Introduction
Materials and Methods
Plant material and treatments
Determination of the storage duration
Measurement of the RFW and RFD
Water absorption, water loss and water balance measurements
Measurement of the leaf malondialdehyde (MDA) content and lipoxidase (LOX) activity
Determination of antioxidant enzyme activity in chrysanthemum leaves
Measurement of the proline (Pro) content in chrysanthemum leaves and petals
Statistical analysis
Results
Effects of MT on the flower storage duration
Effects of MT on the RFW and RFD of cut chrysanthemum flowers
Effects of MT on the water absorption, water loss, and water balance of cut chrysanthemum flowers
Effects of MT on the leaf malondialdehyde content and lipoxidase activity of cut chrysanthemum flowers
Effects of MT on leaf antioxidant enzyme activity in chrysanthemums
Effects of MT on the Pro content in the leaves and petals of cut chrysanthemum flowers
Discussion
Conclusion
Introduction
The high market value of cut flowers has driven the development of the global flower industry, which has created numerous job opportunities and economic prospects, especially in developing countries (Chandler and Brugliera, 2011). Cut flowers such as chrysanthemums (Chrysanthemum spp.), roses (Rosa spp.), carnations (Dianthus caryophyllus), and gypsophila (Gypsophila spp.) account for a large proportion of the global floricultural trade. Chrysanthemum (Chrysanthemum morifolium R.), which originated in China, is one of the most important floricultural crops worldwide with a high economic and ornamental value. It is used as cut flowers and ornamental plants (Teixeira da Silva, 2003). Chrysanthemum is an ethylene insensitive plant where lipid peroxidation and membrane damage are involved in flower deterioration (Bartoli et al., 1995). Research to identify treatments that enhance the shelf life of chrysanthemum cut flowers is necessary and urgent. Petal and leaf senescence primarily affect the quality of cut flowers. The decline in the quality of cut chrysanthemums is mainly due to their leaves wilting due to impeded water transport. This loss of turgidity accompanied by chlorophyll degradation results in the leaves senescing earlier than the inflorescences (Petridou et al., 2001). During aging, oxidative stress created by the production of reactive oxygen species (ROS) induces premature senescence in cut flowers. Nucleic acids (DNA/RNA), pigment molecules, membranes, and proteins are damaged by ROS (Wei et al., 2021). Recently, many studies have shown that many compounds have been identified for their potential antioxidant properties by eliminating ROS. Among these compounds are salicylic acid (Shabanian et al., 2019), volatile oils (Langroudi et al., 2020) and moringa extract (Hassan et al., 2020) as well as melatonin (Nawaz et al., 2016), all of which have been described as ROS scavengers capable of delaying the senescence process and extending the vase life.
Melatonin (N-acetyl-5-methoxytryptamine, MT) is an indolic compound widely distributed in animals and plants. MT has strong antioxidative properties and the capacity to scavenge ROS, and it plays a key role in plant stress resistance (Zhang et al., 2015). The physiological response of higher plants to MT has garnered interest in the last few years. MT can increase the activity of enzymes involved in ROS metabolism, such as superoxide dismutase (SOD), peroxidase (POD), ascorbate peroxidase (APX), peroxidase (POD), and catalase (CAT), thereby reducing the negative impact of ROS (Arnao and Hernández-Ruiz, 2014). Numerous studies have reported that MT possesses strong antioxidant activity, and MT has been used to prolong the storage duration of many fruits and vegetables. For example, 100 µM of MT was used to extend the storage time of sweet cherries and 50 µM of melatonin was used to extend the shelf life of fresh-cut broccoli to 14 days (Sharafi et al., 2021; Giraldo-Acosta et al., 2023). Previous studies have investigated the effect of MT on plant senescence under oxidative stress. Ding et al. (2018) report the role of exogenous melatonin in relieving oxidative stress induced by methyl viologen (MV) in poplar leaves. Wang et al. (2020) reported that the application of melatonin via leaf spraying attenuated cold-induced oxidative stress and promoted photosynthetic carbon fixation in tomato plants. Nevertheless, few studies have focused on the effect of MT on the storage duration and senescence of cut flowers. Aghdam et al. (2019) reported that MT enhances the frost resistance of anthurium cut flowers during low-temperature storage. Lezoul et al. (2022) reported the effects of different MT concentrations on the storage duration of cut carnations. However, knowledge concerning the effect of MT on the quality maintenance of cut chrysanthemums remains limited.
Considering the significant economic value of cut chrysanthemum flowers and their limited storage duration, it is imperative to develop effective treatments that reduce the rate of quality degradation and prolong their postharvest longevity. This will help ensure that these popular flowers remain fresh and aesthetically pleasing for an extended period, thereby meeting consumer demand levels and contributing to the sustainability of the floriculture industry. Presently, there is limited information available in the published literature concerning the relationship between MT and the senescence of cut flowers. The present study aimed to assess the effects of different concentrations of MT on the storage duration, water balance, and on various aspects of senescence occurring in cut chrysanthemum flowers, such as changes in the relative fresh weight (RFW), relative flower diameter (RFD), malondialdehyde (MDA) content, free proline (Pro) content, and antioxidant enzyme activity.
Materials and Methods
Plant material and treatments
Cut chrysanthemum flowers (Chrysanthemum morifolium R.) were bought from a floral market in Dongchangfu District, Liaocheng City, Shandong Province. The selected cut chrysanthemum flowers were in full bloom. MT (purchased from Sigma Chemical Co. Ltd.) was dissolved with 0.5mL of ethanol and diluted to each concentration with distilled water. Each flower was subjected to pruning, which involved cutting the stem along the base of the branch at a certain length, and damaged leaves were removed. The processed cut chrysanthemum flowers were put in graduated cylinders containing MT solutions at the following concentrations: 0 (Control), 1, 5, 20 and 50 µM. Twenty flowers were allocated to each experimental group. The chrysanthemums were kept in a biochemical incubator at 20°C ± 1°C, with relative humidity of 90%, and a 12 h photoperiod using white fluorescent light. The MT solutions at the respective concentrations were added to the graduated cylinders as necessary every day (while adjusting the volume to 250 ml). The storage duration, fresh weight (FW), and floral diameter were determined every two days for each treatment group (three replicates per group) to establish the optimum concentration of MT. Leaves and petals of cut flowers treated with the optimal MT concentration were removed every two days, frozen in liquid nitrogen, and stored at −80°C until further analysis to determine their physiological and biochemical parameters. All assays were performed in triplicate, and each experiment was repeated twice.
