table 3). The values of Fo, Fm, and Fv tended to be higher in H. yingeri than in H. minor. However, no significant difference was demonstrated in the Fv/Fm or NPQ values between the two species. Both species exhibited Fv/Fm values within the normal range of 0.082–0.83, irrespective of the light level at week 12 (Table 3). H. minor exhibited a higher OJIP value under L10 compared to that under L30 and L50, while H. yingeri showed a slight increase under the L10 and L30 conditions in comparison to L50. These outcomes are attributable to the elevated values of Fo, Fm, and Fm. Furthermore, the OJIP kinetics showed typical patterns without distortion or unusual inflections (Fig. 5).
Table 3.
Chlorophyll fluorescence in a dark-adapted phase and NPQ of two Korean endemic Hosta species grown under different light conditions (L10, L30, and L50) for 12 weeks
Species | Light treatment | Fo | Fm | Fv | Fv/Fm | NPQ |
H. minor | Initial value | 276.2 az | 1567.2 a | 1290.9 a | 0.82 b | - |
L10 | 267.4 ab | 1567.4 a | 1300.0 a | 0.83 ab | 1.66 a | |
L30 | 252.1 b | 1537.4 ab | 1285.3 a | 0.84 a | 1.89 a | |
L50 | 254.7 b | 1462.3 b | 1207.6 b | 0.83 b | 1.55 a | |
H. yingeri | Initial value | 292.6 a | 1630.8 a | 1338.2 a | 0.82 a | - |
L10 | 279.6 a | 1636.9 a | 1357.3 a | 0.83 a | 1.47 a | |
L30 | 272.8 a | 1619.9 a | 1347.1 a | 0.83 a | 1.47 a | |
L50 | 272.3 a | 1570.1 a | 1297.9 a | 0.83 a | 1.55 a | |
Significance | ||||||
Species (A) | * | ** | ** | ns | ns | |
Light treatment (B) | ns | ns | ns | ns | ns | |
A × B | ns | ns | ns | ns | ns |
Discussion
The results above highlight the adaptive mechanisms of Hosta species in response to low-light conditions. Both H. minor and H. yingeri displayed increased chlorophyll contents (Table 2) and maintained stable chlorophyll fluorescence parameters (Table 3), suggesting efficient light capture and utilization under suboptimal light intensities. However, their growth and photosynthetic rates were significantly compromised at the lowest light intensity (L10), with better performance observed at higher light levels (L30 and L50). Growth responses, specifically biomass accumulation and the leaf number, leaf area, and shoot biomass, were significantly influenced by the intensity of the light to which the plants were exposed (Table 1).
Both H. minor and H. yingeri exhibited enhanced growth under moderate to higher low light intensities (L30 and L50). The observed increase in growth metrics at L30 and L50 can be attributed to the plants' ability to optimize their light-harvesting capabilities and allocate resources efficiently under these conditions (Wen et al., 2005; Lee et al., 2022a). Conversely, the lowest light intensity (L10) led to a marked reduction in these parameters, indicating suboptimal conditions for growth. Under L10, both species showed reduced growth compared to the initial values for the leaf number, leaf area, and shoot biomass (Fig. 2).
The decreases in both the biomass and the carbohydrate content of the Petunia 'Pretty Flora' under low-light conditions are attributable to reduced photosynthesis (Kwack and Lee, 1999). Similarly, even plants with relatively high shade tolerance, such as Hylotelephium species or Delosperma cooperi, show poor growth when exposed to light intensities below their preferred thresholds (Lu et al., 2003; Lee et al., 2022b; Nam et al., 2022). Moreover, the observed recovery of the leaf number and rate of photosynthesis after eight weeks can be interpreted as a physiological trait of both species tested here, whereby at least eight weeks are required for acclimatization to low-light conditions (Yang et al., 2022). It has been demonstrated that alterations in light availability exert a significant influence over plant survival, growth and the dynamics of plant communities.
The photosynthetic efficiency, as measured by the chlorophyll content (Table 2) and relative photosynthetic rates (Fig. 4), showed adaptive responses to low-light conditions. Both species showed dramatically increased chlorophyll contents, particularly chlorophyll a, which is crucial for capturing light energy. This adaptation likely serves to maximize light absorption in low-light environments. However, despite this increase in the chlorophyll content, the photosynthetic rate declined at lower light intensities, particularly under L10. This reduction in the photosynthetic activity under low light can be attributed to the limited photon flux, which directly affects the light-dependent reactions of photosynthesis (Torres et al., 2021). The reflection of the photosynthesis process by chlorophyll fluorescence is a well-established phenomenon, as evidenced in numerous previous reports (Stirbet and Govindjee, 2011).
While the entire plant or leaf is maintained in darkness, the photosystem II (PSII) reaction centers reach a stable state that can accept a photosynthetically active photon, which is referred to as being ‘open’ (Murchie and Lawson, 2013). When the PSII reaction centers are in an ‘open’ state, a weak measuring beam of approximately 0.1 µmol·m-2·s-1 induces reductions in QA and Fo. Subsequently, a short actinic pulse with a high PPFD is employed to measure Fm. Once chlorophyll fluorescence has reached Fm, the reduction of QA is at its maximum (Strasser et al., 2004). Therefore, it can be posited that the reductions of both Fo and Fm in two Hosta species following the treatments are attributable to a smaller QA pool than that observed initially (Table 3). The chlorophyll fluorescence parameters (Fo, Fm, Fv, Fv/Fm, and NPQ) showed less variability than the photosynthetic rates under low-light conditions (Table 3). Both species maintained Fv/Fm values within the normal range (Lee et al., 2020), indicating that the PSII was not severely damaged by the low light intensities. This stability of the fluorescence indicators suggests that both H. minor and H. yingeri possess mechanisms to protect their photosynthetic apparatus under low-light conditions, as reported by Yang et al. (2022).
The OJIP curves, which reflect the detailed kinetics of chlorophyll fluorescence, did not show any unusual patterns or distortions (Fig. 5), further confirming the stability of PSII in both species. In a previous report (Benkov et al., 2019), a sun-resistant plant was observed to maintain constant maximum quantum efficiency of PSII under variable light intensities. In contrast, a shade-tolerant plant exhibited variable PSII photochemical activity, which was found to be dependent on the growth light intensity. In this study, H. minor and H. yingeri, as shade-tolerant plants, showed greater plasticity under low-light conditions, accompanied by a corresponding decrease in the chlorophyll fluorescence values. H. minor exhibited higher OJIP values under L10 compared to those under L30 and L50, while H. yingeri showed a slight increase in OJIP values under the L10 and L30 conditions relative to that under L50. These results imply that despite the reduced photosynthetic efficiency at lower light intensities, both species can maintain the structural integrity of their photosynthetic machinery.
Conclusion
This study highlights the differential adaptive strategies of wild Hosta species to low-light environments. While H. minor displays greater photo-physiological plasticity, making it more suited to fluctuating light conditions, H. yingeri shows less resilience to extremely low light, with a more significant decline in its photosynthetic activity. The light intensity range of L10 to L30 is critical for the growth of both species, with growth limited below this range and sustained above it. These findings provide insights into ecological strategies and inform horticultural practices in relation to these species.