Introduction
Materials and Methods
Materials and experimental design
Chlorophyll fluorescence and chlorophyll content of leaves
Tomatoes growth characteristics and fruit cracking (%)
Quality analysis of the tomato fruits
Soil sampling and analyses
Statistical analysis
Results
Chlorophyll content and photosynthetic efficiency of leaves
Growth characteristics and fruit cracking (%)
Quality analysis of tomato fruits
Amino acid
Soil chemical and microbial properties
Discussion
Chlorophyll content and photosynthetic efficiency of leaves
Growth characteristics and fruit cracking (%)
Quality compositions of the tomato fruits
Amino acid
Soil chemical and microbial properties
Introduction
Tomato (Solanum lycopersicum L.), one of the most important vegetable crops, is cultivated extensively worldwide (Quinet et al., 2019) with an annual production of approximately 180 million tonnes (http://faostat.fao.org). Tomatoes offer a wealth of nutritional benefits, containing significant amounts of dietary fiber, vitamins, sugars, lipids, proteins, minerals, and various antioxidant molecules (George, 2004; de Alvarenga et al., 2017; Peixoto et al., 2017). Additionally, tomatoes are increasingly in demand due to their numerous health benefits, including their antioxidant, anti-inflammatory, antiallergenic, and anti-cancer properties (Raiola et al., 2014; Coyago-Cruz et al., 2017; Kaur et al., 2018; Ryu et al., 2019). The quality of tomato fruits is influenced by a range of factors, such as the variety, growth environment, and growth stage (Diouf et al., 2018). Consequently, agricultural systems have adopted various strategies to produce high-quality tomatoes, including the engineering of biofortified seeds or fruits that contain essential minerals for human consumption (Xu et al., 2022). These strategies aim not only to satisfy hunger and provide necessary nutrients to humans but also to prevent nutrition-related diseases while also enhancing the physical and mental well-being of consumers (Menrad, 2003). Quality factors, such as the size, firmness, color, taste, and nutritional content, are important criteria related to the successful marketing of tomato fruits (Helyes et al., 2006).
Fertilization impacts tomato yields and quality levels; traditionally, these crops are primarily cultivated by broadcasting inorganic fertilizers in several fertigation steps (Brunetti et al., 2019). Conventional farming systems rely heavily on inorganic fertilizers, which can compromise the environment and human health (Adhikari et al., 2018). The current global situation strongly emphasizes the need for eco-friendly agricultural practices to ensure sustainable food production (Bautista et al., 2020). Thus, there is an urgent need to develop environmentally friendly agricultural practices to address these challenges and ensure sustainable food production. This includes the implementation of live bioeffectors, abiotic bioeffectors and biofertilizers (Dudás et al., 2017). Silicon (Si) fertilizers, whether organic or inorganic, are recognized as high-quality and eco-friendly options that offer cost-effective benefits in agriculture owing to their non-corrosive and pollution-free traits (Tayade et al., 2022). Moreover, Si fertilizers play a beneficial role in regulating the soil pH and enhancing the uptake of both macro and micronutrients, especially when sourced from slag or Si-containing mineral ores (Liang et al., 2015).