Determination of the storage duration
The storage duration was determined as the duration between the day when the flowers were placed in the solution and the day when they lost their ornamental value, indicating the end of their storage duration (Gerasopoulos and Chenbli, 1999). The flowers were considered to have reached the end of their storage duration when one or more of the following senescence symptoms were detected in at flowers: bending of the pedicle and wilting or dropping petals.
Measurement of the RFW and RFD
The fresh weight and flower diameter of each flower were expressed as a percentage of the initial fresh weigh and flower diameter, which was assumed to be 100%. Flower weight was recorded every two days. The following formula was used to measure the RFW:
RFW (% of initial FW) = Wt / Wt=0 × 100,
where Wt is the weight of a stem (g) at day t (t = 0, 2, 4, etc.) and Wt=0 is the weight (g) of the same stem at day 0.
The flower diameter (in mm) was measured using Vernier calipers every two days, and data were expressed in mm (Liu et al., 2018). The RFD was calculated as follows:
RFD (% of initial FD) = FDt / FDt=0 × 100,
where FDt is the flower diameter (mm) at day t (t = 0, 2, 4, etc.) and FDt=0 is the flower diameter (mm) at day 0.
Water absorption, water loss and water balance measurements
The water absorption, water loss and water balance were determined using an analytical balance and recorded every two days. They were calculated as follows:
A = weight of cylinder (g) + weight of solution (g) + flower weight (g)
B = weight of cylinder (g) + weight of solution (g)
The water absorption (g) was the difference between two measured A values.
The water loss was the difference (g) between two measured B values.
The water balance (g) = the water absorption (g) - the water loss (g)
Measurement of the leaf malondialdehyde (MDA) content and lipoxidase (LOX) activity
The MDA content was measured through a thiobarbituric acid (TBA) analysis according to a method described by Zeng et al. (2023). Leaf samples (1 g) were homogenized in 5 mL of 100 g/L trichloroacetic acid (TCA) and centrifuged at 10000 × g for 10 min. The supernatant (2 mL) was mixed with 2 mL of 0.67% TBA. The mixture was placed in a water bath cauldron and boiled in a hot water bath for 30 min. The absorbance values of the reaction mixture at 532, 600, and 450 nm were measured using a 722 spectrophotometer (Shanghai Precision Scientific Instrument Co., Ltd., Shanghai, China).
Lipoxidase (LOX) activity was assessed using a method described by Aghdam et al. (2016). To assess the LOX activity, leaf samples (1 g) were homogenized in 10 mL of a 100 mM phosphate buffer (pH 8) supplemented with 2% polyvinylpyrrolidone. The homogenate was centrifuged at 12000 × g for 30 min at 4°C. A reaction mixture containing 0.5 mL of supernatant, 2.4 mL of a phosphate buffer (100 mM, pH 6.8), and 0.1 mL of 10 mM sodium linoleic acid was used to assess the LOX activity (in mkat kg−1 FW).
Determination of antioxidant enzyme activity in chrysanthemum leaves
Leaf samples (1 g) were homogenized in 5 mL of a 50 mM phosphate buffer (pH 7.8) using a mortar and pestle. The homogenate was centrifuged at 10000 × g for 30 min, and the supernatant was used in the enzyme assays. The activity of the antioxidant enzymes SOD, APX, and CAT was assessed using a method described by Qi et al. (2014) with certain modifications.
SOD activity was measured in a reaction mixture containing a 0.05 M phosphate buffer (pH 7.8), 0.1 mM ethylene diamine tetraacetic acid, 130 mM methionine, and 0.75 mM nitro-blue tetrazolium. To initiate the biochemical assay, 0.05 mL of the leaf extract supernatant was added to 3 mL of the pre-prepared reaction mixture. The reaction was then triggered by adding 0.5 mL of a 0.02 mM riboflavin solution, followed by exposure to fluorescent light (4,000 lux) for 20 min. The complete reaction mixture without the enzyme extract was used as a control. SOD activity was determined by measuring the absorbance value at 560 nm.
To measure POD activity, the leaf extract supernatant was mixed with a reaction solution containing 1 mL of guaiacol, 1 mL of H2O2, and 0.1 mL of crude enzyme. The addition of the enzyme extract triggered the reaction, and absorbance was measured at 470 nm.
CAT activity was determined using an ultraviolet spectrophotometer. The enzyme reaction (consisting of a 50 mM phosphate buffer (pH 7) and 0.75% H2O2) was started by adding 0.1 mL of the enzyme extract to the reaction mixture. The absorbance value at 240 nm was recorded every 30 s for 3 min.
Measurement of the proline (Pro) content in chrysanthemum leaves and petals
The Pro content was determined as described by Hassan and Ali (2014) with minor modifications. Samples of petals (2 g) and leaves (2 g) were homogenized with 10 mL of 3% sulfosalicylic acid at 4°C, and the filtrate was boiled at 100°C for 10 min in a water bath and centrifuged at 10000 × g for 15 min. The supernatant (2 mL) was mixed with 2 mL of ninhydrin reagent and 2 mL of glacial acetic acid. The above mixture was boiled in a water bath for 30 min. After the mixture had cooled, 5 mL of toluene was added and mixed immediately. The absorbance value was measured at 520 nm.
Statistical analysis
The experiments were performed using a randomized complete block design with three replications. Statistical analysis of the experimental data was performed using SPSS 16.0 (SPSS Inc., Chicago, IL, USA). Comparison of means was performed via an analysis of variance, and the intergroup means were compared using Duncan’s multiple range tests (p < 0.05).
Results
Effects of MT on the flower storage duration
Storage duration is an important indicator of the degree of senescence of cut flowers. The various concentrations of MT had different effects on the storage durations of the cut flowers, as shown in Fig. 1. Compared to the control group, the 5 µM MT treatment group has a longer storage duration of cut chrysanthemum flowers by 31.78%. There was no significant difference in the storage duration between the control group and the groups treated with 1 and 20 µM MT. Nevertheless, the group treated with the 50 µM MT solution showed a significantly reduced storage duration of cut chrysanthemum flowers, indicating an adverse effect. Conversely, the treatment with 5 µM MT markedly enhanced the storage duration, indicating a positive effect on the longevity of cut flowers.
