Introduction
Materials and Methods
Plant materials and treatments
Determination of SSC, and TA
Measurement of VC and firmness
Measurement of ethylene production and respiration rate
qRT-PCR RNA isolation and transcript analyses
Extraction and determination of GABA
Phylogenetic analysis
Transient overexpression and silencing of AaGAD1 and AaGAD4 in kiwifruit
ACS and ACO activities
Statistical analysis
Results
Effects of GABA treatment on fruit quality during the storage period
Effects of GABA treatment on fruit ripening during the storage period
Ethylene production inhibited by the biosynthesis of endogenous GABA catalyzed via AaGAD1 or AaGAD4
Effects of exogenous GABA treatment on the expression patterns of genes related to ethylene biosynthesis
Discussion and Conclusion
Introduction
Hardy kiwifruit (Actinidia aruguta), is a perennial deciduous vine fruit belonging to the Actinidiaceae family. The domesticated fruit has experienced a surge in popularity and commercial production on a global scale. It is currently cultivated and distributed in several countries, including China, Italy, New Zealand, and Greece (Latocha et al., 2011; Garcia et al., 2013; Latocha et al., 2014; Wang et al., 2015). Currently, there is a growing trend among consumers to favor fruits with a smooth, hairless surface, distinctive and tasty flavor (Zhu et al., 2019). Significantly, this fruit is characterized by a substantial presence of lutein, phenolics, vitamin C, and various minerals, notably phosphorus, calcium, iron, and zinc. Consequently, it can be classified as a berry fruit with considerable nutritional significance (Nishiyama et al., 2004; Fisk et al., 2006; Song et al., 2022).
The popularity of consuming hardy kiwifruit due to its nutritional profile is widely acknowledged among consumers. However, similar to other varieties of kiwifruit, the storage of hardy kiwifruits after harvest continues to present significant challenges (Song et al., 2022). Due to the softness of ripened hardy kiwifruit, it is typically harvested at a physiologically mature and firm stage to facilitate packaging and transportation (Fisk et al., 2006). Nevertheless, the rapid softening of hardy kiwifruit after harvest poses a significant challenge for industrial use, thereby severely limiting the feasibility of large-scale production (Song et al., 2022). Kiwifruit has the potential to be preserved under refrigeration conditions, specifically at a temperature of 0°C and a relative humidity of 90–95%. When stored under these conditions, kiwifruit can maintain good quality for a period of 3–6 months. In contrast, the shelf life of hardy kiwifruit is limited to only 1–2 months when stored in the same manner (Fisk et al., 2006). As a result, the demand for hardy kiwifruit consistently surpasses the available supply in the market. Therefore, it is of utmost importance to explore efficient strategies for prolonging the storage shelf life of hardy kiwifruit.
Kiwifruit, being a representative climacteric fruit, has the ability to generate autocatalytic ethylene during fruit ripening (Yin et al., 2008). After a few days following the harvest of kiwifruit, there is a notable acceleration in its respiration rate, indicating the onset of fruit ripening. Simultaneously, the emission of ethylene at an accelerated pace leads to a rapid softening and deterioration of the fruit’s flesh. Consequently, the shelf life of kiwifruit is significantly reduced (Sharma et al., 2012). Over the course of several decades, numerous strategies have been implemented to extend the shelf life of kiwifruit by inhibiting the production of ethylene and reducing respiration rate. Prior studies have demonstrated that 1-methylcyclopropene (1-MCP) as a cyclic olefin compound can effectively hinder the activity of ethylene, leading to the suppression of ethylene, delayed softening, and reduced total soluble solid (TSS) content in ‘hayward’ kiwifruit (Boquete et al., 2004; Lim et al., 2016). The application of melatonin as a treatment was found to effectively delay the softening of kiwifruit through the suppression of both the respiration rate and release of ethylene during storage duration (Cheng et al., 2022).
The primary metabolic pathway for GABA is the GABA shunt, which effectively circumvents two steps of the tricarboxylic acid (TCA) cycle (Bouché and Fromm, 2004). The biosynthesis of GABA involves the initial consumption of proton and glutamate, which is catalyzed by the enzyme glutamate decarboxylase (GAD) in an irreversible manner. Subsequently, GABA is metabolized by the mitochondrial enzymes GABA transaminase and succinic semialdehyde dehydrogenase sequentially (Bown and Shelp, 1997). GABA, an essential signaling molecule, exerts a significant influence on various aspects of preharvest and postharvest physiology in numerous horticultural products. Specifically, it is involved in the provision of nitrogenous compounds to cells, regulation of cellular osmotic balance, and the removal of harmful free radicals, particularly in the presence of both biotic and abiotic stressors (Shelp et al., 2017). Several studies have indicated that the application of exogenous GABA treatment has the potential to enhance the storage quality and prolong the shelf life of various types of fruits (Sheng et al., 2017a, 2017b; Asgarian et al., 2022). Nevertheless, there is a scarcity of information regarding the impact of exogenous GABA treatment on the longevity and quality of kiwifruit.
The objective of this study was to investigate the potential of exogenous GABA application in extending the shelf life of kiwifruit through the inhibition of respiration rate and ethylene release. Our study demonstrates that the utilization of exogenous GABA treatment is a viable strategy for extending the shelf life of kiwifruit. This is achieved by effectively reducing the respiration rate and ethylene release, as this treatment enhances the nutritional composition of postharvest kiwifruit, making it a promising option for commercial applications.