Red clay covers 10% of the earth’s surface and is a soil type predominately found in the Republic of Korea (Jung et al., 2014). The main component of red clay is SiO2, and this soil type exhibits a multi-layered honeycomb structure with an extensive surface area (Hwang, 2000; Yang et al., 2020). The agricultural field has reported red clay availability (Kim et al., 2014); however, the prominent components that constitute red clay are insoluble and exhibit limited absorption in soil and plants, even when used as a powder (Seo et al., 2014a). Fortunately, processed red clay (PRC; Patent #0886082, Republic of Korea) is designed to remove impurities and enhance mineral bioavailability and to improve soil fertility and microbial activity (Jung et al., 2014; Yoon et al., 2016). Biofertilization utilizes plant and animal wastes to enrich soil with organic matter and nutrients, employing microorganisms to metabolize these by-products for enhanced absorption by plant roots (Montesdeoca-Flores et al., 2024). Certain types of lactic acid bacteria, such as Lactobacillus fermentum, are renowned biofertilizers for enriching agriculture as they can solubilize minerals such as silicates and phosphates (Jo et al., 2017; Kalayu, 2019; Bist et al., 2020). L. fermentum, a gram-positive bacterium of the Lactobacillus genus reportedly enhances and fortifies the immune response and prevents community-acquired gastrointestinal and upper respiratory infections (Naghmouchi et al., 2020). Research is ongoing to utilize probiotics in various ways beyond food fermentation, but their use in conjunction with crop cultivation remains very limited (Jo et al., 2017). Prior studies have explored the quality levels of radishes and cabbages cultivated with PRC (Seo et al., 2014b, 2015) and have reported the quality of eel (Anguilla japonica) after the application of PRC (Seo et al., 2014a). The effects of PRC on diesel bioremediation in soil bacterial communities and on the molecular mechanisms that enhance bacterial growth in hexadecane have also been studied (Jung et al., 2014, 2015). However, no previous studies have investigated applications of PRC in tomato cultivation or its potential effects on growth, fruit quality outcomes, and soil analysis findings.
This study aimed to explore the potential of sustainable tomato cultivation and production by minimizing the use of conventional chemical fertilizers and compost through the application of PRC, an eco-friendly material. The research analyzed the impact of PRC and microbial fertilizers on the growth, yield, quality of tomatoes, and soil properties. Another goal here is to investigate the synergistic effects between these factors, aiming to ascertain their potential as important aspects of eco-friendly agriculture.
Materials and Methods
Materials and experimental design
This study utilized a patented method (Patent# 0886082, Republic of Korea) to produce a material by blending red clay with 2M NaOH and heating it to 1200°C for 3 h (Ajeon Heating Industrial Co., Korea). This process improves the solubility of SiO2 in a colloidal form, as confirmed by an analysis using an inductively coupled plasma-optical emission spectrometer (DV3300, Perkin Elmer, USA). The resulting material was ground to 20–50 µm according to Jung et al. (2014) and Yoon et al. (2016). The chemical composition of PRC consisted of SiO2 (31.1%), Na2O (25.6%), Al2O3 (19.0%), Fe2O3 (7.47%), K2O (1.30%), CaO (0.95%), TiO2 (0.94%), MgO (0.73%), and other materials (13.91%). The study also utilized a brown liquid formulation known as microbial fertilizer, which contains Lactobacillus fermentum (MFcL). This organic agricultural material is patented under patent #10-2000-0023186 in the Republic of Korea.
Seeds of the ‘Sinheuksu’ tomato (Solanum lycopersicum L.), purchased from Asiaseed Co. Ltd. (Seoul, Korea), were planted individually in seeding trays in a greenhouse. Four-week-old seedlings were transplanted to the field on May 18, 2015. The experiment lasted 58 days and was conducted at an agricultural research farm of Dongguk University (Siksa-dong, Ilsandong-gu, Goyang-si, Gyeonggi-do, Korea; Location: 37° 68' 01.31” N latitude 126°, 80' 35.48” E longitude). The average minimum temperature recorded during the experiment was 13.0°C, while the average maximum temperature was 27.9°C (Fig. 1A). The total precipitation recorded was 37.3 mm, and the average humidity was 61% (www.kma.go.kr). The soil chemistry of the experimental site prior to planting was as follows: pH, 7.0; electrical conductivity (EC), 0.34 dS·m-1; organic matter (OM), 6 g·kg-1; available phosphorus (Av. P2O5), 47 mg·kg-1; available silicon dioxide (Av. SiO2), 187 mg·kg-1; calcium (Ca2+) 5.8 cmol+·kg-1, magnesium (Mg2+), 2.9 cmol+·kg-1; potassium (K+), 0.27 cmol+kg-1; and total nitrogen (TN) 0.04%.
