Introduction
Materials and Methods
Plant Material Culture and Treatment
Measurements of Growth Morphology and Sample Treatment
Determination of AsA and GSH Contents
Measurement of APX and GR Enzyme Activities
Statistical Analysis
Results
Effects of Exogenous Organic Acids on the Growth of C. bipinnatus Under Cadmium Stress
Effects of Exogenous Organic Acids on GSH and AsA in the Roots, Stems, and Leaves of C. bipinnatus Under Cadmium Stress
Effects of Exogenous Organic Acids on the GR and APX activity in the Roots, Stems, and Leaves of C. bipinnatus Under Cadmium Stress
Discussion
Conclusion
Introduction
Heavy metal poisoning of soils is a major issue that has harmful effects on human health and the environment (Wang et al., 2018). Cadmium (Cd) is a very poisonous heavy metal not required for biological development (Wang et al., 2020). High cadmium intake levels cause neurological disorders and cancer (Gao et al., 2022). Cd accumulates in plants after migration into the soil and enters the human body via the food chain, thereby putting human safety at risk (Gao et al., 2022). Plants are also susceptible to the damaging effects of Cd because they lack a functional Cd metabolism mechanism. Cadmium is thus abundant in plants, causing retardation, photosynthesis blockage, and potentially major physiological stressors, such as cell death (Xian et al., 2019).
Several studies postulate that cadmium exerts harmful effects on plants. It is associated with the creation of oxidative stress, including the emergence of reactive oxygen species (ROS) in plant cells and the generation and buildup of enormous levels of reactive oxygen free radicals (O2-, H2O2) (Zhang et al., 2021). Plants have gradually acquired a series of complex physiological systems to withstand adversity stress during the evolution process (Song et al., 2017). For instance, plants have developed various enzymatic and nonenzymatic antioxidant mechanisms, such as ascorbate oxidase (APX), glutathione reductase (GR), ascorbic acid (AsA), and prototype glutathione (GSH) in reaction to stress to remove and balance reactive oxygen species (ROS). Plants' ability to adapt to heavy metal stress is aided by increasing antioxidant resistance (Yang et al., 2020). AsA reacts directly with APX, singlet oxygen, O2-, H2O2, and OH in the AsA-GSH cycle to decrease ROS and oxidative stress in plants. The elimination of ROS radicals is aided by GSH (Ding et al., 2013). The AsA-GSH cycle is thus crucial in the plant's response to Cd stress.
There is a dynamic equilibrium in the generation and clearing of ROS in plant cells under normal environmental circumstances. This equilibrium is disrupted under drought stress, causing an imbalance in ROS generation and metabolism. Biofilm peroxidation, which causes oxidative stress, is caused by ROS-mediated oxidative stress (Wang et al., 2019). Citric acid (CA) has been demonstrated to boost the activity of antioxidant enzymes to scavenge oxygen free radicals. It also efficiently relieves aluminum damage in membrane lipids, which causes growth suppression in Trichosanthes (Chen et al., 2018). The application of CA significantly improves the activities of the major antioxidant enzymes in the AsA-GSH cycle under surface Cd stress conditions. It also boosts the scavenging ability of reactive oxygen free radicals, thereby effectively alleviating the destructive effect of heavy metals on membrane lipids (Chen et al., 2018, Yao et al., 2018). In addition to CA, recent studies suggest that exogenous malic acid (MA) can boost plants' desorption capability for Cd (Tang et al., 2022). Liu also discovered that an optimum concentration of malic acid increases the transportation and enrichment of Cd in S. nigrum by promoting Cd absorption from various plant areas and the ability of Cd to transfer from underground to aboveground (Liu et al., 2020). The addition of malic acid under Cd stress increases the activity of several antioxidant enzymes and gene expression levels, reducing the toxic and oxidative damage caused by Cd by modulating enzyme and non-enzyme antioxidants (Chen et al., 2019). Exogenous MA can reduce H2O2 buildup, increase root activity, and block Cd absorption and transport in the roots, thereby minimizing Cd toxicity.