Materials and Methods
Plant materials and treatments
The hardy kiwifruits (Actinidia aruguta ‘Longcheng No.2’) were collected from an orchard located in Dandong city, Liaoning province, China, after they reached mature commercial maturity. The characteristics of the fruits at harvest were green skin color, about 15 N of firmness, and 8 °Brix of soluble solids content. A sample size of 180 fruits was chosen for the experiment. The criteria for the treatment trial included fruits that exhibited consistent size, a smooth surface, and were free from any signs of disease or physical damage. One experimental group consisting of 90 fruits was subjected to immersion in GABA solutions of varying concentrations (0, 5, 10, and 20 mM) for 15 minutes according to our previous GABA soaking fruits, such as kiwifruit (Actinidia chinensis), peach, and zucchini (Shang et al., 2011; Palma et al., 2019; Liu et al., 2023). In contrast, a control group of 90 fruits was immersed in distilled water for the same duration. The samples were subjected to storage conditions in an incubator maintained at a temperature of 20 ± 0.1°C for 20 days, while maintaining a relative humidity of 85–90%. The experiment involved the collection of samples at several time points, specifically at 0, 5, 10, 15 and 20 days following storage. Each time point consisted of three biological replicates that were afterwards analyzed. The measurements of fruit firmness, soluble solids content (SSC), titratable acid (TA), respiration rate, and ethylene release were conducted at each designated sample period. The samples for gene expression analysis, ACO and ACS enzyme activities, and Vitamin C (VC) levels were preserved at an ultralow temperature of ‒80°C for subsequent study.
Determination of SSC, and TA
The SSC was measured using a digital refractometer (Atago, Japan). In the TA assay, a 10 mL sample of kiwifruit flesh juice was subjected to titration using a 0.1 N solution of NaOH until a pH of 8.0 was reached. The outcome of this titration was then expressed as a percentage of tartaric acid (Asgarian et al., 2022).
Measurement of VC and firmness
The measurement of VC was determined according to Liu et al. (2023). Fruit firmness was determined using a TMS-Touch texture analyzer (FTC, Sterling, Virginia, US) with a 7.5 mm diameter probe running at 10 mm s-1, and firmness was expressed in N force (N).
Measurement of ethylene production and respiration rate
Ethylene production was determined using protocol described in a previous study with a GC-14A flame ionization detector gas chromatograph (Shimadzu, Kyoto, Japan) for quantifying the quantity of ethylene (Wang et al., 2022). The rate of ethylene generation was expressed as μL kg-1 h-1. The measurement of respiration rate was conducted using a CO2 infrared gas analyzer (model TEL7001; GE Telaire). The results were expressed in units of mL of CO2 per kg per hour (mL of CO2 kg-1 h-1). Each sample was measured using three biological replicates.
qRT-PCR RNA isolation and transcript analyses
The methodology employed in this study was the utilization of a quantitative reverse transcription polymerase chain reaction (qRT-PCR) technique after the isolation of RNA for subsequent analysis of transcripts. The modified Trizol method was employed for the extraction of total RNA (Li et al., 2021). The synthesis of first-strand cDNA was performed using a Quantitect reverse transcription kit manufactured (Qiagen). The expression level was determined using qRT-PCR with a LightCycler 480 instrument (Roche). The 2−ΔΔCT method was employed for relative gene expression quantification. The primers employed for qRT-PCR are included in Table 1. The expression level was normalized using the internal control Actin (Genbank no. EF063572) (Li et al., 2021).
Extraction and determination of GABA
The extraction of GABA was performed with some modifications from the original protocol (Zhang et al., 2010). The frozen tissue (500 mg) was mechanically pulverized in a solution containing 1.0 mL of 20 mM hydrochloric acid (HCl). The mixture underwent ultrasonic extraction at 80 watts for 15 minutes, followed by centrifugation at 13,000 rpm for 10 minutes. The supernatant was transferred into an Eppendorf tube using a syringe filter with a pore size of 0.2-μm. The detection was conducted using high-performance liquid chromatography (HPLC). The obtained values were expressed in milligrams per gram of fresh weight (mg/g FW).
Phylogenetic analysis
The Molecular Evolutionary Genetics Analysis software (MEGA7; version 7) was utilized to perform phylogenetic analysis. The tree was constructed using the Neighbor-Joining approach. The statistical reliability of the internal branches was evaluated through the implementation of 1000 bootstrap replicates.
Transient overexpression and silencing of AaGAD1 and AaGAD4 in kiwifruit
The full-length cDNA sequences of AaGAD1 and AaGAD4 were cloned into the pRI101-AN by digestion with Kpn1 and BamHI. The 200–300 bp sequences selected of the full-length cDNA sequences of AaGAD1 and AaGAD4 were cloned into the pTRV2 by digestion with EcoR1 and BamHI. The used primers are listed in Table 1. The vectors 35S: AaGAD1 and 35S: AaGAD4, which exhibit overexpression, along with the vectors pTRV2-AaGAD1 and pTRV2-AaGAD4, which induce silencing, were introduced into Agrobacterium tumefaciens GV3101. Two vacant vectors, namely pRI101 and pTRV2, were employed as controls for overexpression and silencing, respectively. In accordance with a previous study, fruit samples that were comparable in size and devoid of any physical damage were utilized for a transient expression test, conducted 7 days prior to harvest (Gan et al., 2019). The experimental procedure was undertaken with each treatment using three biological replicates consisting of 20 fruits each.
Table 1.
Primers used for experimental validation in this study
ACS and ACO activities
The measurement techniques for ACS and ACO have been previously elucidated in prior studies (Wu et al., 2020). The activities of ACS and ACO enzymes were assessed by quantifying the amount of ethylene produced from the substrates. All measurements were conducted using three biological replicates.