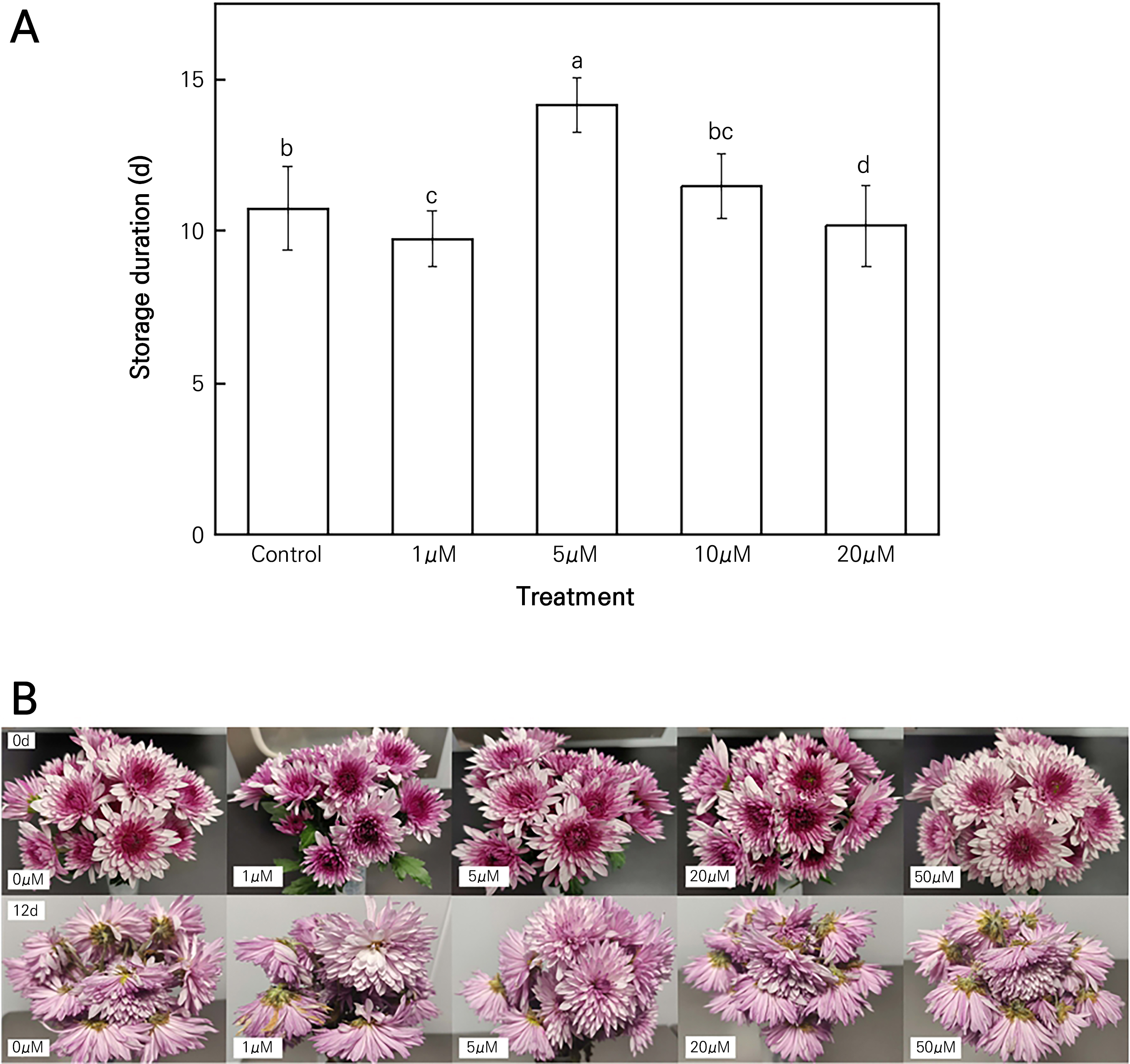
Fig. 1.
Effects of the MT treatment on the storage duration (A) and visual appearance (B) of cut chrysanthemum flowers. Values are presented as the arithmetic mean. The error bars represent the experimental standard errors of the means. Different letters indicate significant differences at p < 0.05 based on Duncan’s multiple range tests.
Effects of MT on the RFW and RFD of cut chrysanthemum flowers
The flower diameter and fresh weight of cut flowers characterize to some extent the degree of aging and the ornamental value of cut flowers. During storage, the RFW of the cut chrysanthemum flowers initially increased and then decreased (Fig. 2A). The most notable increase in the RFW was recorded in the 5 µM MT treatment group, which showed a 10.31% higher RFW than the untreated control group on day 10. In contrast, on day 10, the RFW in the 50 µM MT group was 14.31% lower than that in the control group. The RFD showed a trend of an initial increase and a subsequent decrease thereafter (Fig. 2B). During the first four days, there were few differences in the RFD outcomes among the treatment groups. The RFD levels in the 5 µM MT group were 10.9%, 11.98%, and 18.17% higher than those in the control group on days 6, 8, and 10, respectively. The RFD in the 1 µM MT treatment group did not differ significantly from that in the control group. During days 4 to 10 of storage, the RFD outcomes in the 20 and 50 µM MT groups were lower than that of the control group. These results suggest that exogenous 5 µM MT significantly increased the RFW and RFD, whereas higher concentrations of MT significantly reduced the RFW and RFD. The results thus demonstrate 5 µM MT gave the best results in prolonging the storage duration of cut flowers, increasing the RFW and RFD.