Cosmos bipinnatus is an Asteraceae herbaceous plant with annual herbaceous blooms. It is drought-resistant and adaptable and can successfully absorb Cd and accumulate more than 1,000 mg·kg-1 of enriched Cd. It is a Cd super accumulator that can accumulate a considerable amount of Cd in the root's cell walls and vacuoles (Liu et al., 2017a). It possesses both cadmium accumulation abilities and excellent aesthetic value compared to other cadmium-accumulation and decorative plants (Liu et al., 2017b) and is thus a good phytoremediation material for Cd-contaminated environments. However, there are limited studies of the impact of exogenous organic acids on Cd stress mitigation and the AsA-GSH cycle in C. bipinnatus to date. This study thus focused on the effects of exogenous organic acids on C. bipinnatus development and the mechanism of the AsA-GSH cycle under Cd stress, which are important for the remediation of soils polluted with heavy metals. This study offers a novel approach to the phytoremediation of polluted soil.
Materials and Methods
Plant Material Culture and Treatment
Healthy and consistent sized Cosmos bipinnatus seeds were selected, and were sterilized with a 2% sodium hypochlorite (NaClO) solution for 20 min, washed thoroughly with distilled water, and incubated in perlite for one week. The uniform seedlings were then transplanted into plastic pots (30 cm × 40 cm × 11 cm, 60 plants per pot) containing 5 L 1/2 (pH 6.0) of Hoagland nutrient solution, which was replaced with total nutrient solution after 14 days. The plants were subsequently grown in a growth chamber set at 25°C, 70% relative humidity, and a 500 µmol·m-2 photon flux density level with a 16-hour light period. Cd was added to the plants (in a CdCl2.2.5H2O solution form) along with organic acids as a root application treatment after three weeks. The concentration of Cd used in this study was 40 µmol/L (Liu et al., 2017b). A total of eight treatments were set: CK (0Cd + 0 mmol·L-1 CA/MA), Cd + 0 mmol·L-1 CA, Cd + 0 mmol·L-1 MA, Cd + (0.5, 1, and 2) mmol·L-1 CA, and Cd + (0.5, 1, and 2) mmol·L-1 MA, each replicated thrice. Plant samples were collected after a week of treatment to determine the contents of AsA and GSH and the enzyme activities of APX and GR.
Measurements of Growth Morphology and Sample Treatment
The fresh C. bipinnatus plant samples collected were washed with UP water. The average height and length of the root system and the five plants in each treatment were measured using a straight ruler. A half gram of stem and leaf samples and 0.2 g of root samples in each group were weighed for subsequent index measurements.
Determination of AsA and GSH Contents
The contents of ascorbic acid (AsA) and glutathione (GSH) were determined through ultraviolet spectrophotometry (Wu et al., 2014). The weighted stems and leaves (0.5 g) and roots (0.2 g) were mixed with a buffer solution in ice and ground to form a homogenate. The homogenate was centrifuged at 12000 rpm for 20 min at 4°C, followed by absorbance measurements of the supernatants at 412 nm and 534 nm. The standard curve of AsA was drawn using the mass as the abscissa and the absorbance as the ordinate, while that of GSH was drawn using the amount of reduced glutathione (GSSG) as the abscissa and the absorbance as the ordinate. AsA and GSH contents in the various samples were calculated based on the standard curve and were expressed as µg·mg-1 and µmol·mg-1, respectively.
Measurement of APX and GR Enzyme Activities
The weighted stems and leaves (0.5 g) and roots (0.2 g) were ground in liquid. Ascorbate peroxidase (APX) activity was measured following a method proposed by Nakano and Asada (Nakano and ASAda, 1981). The zero point is set to the OD value measured at 290nm without adding H2O2. The APX activity was calculated at intervals of 0–30 seconds with readings every 10 s. Glutathione reductase (GR) activity was determined following a method proposed by Halliwell and Foyer (Halliwell and Foyer, 1978). Seting zero was performed accordingly by using the buffer solution instead of the enzyme solution and subsequently measuring the OD at OD 340nm.The GR activity was calculated at intervals of 0–3.5 min and was read every 30 s. APX and GR activities were expressed as µmol·min-1·mg-1.