Statistical analysis
The experiments employed the completely randomized block design. The statistical analyses were conducted using SAS software (version 8.2, SAS Institute, Cary, NC, USA). The figures were generated using GraphPad Prism 7.0 (GraphPad Software, San Diego, CA, USA). The statistical analyses employed to assess disparities between means involved the utilization of Student’s t-test. The analysis of variance (ANOVA) was employed in conjunction with Duncan’s multiple range test to ascertain the presence of statistically significant variations among treatments.
Results
Effects of GABA treatment on fruit quality during the storage period
The alterations in TSS and TA of kiwifruits treated with GABA and the untreated control throughout the storage duration showed a consistent increase in TSS levels, while TA gradually decreased in both the GABA treatment and control group (Fig. 1A and 1B). The application of a 10 mM GABA treatment resulted in increasing levels of SSC throughout the storage period. Alterations in VC content and firmness of kiwifruits treated with GABA compared to the untreated control throughout the storage period indicates initial increase followed by a subsequent decrease over the course of the storage period (Fig. 1C and 1D). Notably, the application of 10 mM or 20 mM GABA treatment resulted in a significant higher VC accumulation when compared to the control group. A significant decrease in firmness was observed in both GABA-treated and untreated fruits throughout the storage duration (Fig. 1D). The results of the study indicate that the application of GABA to fruits led to a marked increase in firmness. Moreover, the inhibitory effect of exogenous GABA on firmness was more pronounced at a concentration of 10 mM. Thus, these findings indicate that the application of a 10 mM GABA treatment confers advantageous effects on the quality of kiwifruit throughout postharvest storage.
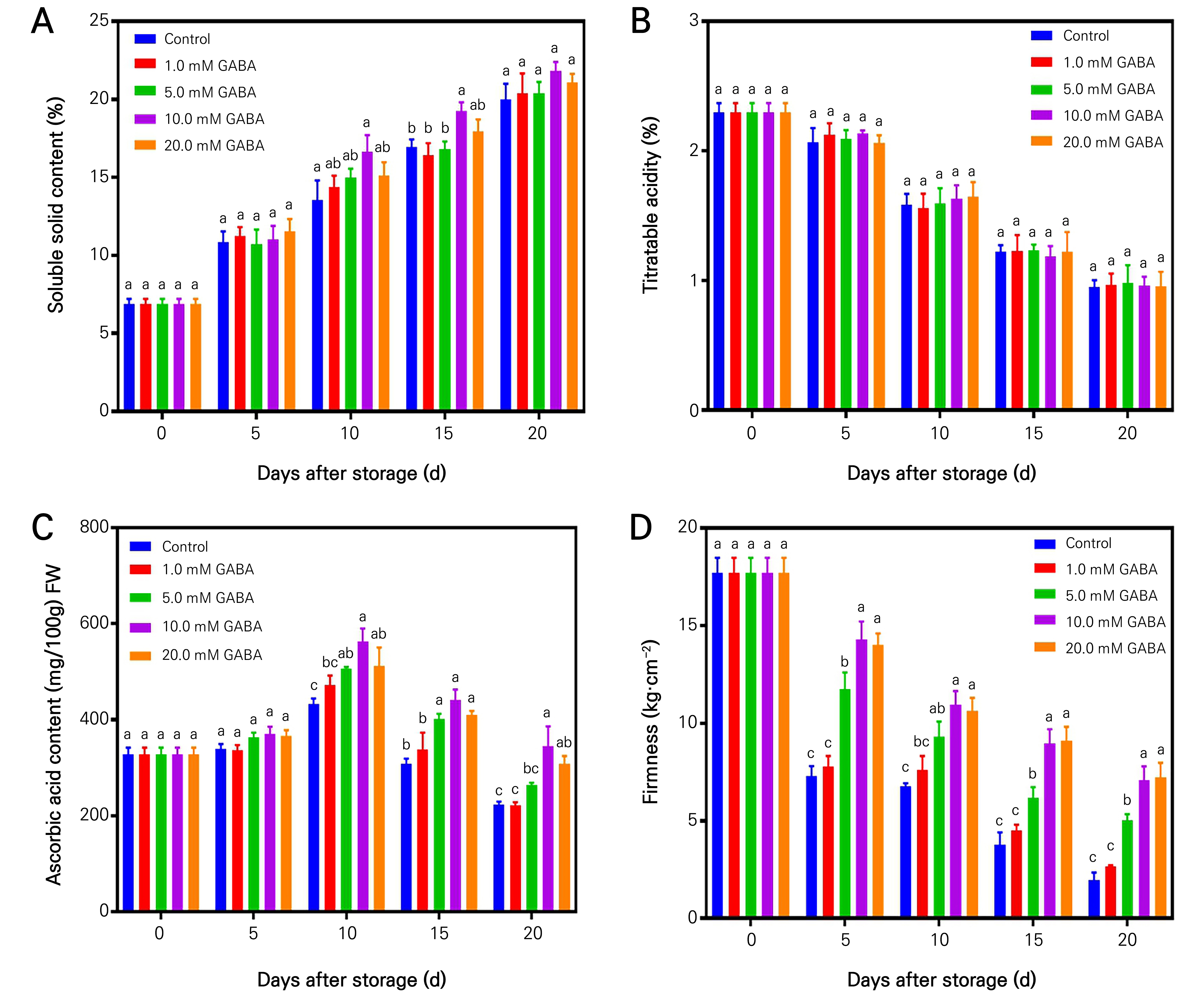
Fig. 1.
Quality changes in hardy kiwifruits treated with different GABA concentrations. Changes in soluble solid content (A), titratable acidity (B), ascorbic acid content (C), and firmness (D) of the control and GABA-treated kiwifruits (at 0, 5.0, 10.0, 15.0 and 20.0 mM) during storage at room temperature. The value measured before treatment was used as the initial value for all treatments at 0 time. Data are the mean ± standard error from three biological replicate assays. Different letters show significant differences (p < 0.05) for each sampling date among treatments.