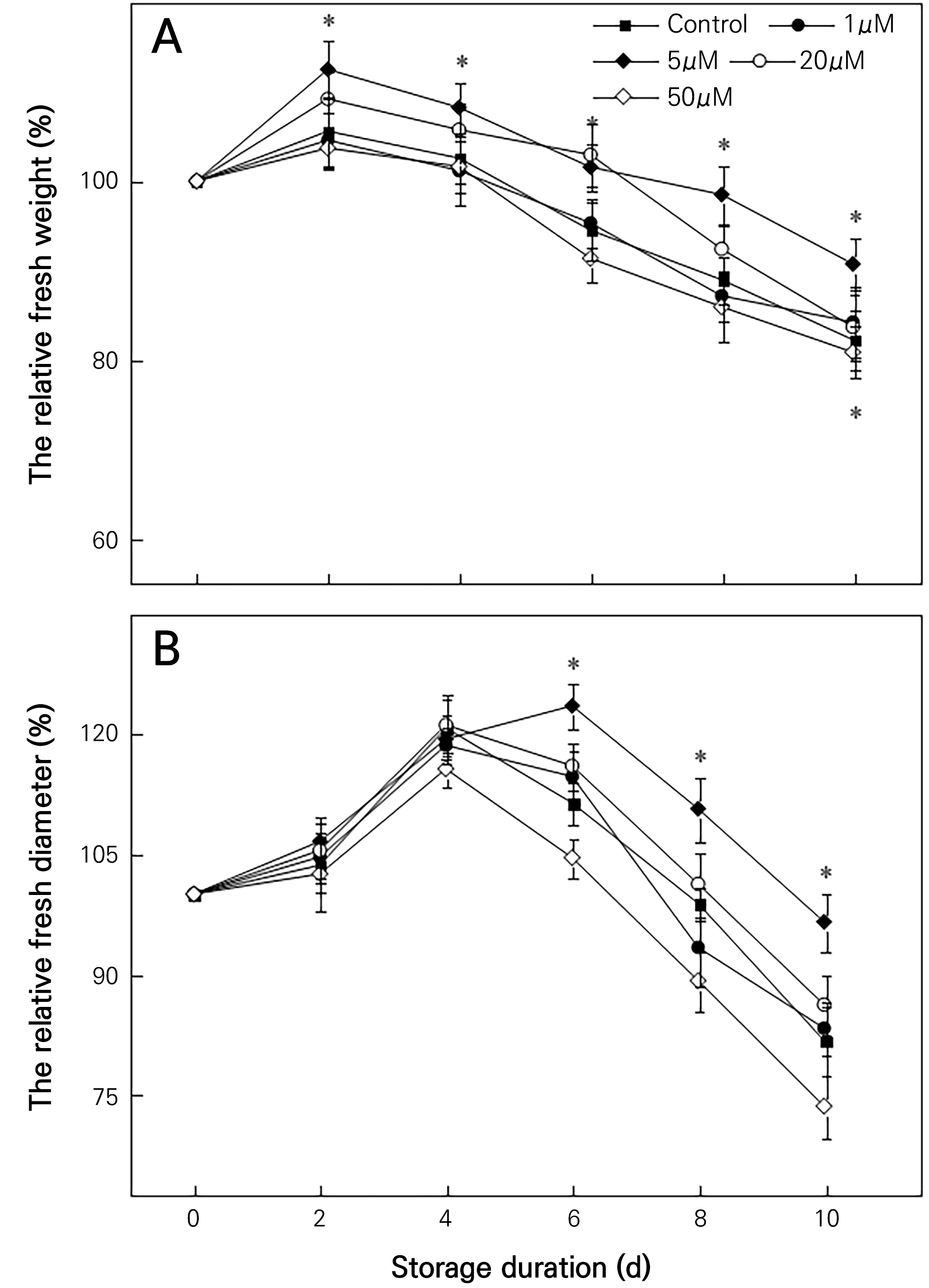
Fig. 2.
Effects of MT on the relative fresh weight (RFW) and relative flower diameter (RFD) of cut chrysanthemum flowers. Values are presented as the arithmetic mean. The error bars represent the experimental standard errors of the means. Values with an asterisk (*) indicate MT-treated cut chrysanthemum flowers showing significant differences from the control at p < 0.05.
Effects of MT on the water absorption, water loss, and water balance of cut chrysanthemum flowers
The longevity of cut flowers depends on the balance between water absorption and water loss. Water absorption in the cut chrysanthemum flowers here initially increased and then decreased both in the control group and in the 5 µM MT group (Fig. 3A). Water absorption in the 5 µM MT group differed slightly from that in the control group. Water loss showed a general trend of an initial increase and a subsequent decrease, after which it increased sharply on day 8 in the control group (Fig. 3B). The water loss in the control group was higher than that in the MT group. Compared with the control, the 5 µM MT group exhibited 48.63% and 55.76% reductions in water loss on days 6 and 10, respectively. Upon increasing the storage time, the water balance initially increased and subsequently decreased (Fig. 3C). The water balance in the 5 µM MT group was higher than the corresponding values of the control groups on days 4 and 10, at approximately 102.22% and 61.70% higher than those of the control, respectively. A similar trend was observed on day 6. Therefore, the treatment with 5 µM MT significantly reduced water loss and helped to maintain the water balance in the cut chrysanthemum flowers.
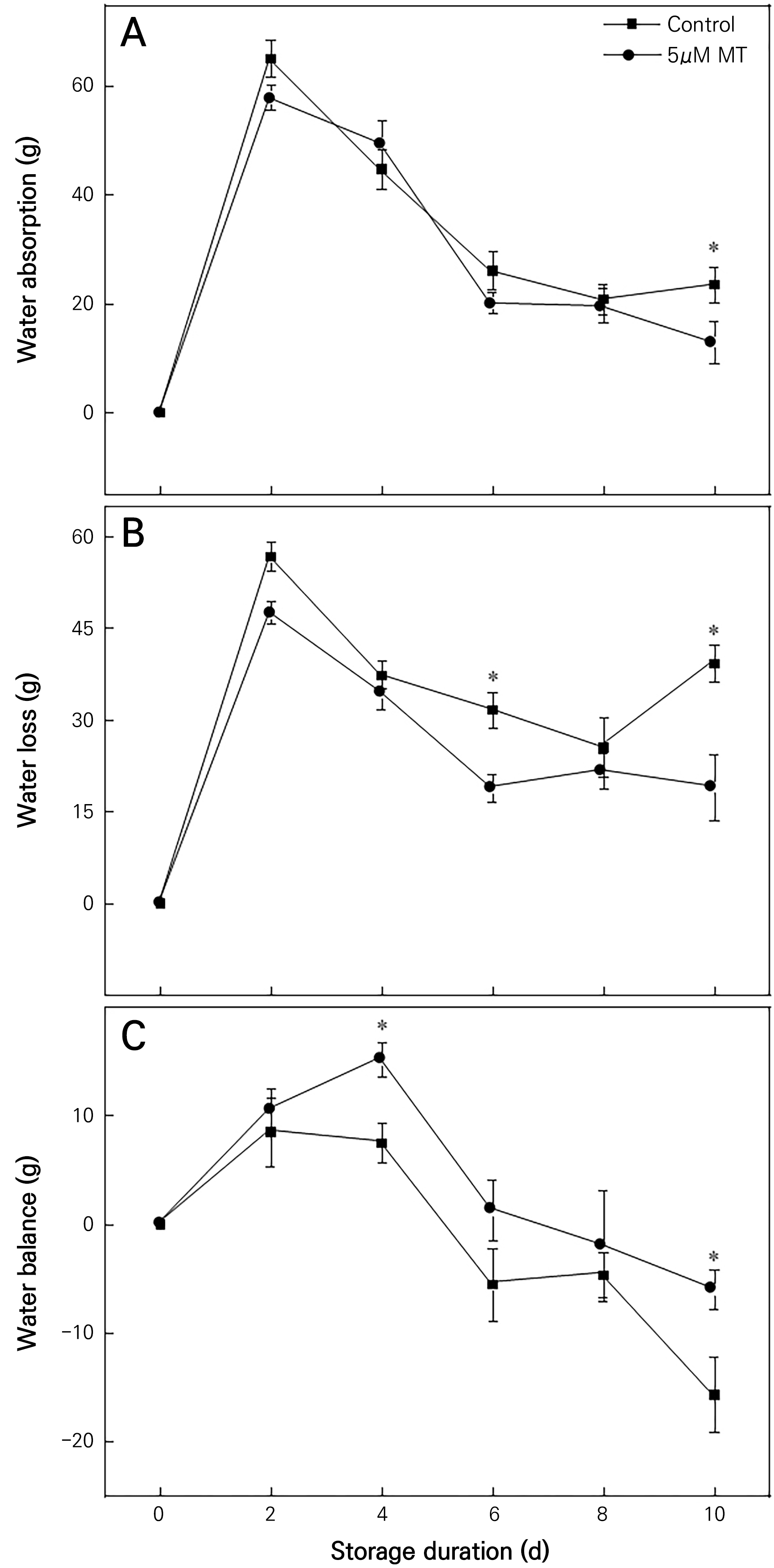
Fig. 3.