The experimental design was a completely randomized design (CRD) with three replications (Fig. 1B). Tomato seedlings were planted in plots (1.8 m × 2.4 m), and each plot contained six plants, with two of them serving as buffer zones to prevent contamination. The basal fertilizer used was compost (1,000 kg·10a-1) applied before plowing, and nitrogen fertilizer (Urea 8.8N–0P–0K) was top-dressed (NIAST, 2010b). The treatments were applied four times at seven-day intervals using three different solutions: non-fertilizer (NF, only water), PRC (diluted 200-fold), and MFcL (diluted 250-fold).
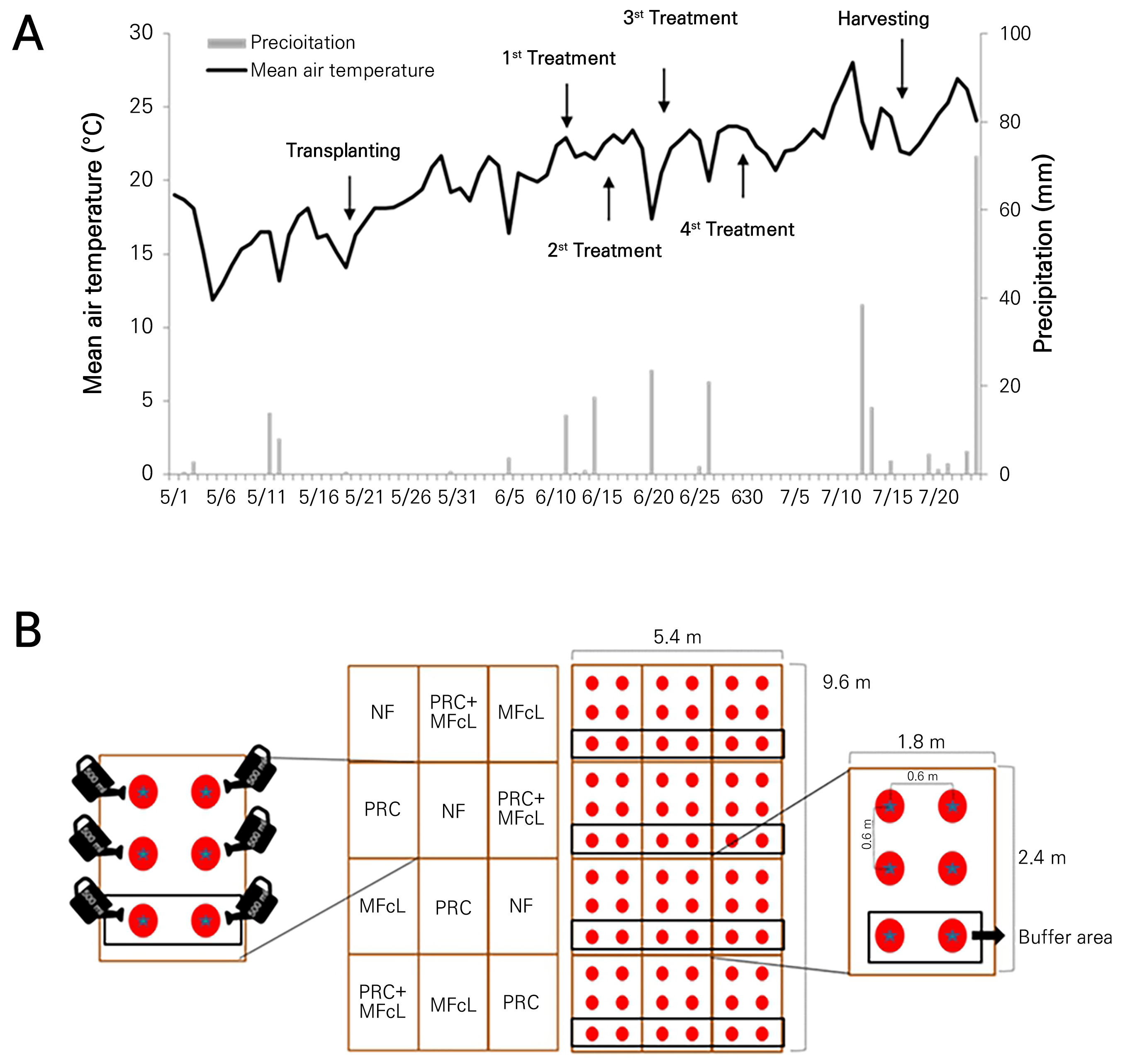
Fig. 1.