Effects of GABA treatment on fruit ripening during the storage period
In order to elucidate the involvement of GABA in fruit ripening, an initial step was taken to quantify the levels of naturally occurring GABA throughout the various stages of fruit development. The data demonstrates a gradual decline in endogenous GABA content throughout the developmental process (Fig. 2A). Fruit samples were collected at regular intervals of 20 days, starting from 40 days after full bloom (DAFB) and continuing until 120 DAFB, which corresponds to the day of commercial harvest. Four homologous sequences were discovered, specifically AaGAD1, AaGAD2/5, AaGAD3, and AaGAD4. In order to evaluate the degree of similarity between the four presumed AaGAD amino acid sequences and those of Arabidopsis thaliana, we generated a phylogenetic tree of the GAD family’s amino acid sequences using MEGA 7.0. The findings indicate that the four AaGAD amino acid sequences can be classified into two distinct clades. Within the initial group, AaGAD1, AaGAD3, and AaGAD4 exhibited similarities to AtGAD1. Conversely, in the subsequent group, AaGAD2/5 displayed similarities to both AtGAD2 and AtGAD5 (Fig. 2B). Furthermore, the expression levels of four AaGAD genes, which are responsible for regulating the production of GABA in kiwifruit, exhibited a consistent pattern with the fluctuation of endogenous GABA concentration throughout fruit development. Specifically, these expression levels gradually reduced over time (Fig. 2C). The expression levels of AaGAD1 and AaGAD4 were found to be significantly higher than those of AaGAD2/5 and AaGAD3 throughout the process of fruit development in kiwifruit. Based on this observation, it can be inferred that either the AaGAD1 or AaGAD4 gene plays a crucial role as an enzyme in the production of GABA in kiwifruit. The decrease in endogenous GABA levels seen during the process of fruit growth suggests that GABA may play a role in inhibiting the ripening of fruits.
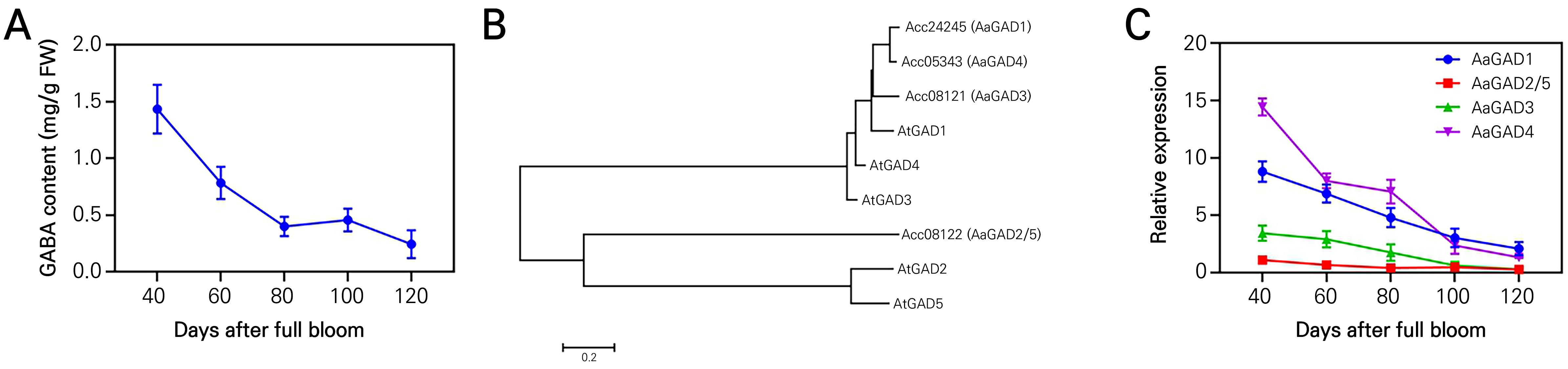
Fig. 2.
Phylogenetic analysis based on the proteins of GABA family members from A. thaliana and A. aruguta, and determination of endogenous GABA content and the relative expression levels of genes which are involved in GABA biosynthesis at days after full bloom in kiwifruit. Determination of endogenous GABA content at days after full bloom in kiwifruits (A). Phylogenetic tree analysis of the proteins of GABA family members from A. thaliana and A. aruguta. The red fonts represent AaGAD1, AaGAD2/5, AaGAD3 and AaGAD4 from A. aruguta, respectively. The tree was constructed by the neighbor-joining method based on the amino acid differences (p - distance) using MEGA 7.0 with complete deletion and 1000 bootstrap replicates (B). Changes in the transcripts of AaGAD1, AaGAD2/5, AaGAD3, and AaGAD4 were determined by qRT-PCR at days after full bloom (C). Data are means of three biological replicates ± SE.
To examine this further, an experiment was conducted including the administration of exogenous GABA to investigate the impact of GABA treatment on ethylene production and respiration rate, which are considered crucial indicators of climacteric fruit ripening during storage duration. Based on the aforementioned findings, a concentration of 10 mM GABA was selected for subsequent investigation. The findings indicated that the ethylene production displayed characteristic climacteric fruit patterns, with the highest levels occurring after 15 days of storage in both the control and GABA-treated fruits (see Fig. 3A). The application of GABA treatment resulted in a marked reduction in ethylene production when compared to untreated control fruits during the storage period (Fig. 3A). In a similar vein, the application of GABA resulted in a significant decrease in the rate of respiration, particularly during the climacteric peaks, which corresponded with the observed impact on ethylene synthesis (see Fig. 3B). The activities of ACO and ACS, which are crucial components in the production of ethylene, were also assessed. It was observed that the application of GABA substantially suppressed ACO and ACS enzyme activities in comparison to the control fruits, throughout the duration of the storage period (Fig. 3C and 3D). Collectively, the findings of this study indicate that the application of exogenous GABA has the capacity to suppress the emission of ethylene, thereby exerting an influence on fruit ripening.