Effects of 5 µM MT on water absorption, water loss and water balance of cut chrysanthemum flowers. Values are presented as the arithmetic mean. The error bars represent the experimental standard errors of the means. Values with an asterisk (*) indicate MT-treated cut chrysanthemum flowers showing significant differences from the control at p < 0.05.
Effects of MT on the leaf malondialdehyde content and lipoxidase activity of cut chrysanthemum flowers
MDA is one of the indicators characterizing the degree of plant senescence. As shown in Fig. 4A, there was a gradual increase in the leaf MDA content. From day 0 to day 2, the MDA content did not differ significantly between the treatment groups and the control group. However, from day 4 to day 10, the MDA content was significantly higher in the control group than in the MT treatment groups. At the end of the storage period, the leaf MDA content in the 5 µM MT treatment group was 18.72% lower than that in the control. LOX activity exhibited an upward trend over the course of storage (Fig. 4B). Notably, LOX activity was significantly lower in the MT groups than that in the control group. LOX activity levels in the 5 µM MT group were approximately 35.99% and 38.87% lower than the corresponding values in the control group on days 8 and 10, respectively. These results (Fig. 4B) indicate that the increase in the LOX activity was effectively inhibited by 5 µM MT.
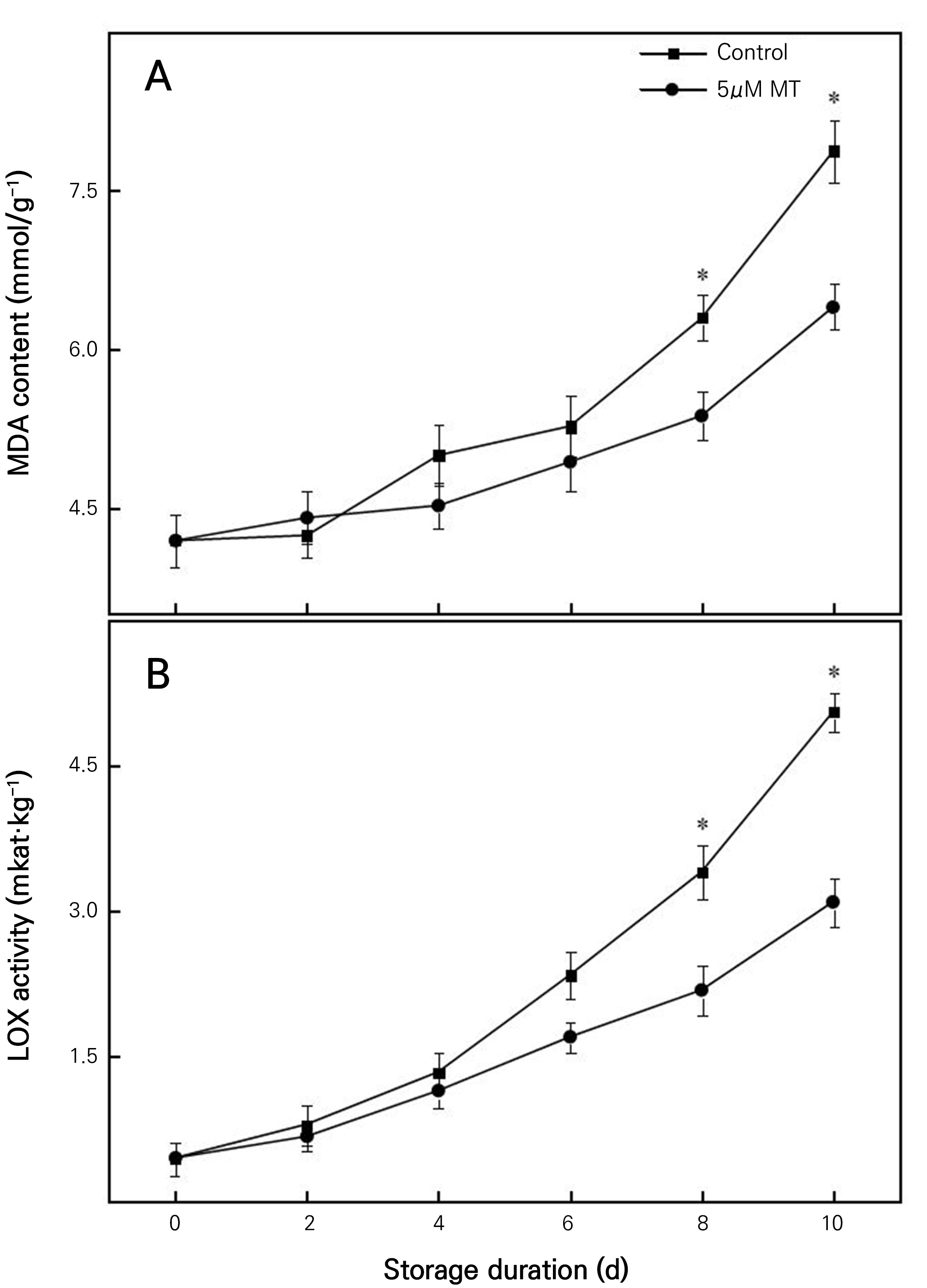
Fig. 4.