Mean air temperature and precipitation during the experimental period of May 1 to July 24 (2015) at an agriculture research center at Dongguk University (A), and experimental field design of each plot size of 1.8 m × 2.4 m (B), Black square: buffer area, NF: non-fertilizer (only water: 500 mL); PRC: processed red-clay (200-fold dilution: 500 mL); MFcL: microbial fertilizer containing L. fermentum (250-fold dilution: 500 mL), PRC+MFcL: PRC (200-fold dilution: 250 mL) + MFcL (250-fold dilution: 250 mL).
Chlorophyll fluorescence and chlorophyll content of leaves
Chlorophyll fluorescence was measured four times at seven-day intervals between 10 am and 12 pm, 14 days after the treatment (DAT). The measurements were taken on the third youngest attached leaf and were done on three plants at each treatment. A portable fluorometer (Opti-sciences OSI 30p, UK) was used to measure chlorophyll fluorescence following a 20-min period of dark adaptation. The minimal fluorescence (Fo), maximum fluorescence (Fm), and variable fluorescence (Fv/Fm) values were measured according to a method described by Baker (2008).
Tomato leaves were collected from each treatment between the third and fourth divisions and were cut into 1 mm pieces. Briefly, an amount of 0.1 g of fresh leaves was incubated in 80 mL of 80% (v/v) acetone in the dark at 4°C for seven days. The absorbance was measured using a UV/VIS spectrometer (UV-2100, Shimadzu, Japan) at 663 nm, 645 nm, and 470 nm. The chlorophyll and carotenoid contents were calculated using the equations provided by Arnon (1949) and Lichtenthaler (1987). The chlorophyll and carotenoid contents were calculated using the following equations:
Tomatoes growth characteristics and fruit cracking (%)
After 58 days transplanting, we harvested the fruits when they reached the red stage (when 90% of the fruit surface exhibited red coloration, following the ripening criteria for tomatoes) as described by Cantwell (2000). Data were taken from four plants for every three replications in accordance with the Agricultural Science and Technology Analysis Standard (RDA, 2012). All harvested fruits were analyzed for growth characteristics, specifically for the fruit length, width, weight, fruit shape index (FSI), yield, and cracking percentage. Fruit length (mm) and width (mm) were measured using Vernier calipers (CD-15CP, Mitutoyo Corp., Japan), while FSI was determined as the ratio of the fruit length to the fruit width. To investigate the incidence of fruit cracking, the harvested fruits were visually inspected and sorted into two categories: normal and cracked. The cracked fruits were further categorized based on their appearance, which included radial, concentric, irregular, and a category termed multiple type. The rate of fruit cracking (%) was determined using the following formula:
Quality analysis of the tomato fruits
The moisture content and total soluble solids (TSS) were measured in ten fruits for each treatment. Moisture content was determined by weighing the fruit before and after drying each one in an oven at 105°C for 5 h (AOAC, 1990). TSS was measured using a digital handheld refractometer (PAL-3, Atago, Japan).
The total glucose and fructose contents were analyzed by means of an HPLC device (Shiseido Nanospace SI-2, Tokyo, Japan) equipped with a Unison UK-Amino column (250 mm × 3.0 mm, Imtakt Co. USA). The HPLC instrument was equipped with a RI detector (Shodex, Japan). The sample (0.1 g) in each case was dissolved in a solution containing H2O: ethanol (EtOH) (1:1) at a volume of 20 mL, followed by an ultrasonic treatment at 80°C for 30 min. The resulting mixture was centrifuged at 3000 rpm and 25°C and then filtered through a 0.45 μm syringe filter. The mobile phase consisted of 90% acetonitrile, and the column was maintained at a temperature of 55°C. The flow rate was set to 400 µL·min-1.