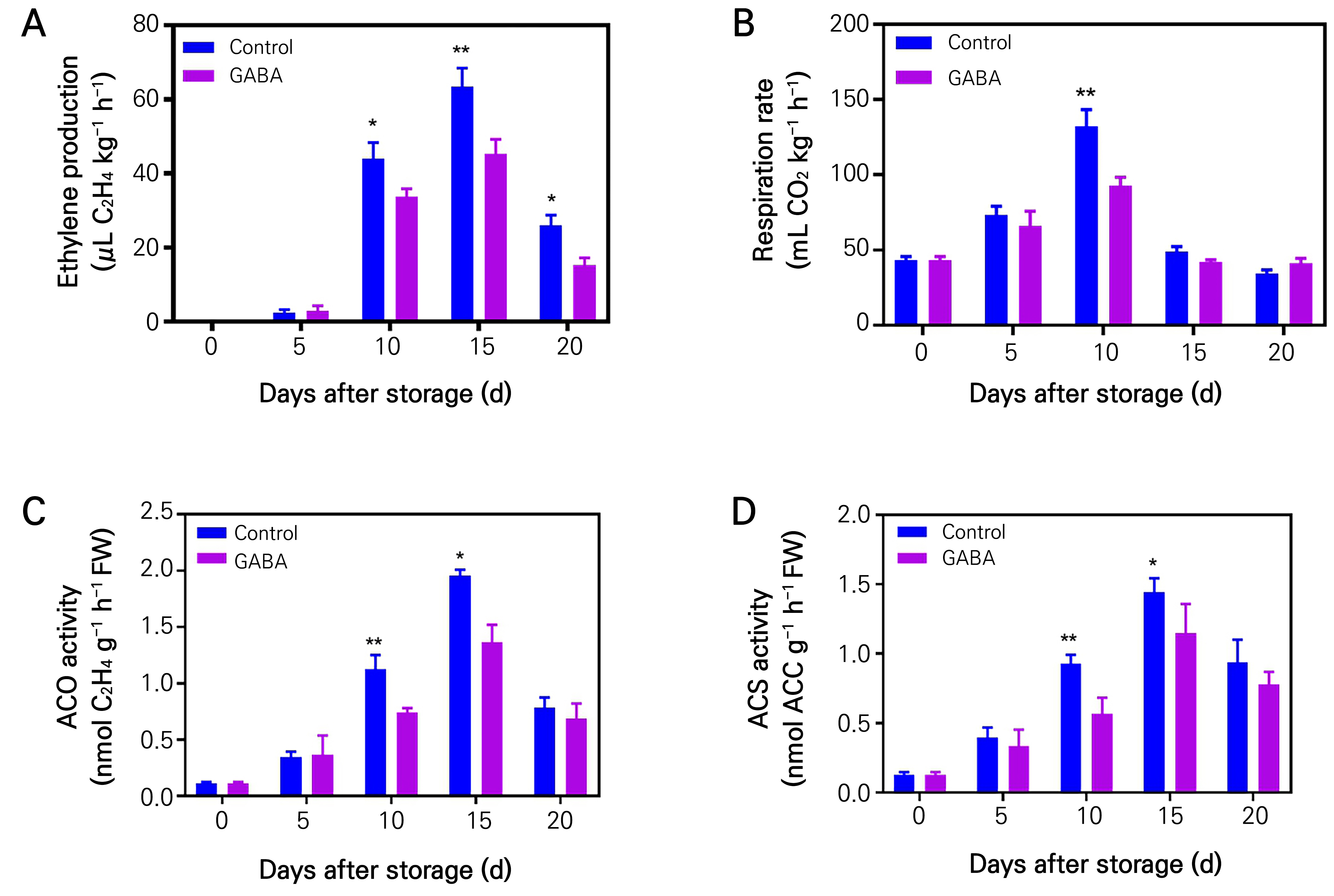
Fig. 3.
Effects of 10 mM GABA treatment on physicochemical characters in kiwifruit. Ethylene production (A), respiration rate (B), ACC oxidase activity (C), and ACC synthase activity (D) of the control and GABA-treated kiwifruits (at 0, 5.0, 10.0, 15.0 and 20.0 mM) during storage at room temperature. Data are means of three biological replicates ± SE. The different lowercase letters indicate significant differences (p < 0.05). Asterisks indicate a significant difference between the treatment and control, according to Duncan’s multiple range tests (*p < 0.05, **p < 0.01).
Ethylene production inhibited by the biosynthesis of endogenous GABA catalyzed via AaGAD1 or AaGAD4
The AaGAD1 and AaGAD4 genes are believed to play a crucial role as essential enzymes in the production of GABA in kiwifruit (Fig. 2C). To study their functions, we cloned both AaGAD1 and AaGAD4, and subsequently produced expression vectors for overexpression and gene silencing experiments. The expression level of AaGAD1 or AaGAD4 was shown to be higher in fruits that underwent overexpression and lower in fruits that underwent gene silencing, as compared to the untreated control, as observed using transient transformation (Fig. 4A and 4B). In a similar manner, the levels of endogenous GABA were shown to be elevated in plants expressing 35S: AaGAD1 or 35S: AaGAD4 constructs, whereas they were reduced in plants expressing pTRV2-AaGAD1 or pTRV2-AaGAD4 constructs, as compared to the control group (Fig. 4C and 4D). In contrast, there was a decrease in ethylene production in the 35S: AaGAD1 or 35S: AaGAD4 lines, while an increase was observed in the pTRV2-AaGAD1 or pTRV2-AaGAD4 lines, as compared to the control (Fig. 4E and 4F).