Statistical Analysis
All data were analyzed using the SPSS 22.0 statistical analysis software. The experiments were completely randomized and were repeated at least thrice. Multiple comparisons of means were conducted via a one-way analysis of variance (ANOVA), and the LSD test was subsequently used to estimate significant differences, with the threshold set to p < 0.05. All images were drawn using Origin 9.1. Data in the figures were expressed as the means ± standard error (SE).
Results
Effects of Exogenous Organic Acids on the Growth of C. bipinnatus Under Cadmium Stress
Cd is one of the most toxic heavy metals and has a serious inhibitory effect on plant growth. Our results (Fig. 1) show the addition of exogenous organic acids under Cd stress, the elongation of the cosmos root system and the accumulation of biomass all have an inhibitory effect. Fig. 1 shows the changes in the plant phenotype, plant height and root length of C. bipinnatus under the different treatments. The plant height and root length of C. bipinnatus under the single Cd treatment were lower than in the control group but were not significantly different (p < 0.05). The addition of CA and MA did not alleviate the inhibited growth of C. bipinnatus caused by Cd toxicity. An increase in the concentration of organic acid led to an increase in the degree of growth inhibition of C. bipinnatus. The exogenous organic acids also did not alleviate the growth of the root and stem caused by Cd toxicity. Medium and high concentrations of CA and high concentrations of MA significantly reduced the root length of C. bipinnatus compared to CK (p < 0.05, Fig. 1C). Similarly, medium and high concentrations of CA and all three concentrations of MA significantly reduced the height of C. bipinnatus (p < 0.05, Fig. 1D).
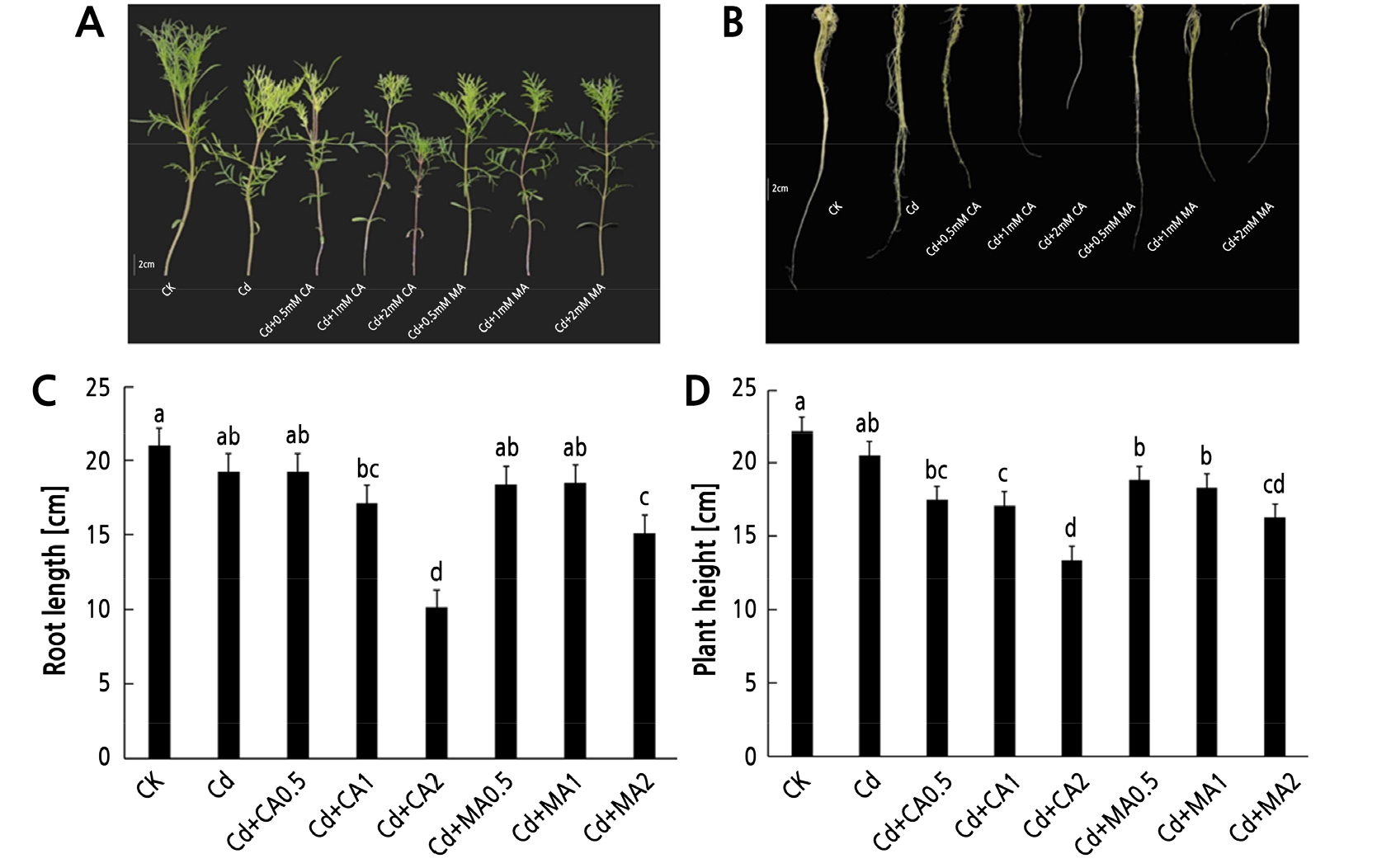
Fig. 1.
Effects of exogenous organic acid on the phenotype (A, B) under Cd stress and effects of an exogenous organic acid on root length (C) and plant height (D) outcomes under Cd stress. Data are expressed as the means ± SE (n = 3). Small letters indicate significant differences among the treatments (p < 0.05).
Effects of Exogenous Organic Acids on GSH and AsA in the Roots, Stems, and Leaves of C. bipinnatus Under Cadmium Stress