The crude fat and protein contents were analyzed using the AOAC method (2005). Crude fat was extracted and quantified with dimethyl ether using an analyzer (Soxtec 2050 Analyzer Unit, FOSS Tecator, USA), while protein was analyzed using a protein extractor (2300 Kjeltec Analyzer Unit, FOSS Tecator, USA) through the micro-Kjeldahl method.
The amino acids were extracted from the proteins using the ninhydrin post-column reaction method in conjunction with ion exchange chromatography. This method was modified from the AOAC method (2005). The amino acid was obtained using an analyzer (L-8900, Hitachi High Technologies Corporation, Japan). The sample (0.2 g) was mixed with 20 mL of 6 N HCl in digestion tubes, vortexed for 1 min, and then hydrolyzed for 22 h at 110°C for further analysis. The extract was concentrated using a vacuum concentrator (CCA-1111-CE, EYELA, Unit C Bohemia, USA) and then diluted to a final volume of 25 mL with a 0.2 N sodium citrate buffer (pH 4.2). The solution was filtered through a 0.45 µm nylon syringe filter (Whatman Inc., USA) and subsequently analyzed using a cation exchange column (60 mm × 4.6 id mm). The column temperature was set to 57°C, and the reaction temperature was set to 135°C. The flow rate was 0.4 mL·min-1 and the injection volume was 20 μL. The analysis was conducted using a UV/Vis detector, measuring 440 nm and 570 nm. Amino acids were eluted using ninhydrin, ninhydrin buffer solution, and mobile phase solution (PH-1, PH-2, PH-3, PH-4 and PH-RG).
Soil sampling and analyses
The soil samples were collected and analyzed following the standards outlined by NIAST (2010a). The soil samples were collected in triplicate before transplanting and after harvesting. Each soil sample was taken from a depth of 20 cm using an auger and represented a composite of ten cores collected from each treatment. The soil analyses were conducted on air-dried samples that were sieved through a 2-mm mesh. Soil pH was measured by a pH meter based on a soil/distilled water (w/v) mixture at a ratio of 1:5 and EC was measured in a soil/water mixture (1:5) using an electromagnetic device. OM was analyzed using the Tyurin method (Tyurin, 1931). TN was measured using the Kjeldahl method (Kirk, 1950). Av. P2O5 was measured using the Lancaster method (Alban et al., 1964). The concentration of Av. SiO2 was determined by measuring the absorbance at a wavelength of 700 nm using a 1 N NaOAc (pH 4.0) buffer. The exchangeable cations in the filtrate were extracted using 1N NH4OAc and then analyzed with an ICP-OES spectrometer (Optima 8300, Perkin Elmer, USA).
The soil microbe density was analyzed using the soil dilution plate method (Clark, 1965) to determine the population of bacteria and the actinomycetes in the soil. The soil samples were collected and stored in a cooler with ice. The samples were diluted with distilled water and shaken on a shaker for 10 min. Yeast glucose agar was used as a general medium for bacterial growth, while starch casein agar supplemented with 30 mL of streptomycin was employed to cultivate the actinomycetes. The plates were incubated for three to five days at 28±2°C for the bacteria and for seven days at the same temperature for the actinomycetes. The observer counted the number of colonies on each plate manually using a colony counter. The values for bacteria and actinomycetes were expressed as colony-forming units per gram of dry soil (CFU·g-1).
Statistical analysis
A one-way analysis of variance (ANOVA) was used to analyze the data, and Duncan’s multiple range test (DMRT) comparisons (p < 0.05) were conducted to assess significant means using SPSS 28.0 (IBM Corp., Armonk, USA).