Effects of 5 µM MT on MDA content of cut chrysanthemum flowers. Values are presented as the arithmetic mean. The error bars represent the experimental standard errors of the means. Values with an asterisk (*) indicate MT-treated cut chrysanthemum flowers showing significant differences from the control at p < 0.05.
Effects of MT on leaf antioxidant enzyme activity in chrysanthemums
Increased activity of antioxidant enzymes in plants helps to slow down the aging process. In general, the leaf antioxidant enzyme activity tended to increase initially and decrease subsequently (Fig. 5). Between days 0 and 6, SOD activity did not significantly differ between the MT treatment groups and the control group (Fig. 5A). On days 8 and 10, SOD activity in the MT group was significantly higher than that in the control group (50.86% and 69.74% higher, respectively). POD activity was significantly elevated over control levels observed in the MT group (Fig. 5B). On days 8 and 10, POD activity in the MT group was significantly higher than that in the control group (34.81% and 39.71% higher, respectively). At the initial stage of storage, CAT activity in the MT group was not significantly different than that in the control group (Fig. 5C). Nevertheless, on days 8 and 10, CAT activity in the MT group was higher than that in the control group. These findings demonstrate that the 5 µM MT treatment significantly promoted the activity of antioxidant enzymes.
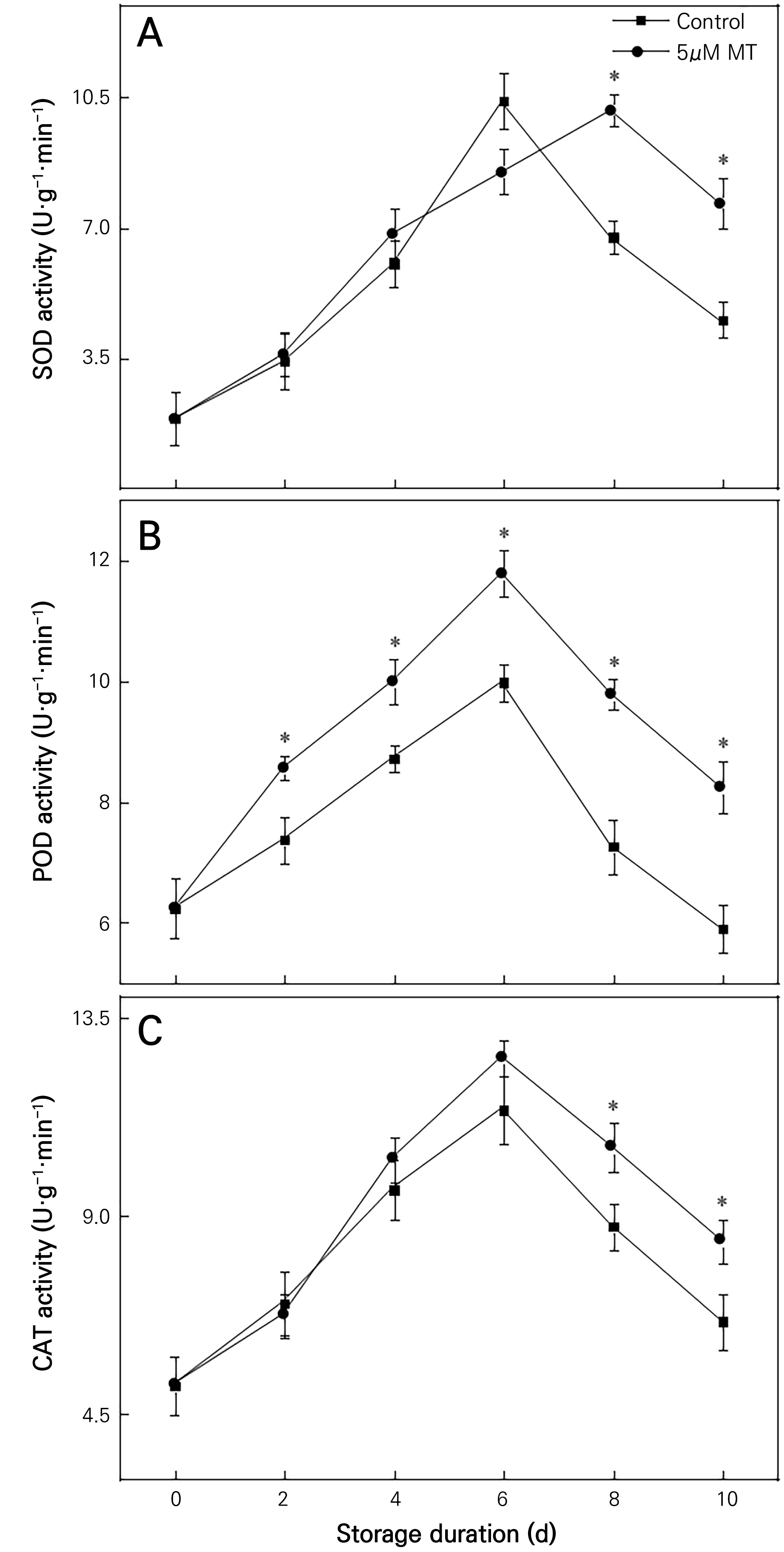
Fig. 5.
Effects of 5 µm mt on the antioxidant enzymes activities in the leaves of chrysanthemum flowers. values are presented as the arithmetic mean ± sd deviation of triplicate assays. the error bars represent the experimental standard errors of the means. values with an asterisk (*) indicate mt-treated cut chrysanthemum flowers showing significant differences from the control at p < 0.05.
Effects of MT on the Pro content in the leaves and petals of cut chrysanthemum flowers
Plant senescence accelerates internal proline accumulation. The Pro content of cut chrysanthemum flowers gradually increased with storage time, and MT-treated flowers showed lower Pro levels than control flowers (Fig. 6). It was also observed that the leaf Pro content of cut chrysanthemums treated with 5 µM MT was considerably lower than that in the control group on days 6, 8, and 10 (Fig. 6A). Similarly, petal Pro content in the 5 µM MT treatment groups was significantly lower than that in the control group (Fig. 6B). At the end of the storage period, the leaf and petal Pro content in the 5 µM MT treatment groups was 12.61% and 16.11% lower than that in the control group, respectively.