Fig. 2 shows the effects of adding organic acids on the GSH content in the roots, stems, and leaves of C. bipinnatus under Cd stress. The GSH content in the roots and leaves of C. bipinnatus under Cd stress increased significantly (Fig. 2A and 2C) compared to CK. However, there were no significant differences in the GSH content in the stem between the control plants and those under Cd stress (p < 0.05, Fig. 2B). Notably, the content of GSH in the roots and stems of C. bipinnatus increased significantly after the addition of CA (Fig. 2A and 2B). The root GSH content increased at low CA concentrations (Fig. 2A). The GSH content in the leaves decreased with an increase in the CA concentration (Fig. 2C). In contrast, the content of GSH in the roots also increased with an increased MA concentration (Fig. 2A). In contrast, the GSH content in the stem and leaves decreased with an increase in the MA concentration (Fig. 2B and 2C).
The AsA content in the roots, stems, and leaves of C. bipinnatus increased significantly under Cd stress (p < 0.05, Fig. 3). Identical outcomes were found for the Cd + MA2 and the Cd + MA1 treatments (Fig. 3A). Notably, the content of AsA in the stems of C. bipinnatus decreased as the concentration of citric acid was increased, and it increased as the concentration of malic acid was increased (Fig. 3B). Similarly, the AsA content of C. bipinnatus leaves was significantly increased under a low CA concentration and in all three MA treatments (Fig. 3C).
Effects of Exogenous Organic Acids on the GR and APX activity in the Roots, Stems, and Leaves of C. bipinnatus Under Cadmium Stress
The GR activity in the roots, stems, and leaves of C. bipinnatus was significantly increased by the Cd treatment (p < 0.05). However, an increase in the concentration of CA led to a decrease in the activity of GR in the roots, stems, and leaves. An increase in the MA concentration also led to a decrease in the GR activity in the roots (Fig. 4A) and leaves (Fig. 4C) of C. bipinnatus but showed an increasing trend in the stems (Fig. 4B). The GR activity in the roots, stems, and leaves generally decreased under medium and high concentrations of organic acids.