Results
Chlorophyll content and photosynthetic efficiency of leaves
The levels of chlorophyll and carotenoid content, measured at seven-day intervals starting from 14 DAT, showed a gradual decrease over time (Fig. 2A-2C). Notably, at 35 DAT, just before harvesting the tomatoes, the MFcL group demonstrated significantly higher values compared to all other experimental groups: 6.81 mg·g-1FW for chlorophyll a (Fig. 2A), 3.46 mg·g-1FW for chlorophyll b (Fig. 2B), and 1.07 mg·g-1FW for carotenoids (Fig. 2C). In particular, the carotenoid content at 35 DAT exhibited values exceeding 1.0 in MFcL and PRC+MFcL, with 31.65% and 19.52% higher values than NF, respectively. The range of observed leaf fluorescence efficiency values was 0.76–0.81. Although there were no differences in fluorescence efficiency values among the treatments at 14 DAT, statistical differences were observed 21 DAT days. MFcL maintained a value of approximately 0.81 (Fig. 2D).
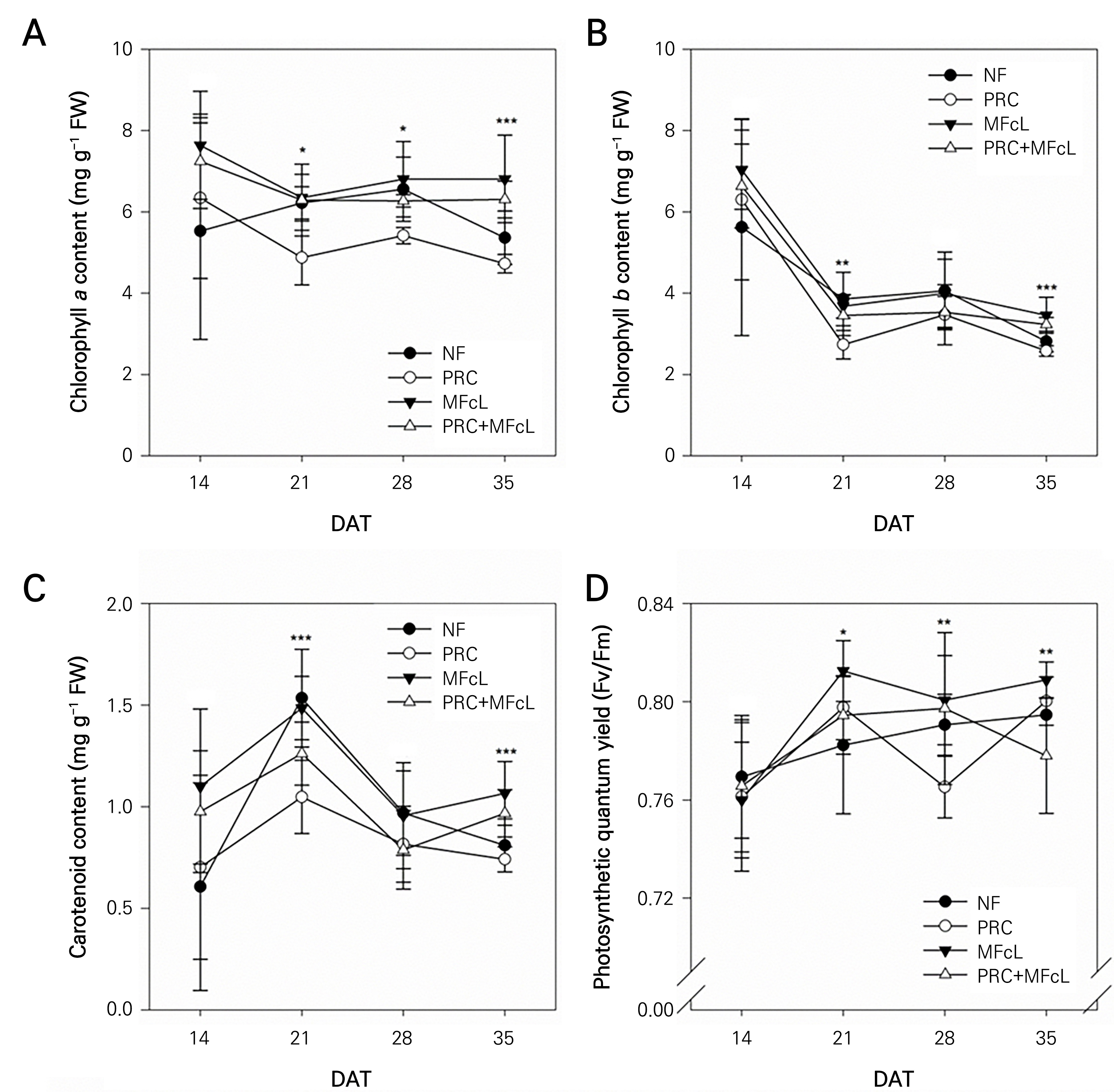
Fig. 2.