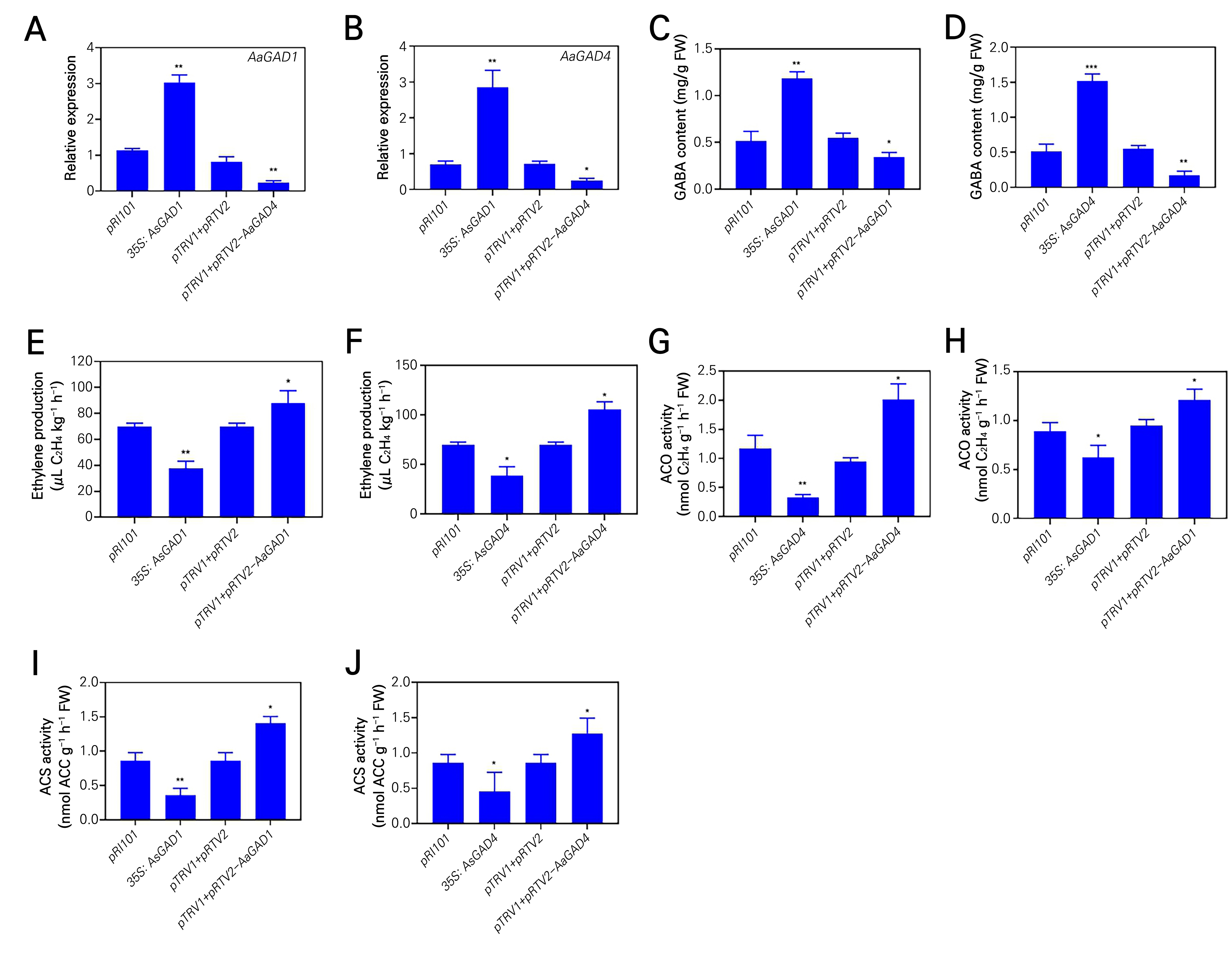
Fig. 4.
Transient overexpression of AaGAD1 and AaGAD4 in kiwifruit. Changes in the transcripts of AaGAD1 (A) or AaGAD4 (B) were determined by qRT-PCR in the AaGAD1 overexpression kiwifruits and silencing kiwifruits or the AaGAD4 overexpression kiwifruits and silencing kiwifruits. GABA content (C and D), Ethylene production (E and F), ACC oxidase activity (G and H) and ACC synthase activity (I and J) in the AaGAD1 overexpression kiwifruits or silencing kiwifruits and the AaGAD4 overexpression kiwifruits or silencing kiwifruits. Data are means of three biological replicates ± SE. Asterisks indicate a significant difference between the treatment and control, according to Duncan’s multiple range tests (*p < 0.05, **p < 0.01).
Additionally, the activities of ACO and ACS were studied, as both enzymes serve as crucial components in the production of ethylene. In contrast to the endogenous GABA, the activities of ACO and ACS were found to be reduced in 35S: AaGAD1 or 35S: AaGAD4 condition, while they were elevated in pTRV2-AaGAD1 or pTRV2-AaGAD4 condition, as compared to the control group (Fig. 4G-4J). In summary, the findings of this study suggest that the AaGAD1 or AaGAD4 gene plays a crucial role in the production of endogenous GABA, which acts as a suppressor of ethylene biosynthesis.