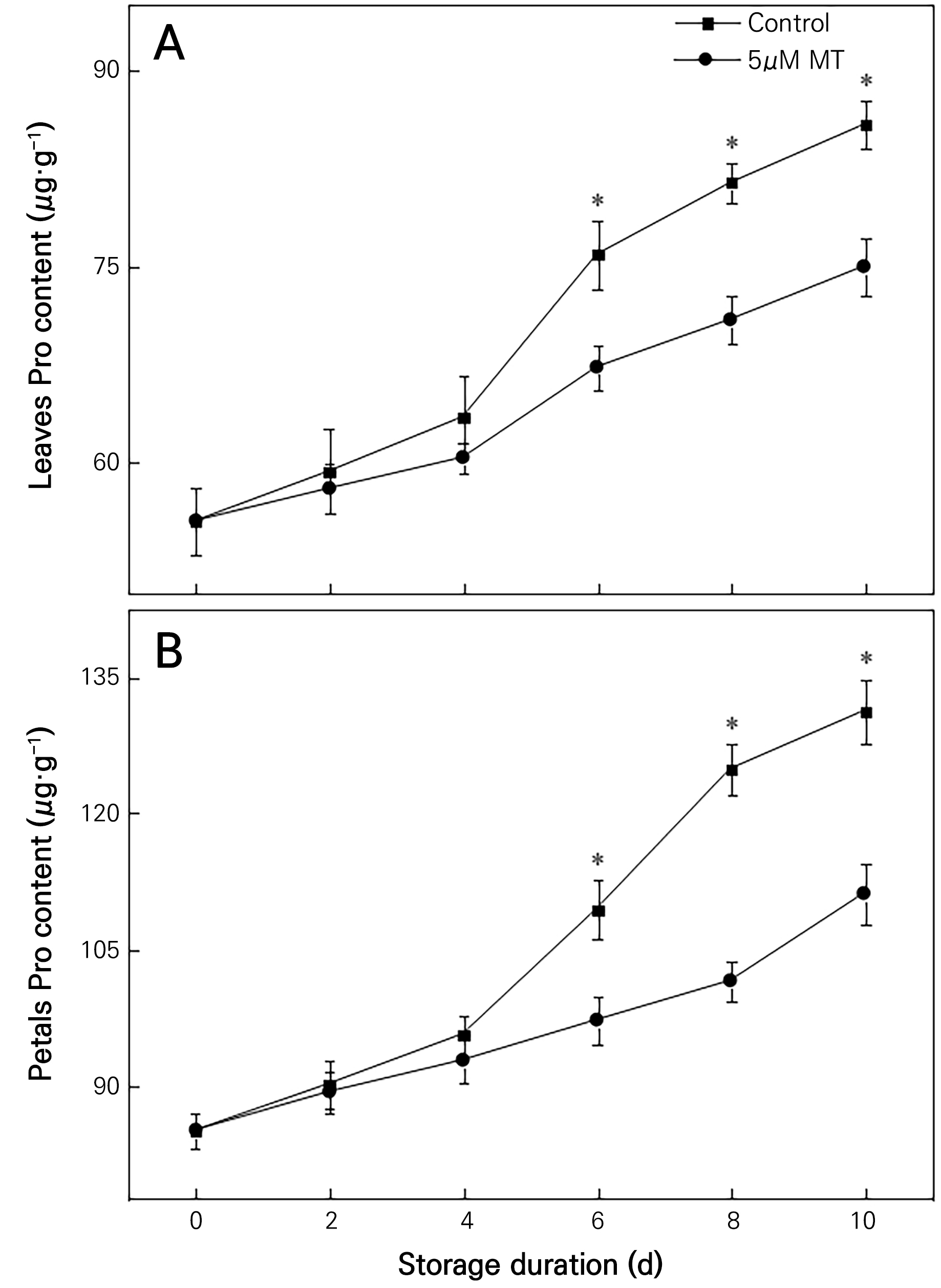
Fig. 6.
Effects of 5 µM MT on the Pro content in the leaves and petals of cut chrysanthemum flowers. Values are presented as the arithmetic mean. The error bars represent the experimental standard errors of the means. Values with an asterisk (*) indicate MT-treated cut chrysanthemum flowers showing significant differences from the control at p < 0.05.
Discussion
The onset of flower senescence is associated with a complex array of tightly regulated physiological and biochemical processes, encompassing protein degradation, membrane permeabilization, stem flexibility, floral abscission, changes in coloration, and leaf chlorosis (Reid and Jiang, 2012). These interconnected mechanisms collectively contribute to the deterioration of flower quality and longevity and help in understanding their intricate relationships, which is crucial for developing effective strategies to extend the postharvest life of flowers (Kaur and Jhanji, 2023). This study demonstrated that MT extends the life of cut chrysanthemum flowers by delaying the senescence of cut chrysanthemums. The beneficial effects of MT treatment on cut flowers were attributed to its ability to modulate water homeostasis, diminish lipid peroxidation, and boost antioxidant defenses, thereby mitigating the accumulation of oxidative damage and prolonging the storage duration of cut flowers.
Flower size is an important ornamental characteristic that increases the commercial value of ornamental plants (Noman et al., 2017). Xiang et al. (2020) showed that MT treatment increased the flower diameter of snapdragons. Liao et al. (2013) showed that treatment of cut roses with certain concentrations of exogenous NO increased the maximum flower diameter and prolonged the storage duration. Similar results were obtained in the present study. Decreased water absorption or increased water loss may be responsible for the changes in FW. The positive effect of MT on retaining FW is attributable to reduced transpiration, which prevents FW loss. It has been reported that the FW of cut flowers was positively affected by a S-carvone treatment, resulting in an increased storage duration (Damunupola et al., 2010). In the present study, the 5 µM MT treatment increased the RFW and RFD of cut chrysanthemum flowers, improving their quality and ornamental value.