Effects of different treatments on the chlorophyll a (A), chlorophyll b (B), and carotenoid (C) contents and changes in the maximum quantum yield (Fv/Fm) (D) for tomatoes cultivated in an open field for 35 days after each of the different treatments (DAT). Data represent the mean ± SD. Asterisks on the bar indicate significant difference according to Duncan’s multiple range test (DMRT) (*p < 0.05, **p < 0.01 and ***p < 0.001, n = 6). NF: non-fertilizer (only water: 500 mL); PRC: processed red-clay (200-fold dilution: 500 mL); MFcL: microbial fertilizer containing L. fermentum (250-fold dilution: 500 mL), PRC+MFcL: PRC (200-fold dilution: 250 mL) + MFcL (250-fold dilution: 250 mL).
Growth characteristics and fruit cracking (%)
The results of the fruit growth traits of the fruit length, width, weight, and FSI of the tomatoes harvested after treatment with PRC and MFcL are presented in Table 1. Although the tomato length exhibited no significant differences among the treatments, there was a notable decrease of approximately 4.6% in the fruit width within the MFcL compared to the NF. Similarly, the fruit weight demonstrated a tendency to decrease in MFcL, being approximately 10.6% lower than NF. Significant (p < 0.05) differences were observed in FSI among the treatments, with this value being lowest in NF (0.89). In the PRC and PRC+MFcL cases, it increased by 4.3% and 3.2%, respectively, compared to NF. However, MFcL did not show a significant difference compared to NF.
Table 1.
Comparative analysis of tomato fruit characteristics as affected by the different treatments
Treatmentsz | Length (mm) | Width (mm) | Fresh weight (g) | Dry weight (g) | FSI |
NF | 46.10 ay | 52.09 a | 76.88 a | 1.01 a | 0.89 c |
PRC | 46.34 a | 50.28 ab | 71.89 ab | 0.92 a | 0.92 a |
MFcL | 45.49 a | 50.63 ab | 71.17 ab | 1.12 a | 0.90 bc |
PRC+MFcL | 45.40 a | 49.69 b | 68.70 b | 0.91 a | 0.91 ab |
Concerning the number of fruits, NF resulted in approximately four more fruits compared to using PRC and MFcL alone (Fig. 3). However, despite the increased numbers of fruit, the percentage of cracking in NF was approximately eight times higher than that of PRC.