Fig. 5 shows the changes in the APX activity in C. bipinnatus under the different treatments. There were no significant differences in the APX activity in the roots of the control C. bipinnatus plants and those under Cd stress (Fig. 5A). However, there were significant increases in APX activity levels in the stems (Fig. 5B) and leaves (Fig. 5C) of C. bipinnatus plants under Cd stress compared to the control plants. An increase in the concentration of CA led to a decrease in the activity of APX in the roots, stems, and leaves. Of note, there was a significant increase in the APX activity in the leaves at low concentrations of CA and MA, with the highest APX activity recorded at 1 mmol·L-1 MA (Fig. 5C).
Discussion
Cd hampers plant growth and development by disrupting the uptake and equilibrium of mineral elements, particularly divalent metal ions (Liu et al., 2021). It replaces certain critical elements in important enzymes and proteins, causing denaturation or lowering enzyme and protein functions (Wang et al., 2021). It also interferes with the physiological systems, including the water balance, antioxidant cycle, and photosynthetic metabolism, limiting the plant's ability to develop (Zhang et al., 2019; Jiang and Huang, 2020). Typical cadmium poisoning signs include reduced plant height, reduced root extension, and leaf yellowing (Xiong et al., 2021). A plant’s height and the length of its roots can directly represent the plant's growth (Rizwan et al., 2019).
Yang discovered that organic acids could stimulate the growth of Solanum nigrum L. under cadmium stress when applied at specific concentrations. However, they can inhibit growth when their limits are exceeded (Yang et al., 2021a). Studies postulate that a high concentration of citric acid inhibits the relief of aluminum toxicity in Pinus massoniana (Yao et al., 2018). In contrast, a low concentration of MA efficiently alleviates the development of Salix variegata under cadmium stress (Chen et al., 2019). In this study, cadmium stress reduced the plant height and root length of C. bipinnatus to varying degrees. These reductions were not alleviated after the application of exogenous organic acids, with the inhibition effect increasing with an increase in the concentration of the organic acid. This phenomenon was attributed to a decrease in cadmium tolerance at higher concentrations of organic acids. A previous study reported that a higher concentration of CA changes the pH and affects the carbonate-bound and total amount of Cd, thus negatively affecting plant function (Lu et al., 2020). We thus inferred that the organic acid concentration was the primary reason for the restrained plant height and root length of C. bipinnatus seedlings. Applying a lower concentration of organic acids may have a significant alleviating effect on the toxicity of C. bipinnatus under cadmium stress.
Plants have formed a series of relatively perfect self-defense mechanisms in the long-term natural evolution process, including enzymatic and non-enzymatic reactions (Zheng et al., 2021). Non-enzymatic components include AsA and GSH, non-enzymatic antioxidants (Yao et al., 2021). AsA and GSH can directly restore ROS and serve as enzyme substrates to remove ROS (Du et al., 2022). Earlier work found that AsA and GSH responded to Cd stress and played an important role in maintaining stable ROS levels in the leaves of C. cathayensis Sarg. seedlings (Luo and Wang, 2019). Clearly, AsA and GSH are important antioxidant substances involved in scavenging reactive oxygen species produced by oxygen metabolism and environmental stress in plants (Wang et al., 2014, Yang et al., 2021b).
GSH contains sulfenyl substances (-SH) and thus forms chelates with Cd2+, thereby reducing the concentration of free Cd2+ in the cytoplasm, which is then transported to the extracellular cells or the vacuole region (Zhou et al., 2022). In this study, the contents of GSH in the roots and leaves of C. bipinnatus under a Cd treatment increased more than those in the CK group. This finding indicated that Cd stress did not damage the endogenous synthesis of GSH but rather promoted the antioxidant system in the plant to maintain a dynamic balance of normal physiological metabolism of the plant. The addition of three organic acids significantly increased the GSH content in the roots of S.variegata under Cd stress, indicating that this species could sufficiently produce and supplement GSH for antioxidant consumption in the presence of organic acids (Zhang et al., 2020). In this study, the GSH content in the roots and stems increased relative to Cd after exogenous citric acid and malic acid were added. However, there was a decrease in the GSH content in the leaves (Fig. 2C), which was attributed to the inhibition of GSH synthesis, reduced GR activity, and damage to the antioxidant system. The reduction of GSSG is affected, ultimately leading to a decrease in the GSH content. These findings collectively suggest that higher citric acid and malic acid concentrations (1–2 mmol·L-1) reduce the antioxidant response of C. bipinnatus.