Effects of exogenous GABA treatment on the expression patterns of genes related to ethylene biosynthesis
In order to clarify the internal association between exogenous GABA and ethylene production in fruit ripening, we measured the expression levels of AaCO1 and AaACO3, as well as AaACS1 and AaACS2 (Wu et al., 2020; Gan et al., 2021). As expected, the expression levels of AaACO1 and AaACO3, as well as AaACS1 and AaACS2, were downregulated by the application of GABA during the storage period (Fig. 5A-5D). In contrast, the levels of expression for AaACO1, AaACO3, AaACS1, and AaACS2 were reduced in 35S: AaGAD1 or 35S: AaGAD4 condition, while they were elevated in pTRV2-AaGAD1 or pTRV2-AaGAD4 condition, as compared to the control group (Fig. 5E-5L). The findings of this study indicate that the applicaduction of exogenous GABA plays a role in the suppression of ethylene production, hence preserving the quality of fruits during storage.
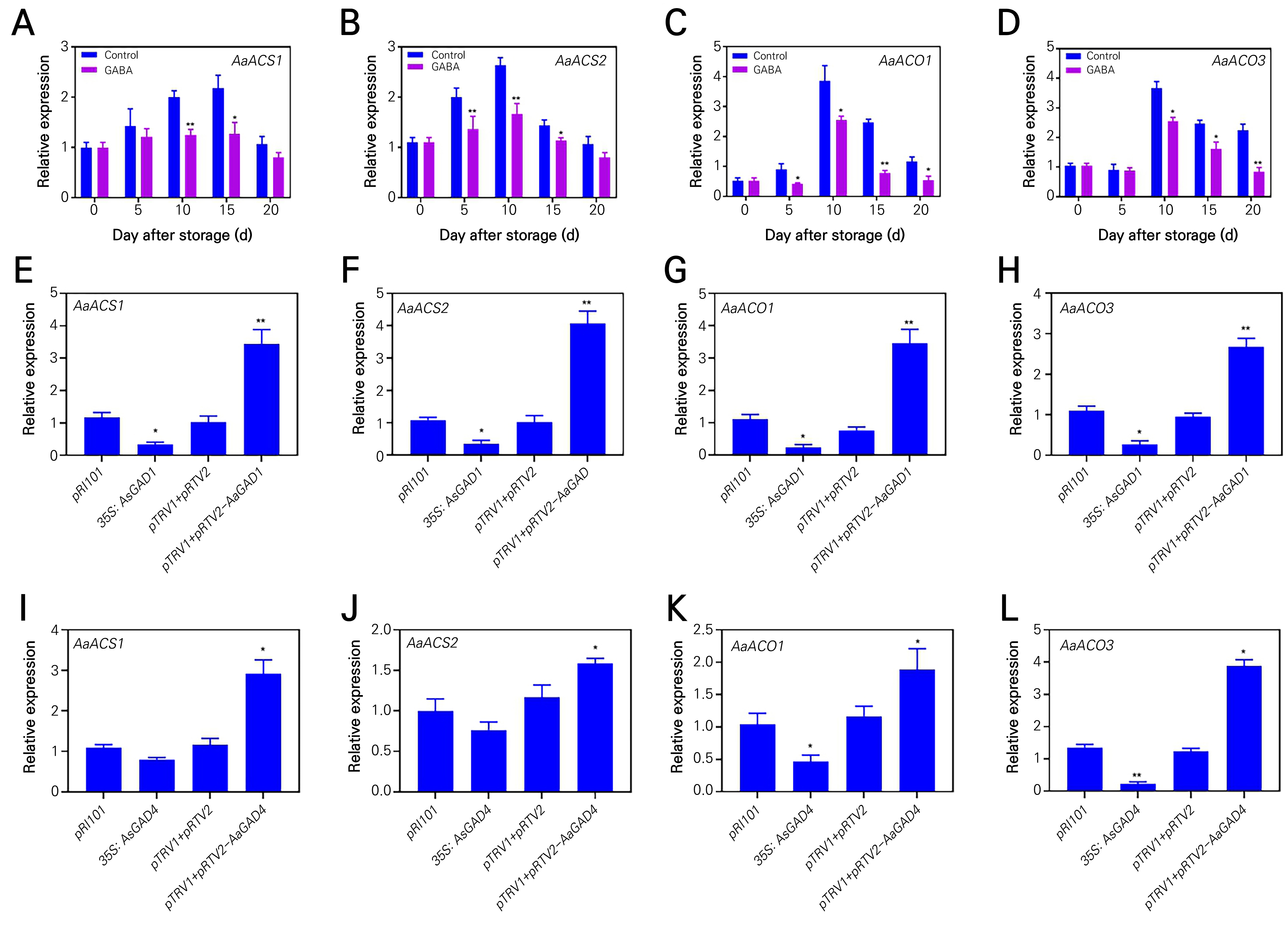
Fig. 5.
The relative expression levels of genes related to ethylene synthesis in the control and GABA-treated kiwifruits at days after full bloom, and transient overexpression of AaGAD1 and AaGAD4 in kiwifruit. Changes in the transcripts of AaACS1 (A), AaACS2 (B), AaACO1 (C) and AaACO3 (D) were determined by qRT-PCR in the control and GABA-treated kiwifruits (at 10.0mM) during storage at room temperature. Relative expression levels of AaACS1 (E), AaACS2 (F), AaACO1 (G), and AaACO3 (H) in the AaGAD1 overexpression kiwifruits or silencing kiwifruits. Relative expression levels of AaACS1 (I), AaACS2 (J), AaACO1 (K), and AaACO3 (L) in the AaGAD4 overexpression kiwifruits or silencing kiwifruits. Data are means of three biological replicates ± SE. Asterisks indicate a significant difference between the treatment and control, according to Duncan’s multiple range tests (*p < 0.05, **p < 0.01).