Water balance is an important factor that affects the life of cut flowers (Woltering and Paillart, 2018). The heterogeneous distribution of water uptake within the xylem of the stem along with water loss through both stomatal and nonstomatal routes in leaves and other plant parts promote floral senescence (Roddy et al., 2016). Conversely, the maintenance of optimal water homeostasis within the plant delays the onset of senescence and extends the storage duration of cut flowers. By maintaining the water balance, the ornamental appeal and longevity of cut flowers can be significantly improved, thereby enhancing both their commercial value and consumer satisfaction (Li et al., 2021). Water loss reduction and water balance maintenance provide energy from carbohydrate metabolism to the petal cells (Gururani et al., 2023). It has been shown that a combined treatment with nano silver and aminoethoxyvinylglycine prevents water stress, which helps to maintain cell turgor and improve flower opening (Ha and In, 2023). Bacterial stem obstruction is another important factor that leads to insufficient water uptake, and thus premature wilting, of cut flowers and cut foliage (Lin et al., 2019). Ha et al. (2019) reported that a combined treatment with sodium hypochlorite, aminoisobutyric acid and 1-methylcyclopropene effectively inhibits bacterial growth in the vase, enhances solution uptake, and maintains the initial fresh weight and a positive water balance for longer periods of cut roses. In the present study, the water absorption of the 5 µM MT group was only significantly different at d10 compared to the control group. This may mean that MT has little effect on the bacteria in the solution. The extended storage duration of cut chrysanthemums realized by the MT treatment can be partly explained by its effects on the maintenance of appropriate water relationships, as observed in the 5 µM MT treatment group. In this study, the MT treatments delayed the water loss in cut chrysanthemums. Similarly, Lezoul et al. (2022) reported that an exogenous MT treatment resulted in an improved water balance of cut carnation flowers. Previously, it was reported that MT governed stomatal closure in the model plant Arabidopsis (Yang et al., 2021). In the present study, water absorption in the MT group showed little difference from that in the control; MT may have induced stomatal closure, leading to water balance maintenance.
The MDA content signifies the degree of lipid peroxidation, and an increased MDA content in cells indicates plant cell membrane damage (Mohammadi et al., 2023). As plants age, the MDA content of the leaves increases (Rogers, 1973). Increased lipid peroxidation increases phospholipid-degrading enzymes, such as phospholipase D and LOX, which leads to a loss of membrane integrity (Darvish et al., 2021). Under cellular stress, LOX enzymes are activated, and they facilitate the conversion of freely available unsaturated fatty acids into conjugated hydroperoxides through O2 oxidation; this reaction is believed to play a role in the regulation of various physiological processes (Singh et al., 2022). In the present study, the 5 µM MT group showed significantly lower leaf MDA contents and LOX activity levels. This finding is consistent with those in earlier works; Zeng et al. (2011) reported that a salicylic acid treatment inhibited MDA accumulation and LOX activity, and Zulfiqar et al. (2023) reported that a MT treatment reduced the MDA content of cut tuberoses. The longest storage duration was found in MT-treated leaves; this finding is attributable to the prolonged maintenance of the membrane integrity, indicating that exogenous MT effectively protected the integrity of the cell membrane. This is similar to the findings of Zhou et al. (2023).
ROS play a key role in plant cell damage and senescence by inducing DNA, protein, and lipid damage (Saeed et al., 2014). The homeostasis of ROS is dependent on the balance between ROS production and scavenging, which is usually tightly regulated in plants (Gechev et al., 2006). Antioxidant enzymes, including POD, SOD, and CAT, are crucial in maintaining redox homeostasis (Kumar et al., 2010). The activity of antioxidant enzymes signifies the ability to eliminate oxygen free radicals and ROS, which is inversely correlated with oxidative damage to the plant. Of the universal antioxidant systems in plants, SOD scavenges superoxide anion (O2−) radicals into H2O2, which is subsequently converted into water by CAT and APX (Apel and Hirt, 2004). Shaheen et al. (2015) reported that balanced zinc nutrition enhances the antioxidative activity in oriental lily cut flowers, leading to extended storage times. Mazrou et al. (2022) reported that a MT treatment significantly increases CAT and glutathione reductase activity in cut rose flowers. Similarly, Zhang et al. (2020) showed that an exogenous MT treatment promotes antioxidant gene expression, enhances the activity of antioxidant enzymes, and reduces the production of ROS in cucumber seedlings under salt stress. In the present study, a MT treatment significantly increased the activity of antioxidant enzymes (SOD, POD, and CAT), possibly reducing intracellular ROS levels and contributing to delayed cell senescence and death.
Proline, an amino acid highly associated with stress-related responses in plants, plays an important role in maintaining the redox balance and acts as a signaling molecule. It is well recognized for its role in plant stress responses (Lehmann et al., 2010). The increase in free Pro signifies the progression of senescence (Zhang and Becker, 2015). Pro maintains the osmotic pressure and reduces water loss in plant cells. Under abiotic stress, the Pro content increases owing to extensive protein degradation (Ghasemi et al., 2016). The Pro content in plants has been positively correlated with the degree of water deficit and cut flower senescence (Verbruggen and Hermans, 2008). In the present study, the 5 µM MT treatment significantly reduced the Pro content of leaves and petals. Thus, MT may maintain the water balance between leaves and petals by reducing water loss, which in turn alleviates water stress during senescence and reduces Pro production. Luo et al. (2023) showed that in drought-stressed chrysanthemum seedlings, exogenous melatonin increased the content of soluble proteins and soluble sugars while decreasing the content of proline; this is similar to the findings reported here. Similar findings were found in cut flowers; salicylic acid significantly suppressed Pro levels during storage, which in turn increased the storage duration of cut flowers (Bahrami et al., 2013).
Conclusion
The study demonstrated that MT acted as a positive regulator at certain concentrations and prolonged the storage duration of cut chrysanthemum flowers. An exogenous application of 5 µM MT enhanced the water balance and decreased the water loss in these flowers. Exogenous 5 µM MT significantly enhanced both the RFD diameter and RFW of cut chrysanthemum flowers. Consistent with this finding, 5 µM MT markedly decreased the MDA levels and LOX activity. In addition, the 5 µM MT application enhanced the activity of SOD, POD, and CAT in the leaves of cut chrysanthemum flowers. These findings suggest a key molecular mechanism for postharvest freshness preservation in chrysanthemums by MT. In summary, 5 µM MT effectively prolonged the storage duration of cut chrysanthemum flowers by promoting the enhancement of the antioxidant ability and reducing the water loss and MDA and Pro contents. Thus, to ensure a comprehensive understanding of the regulatory role of MT in delaying the senescence of cut flowers, additional research on the underlying molecular mechanisms is warranted. Specifically, future studies should focus on elucidating the expression patterns of relevant genes and their associated protein–protein interactions.