AsA is involved in various adverse resistance reactions in plants (Meng et al., 2018). Many studies postulate that applying exogenous substances increases the AsA content. For example, applying chitosan can reduce the ROS content in the leaves of maize seedlings under cadmium stress, thereby promoting the regeneration of AsA and increasing its content significantly (Qu et al., 2018). The application of malic acid maintained the ASA content in C. bipinnatus stems and leaves at a high level (Fig. 3B and 3C). The plants potentially produced more active oxygen after applying exogenous malic acid, which further increased the AsA content. The AsA content decreased in the stems and leaves after the application of citric acid, possibly because the AsA-GSH cycle was blocked, leading to a decrease in the AsA content in the stems and leaves of C. bipinnatus owing to the decrease in GSH. However, the AsA content in the roots of C. bipinnatus increase after the application of citric acid and malic acid (Fig. 3A), possibly due to the transportation of Cd and other environmental factors. Root stress is relieved, and the AsA-GSH system is promoted when Cd is transported to the upper part of the ground (Ge et al., 2020), leading to the regeneration of AsA. Nonetheless, the specific causes of this phenomenon should be studied further.
APX and GR are important antioxidant enzymes for scavenging reactive oxygen species in plants (Gao et al., 2021). H2O2 and O2- in plants accumulate in large quantities under stress owing to the destruction of the reactive oxygen scavenging system, thereby activating the stress response of plants (Zhao et al., 2021). Previous studies postulate that cadmium stress decreases plant-growth-related attributes but enhances the activities of APX and GR (Ck et al., 2020). In this study, the activities of GR and APX in C. bipinnatus under a citric acid treatment showed a decreasing trend when the concentration was 1–2 mmol·L-1. This decrease was even lower than that of a sole Cd treatment. This finding was consistent with the outcome of adding exogenous citric acid, which causes the ascorbate peroxidase (APX) activity to rise first and then decrease under aluminum stress (Yao et al., 2018). This phenomenon was attributed to the relatively high concentration of citric acid, which increased the toxicity in the plants and damaged the scavenging system of reactive oxygen species (Xiang et al., 2016). The high concentration of citric acid may have also caused a sharp decline in the plant environmental pH, leading to a decrease in the activities of corresponding enzymes in tissues (Li et al., 2011). The GR activity in the C.bipinnatus roots increased and then decreased below the CK level (Fig. 4A). Along the same lines, the APX activity in C.bipinnatus stems and leaves increased significantly under high and low concentrations of malic acid (Fig. 5B and 5C), indicating that an optimum concentration of malic acid can regulate antioxidant enzyme activity and maintain the balance of ROS, thus regulating its coercion and defense systems (Qi et al., 2021). Similar results have been reported in grapes [39]. Treatment of grapes with a low concentration of organic acid increases antioxidant enzyme activity, facilitating the removal of H2O2 and maintaining the stability of cell membranes (Sun et al., 2015). Xian et al. confirmed that cosmos can be treated with a low concentration of MeJA under Cd stress to increase the antioxidant enzyme activity in the leaves, thereby improving plant resistance (Xian, 2019). Similarly, there was an increase in the activities of GR and APX enzymes in each tissue of C. bipinnatus.
Conclusion
The AsA-GSH cycle is crucial in a plant's response to Cd stress. In this study, low concentrations of exogenous organic acids positively affected the growth and efficiency of AsA-GSH of C. bipinnatus treated with 40 µmol·L-1 Cd. However, the positive effect decreased with an increase in the concentration of organic acids. Cd causes oxidative stress in plants, producing ROS to poison the plant. Exogenous organic can sufficiently produce and supplement GR and APX to facilitate the generation of AsA and GSH to promote the efficiency of the removal of oxidative damage caused by Cd. Moreover, exogenous organic acids can regulate the activities of enzymes involved in ASA-GSH cycle metabolism (APX, GR) to reduce the oxidative damage of ROS accumulation in cells, as induced by cadmium stress.