Discussion and Conclusion
GABA is known to have a significant impact on the growth and development of plants, particularly when it comes to their reaction to various abiotic and biotic stresses. These factors encompass pathogen infection, drought, wound, cold, and heat stress (Khan et al., 2021). Multiple investigations have shown substantial involvement of the GABA pathway in citrate metabolism, and the application of exogenous GABA has been shown to enhance the buildup of citrate content and enhance storage quality (Sheng et al., 2017a).
This study demonstrates that the application of exogenous GABA has a positive impact on enhancing the storage quality of kiwifruit that is characterized by its firmness during postharvest storage. Although there were no significant changes in TAamong the concentrations of GABA (0 mM, 5 mM, 10 mM, and 20 mM) compared to the control during postharvest storage, our findings indicate that treating with exogenous GABA at a concentration of 10 mM significantly increased the level of SSC, increased accumulation of VC, and enhanced reduced fruit firmness compared to the control during postharvest storage (see Fig. 1). Consequently, the application of GABA at a concentration of 10 mM showed a substantial enhancement in the postharvest storage quality of hardy kiwifruit. The application of exogenous GABA markedly improved the storage quality of peach or citrus fruit by increasing the accumulation of proline and the content of citrate, respectively (Sheng et al., 2017b). Furthermore, the application of GABA during the postharvest storage was found to effectively preserve the internal quality of ‘Sahebi’ grape by enhancing the levels of soluble sugars and organic acids (Asgarian et al., 2022). The outcome of our study is consistent with prior studies on citrus, peach, and grape (Sheng et al., 2017a; Asgarian et al., 2022). This suggests that the use of exogenous GABA treatment has the potential to enhance the storage quality and prolong the shelf life of kiwifruit. This can be achieved by increasing the level of SSC, enhancing the accumulation of VC, and mitigating the reduction in firmness.
In general, GABA is a significant metabolic compound within TCA cycle. It is mostly processed through a concise mechanism known as the GABA shunt. In addition to serving as a significant factor in the TCA cycle, our study has demonstrated that the application of exogenous GABA has a substantial impact on the rate of respiration (Fig. 3B). Previous study has indicated that high-acid fruits generally exhibit higher levels of GABA content compared to low-acid fruits (Sheng et al., 2017a). Additionally, it was observed that the respiration rate of high-acid fruits was much lower than that of low-acid fruits during postharvest storage in citrus (Sheng et al., 2017b). In a similar vein, a separate study showed that the application of exogenous GABA had an indirect effect on the respiratory rate, leading to a decrease in the utilization of citrate and crucial amino acids in citrus fruits (Sheng et al., 2017a). The application of exogenous GABA resulted in a notable decrease in the respiration rate as compared to the untreated control in apple (Han et al., 2018). Thus, it was commonly inferred that the presence of exogenous GABA had a negative correlation with the respiration rate in kiwifruit.
In the present study, the application of exogenous GABA exerted inhibitory effects on the rate of respiration. Additionally, a notable decrease in ethylene production was observed following exogenous GABA treatment (Fig. 3A). Furthermore, the activities of ACO and ACS were significantly diminished in the exogenous GABA treatment group compared to the control (Fig. 3C and 3D). The AaGAD1 or AaGAD4 gene has been proved to be a crucial enzyme involved in the production of GABA in kiwifruit (Fig. 2). Again, overexpression of AaGAD1 or AaGAD4 gene inhibited ethylene production (Fig. 4E and 4F) by reducing the activities of ACO and ACS (Fig. 4G-4J), whereas silencing of AaGAD1 or AaGAD4 gene led to the reverse effect (Fig. 4E-4J). In contrast, the overexpression of AaGAD1 or AaGAD4 culminated in reduced expression levels of AaACO1, AaACO3, AaACS1, and AaCS2 (Fig. 5E-5L). Additionally, the silencing of the AaGAD1 or AaGAD4 gene led to an increase in the expression levels of these four genes (Fig. 5E-5L). These findings indicate that GABA has a role in the molecular-level regulation of ethylene production and signal transduction. Nonetheless, the direct correlation between GABA and ethylene synthesis pathways in kiwifruit has yet to be explicitly elucidated, it is important to note that this interaction is not entirely missing in the realm of plant biology. Exogenous application of GABA was found to significantly suppress ethylene production and reduce the relative expression levels of key genes involved in ethylene biosynthesis and signal transduction, namely MdACS, MdACO, and MdERF, in apple during the storage period (Han et al., 2018). In a study on sunflower, it was found that the application of GABA increased ethylene production by upregulating ACS mRNA accumulation, ACC and ACO mRNA levels, and enhanced in vitro ACO activity in both the cotyledons and leaves of the plants (Kathiresan et al., 1997). Likewise, it was established that the application of exogenous GABA in poplar resulted in the activation of key genes associated with ethylene production and abscisic acid (ABA) biosynthesis under salt stress conditions (Ji et al., 2018). Hence, it can be inferred that the interplay between GABA and ethylene biosynthesis exhibits variations across different plant species. Additionally, our findings provide substantial evidence supporting the involvement of GABA in the molecular-level control of ethylene production and signal transduction specifically in kiwifruit.
In summary, our study has illustrated and confirmed earlier findings that: (i) the application of exogenous GABA has the potential to enhance the storage quality and prolong the shelf life of kiwifruit; (ii) there exists an inverse relationship between exogenous GABA and respiration rate in kiwifruit; and (iii) GABA plays a role in the molecular-level regulation of ethylene biosynthesis and signal transduction in kiwifruit. Based on these findings, we have developed a theoretical framework that examines the impact of exogenous GABA treatment on the postharvest quality of kiwifruit (as seen in Fig. 6). Thus, utilization of exogenous GABA treatment presents a viable and secure strategy for extending the shelf life of kiwifruit and augmenting its nutritional content. This postharvest practice holds potential for commercial valorization of kiwifruits.