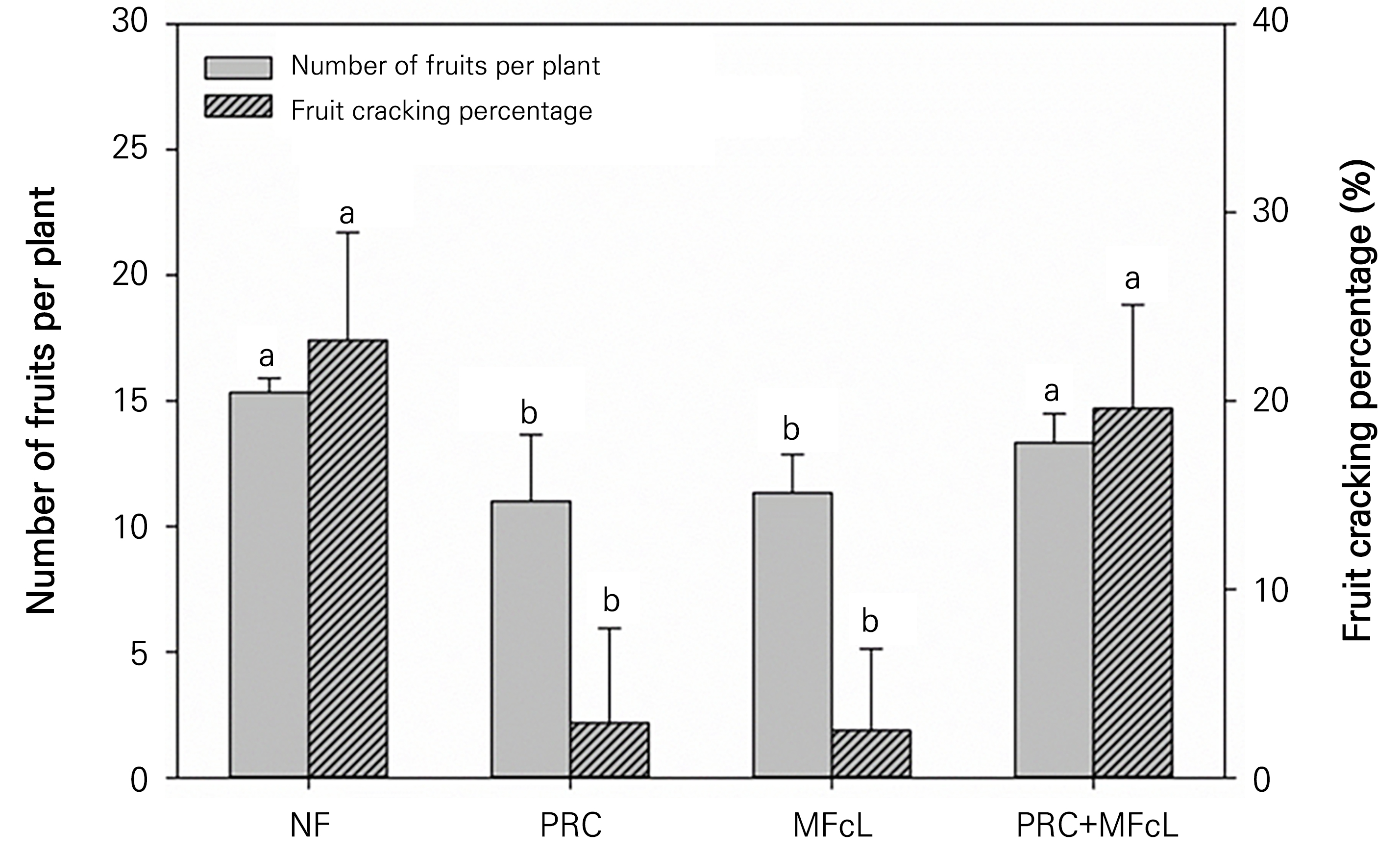
Fig. 3.
Number of fruits per plant and fruit cracking percentage (%) according to the different treatments. Data represent the mean ± SD. Different letters on a bar indicate significant differences according to Duncan’s multiple range test (DMRT) (p < 0.05, n = 3) NF: non-fertilizer (only water: 500 mL); PRC: processed red-clay (200-fold dilution: 500 mL); MFcL: microbial fertilizer containing L. fermentum (250-fold dilution: 500 mL), PRC+MFcL: PRC (200-fold dilution: 250 mL) + MFcL (250-fold dilution: 250 mL).
Quality analysis of tomato fruits
No significant differences were observed in the moisture content and TSS of the fruits among the treatments. However, the moisture content of the tomatoes was approximately between 93 and 94% (Fig. 4A), while the TSS was in the range of 5.1–5.7 °Brix (Fig. 4B). A glucose content analysis showed no significant differences among the treatments (Fig. 4C), whereas the fructose content exhibited an increase in the MFcL case (Fig. 4D). PRC demonstrated a tendency to decrease by 0.93 mg·g-1 compared to NF. Furthermore, when MFcL was combined with PRC, the fructose content increased by approximately 1.2 mg·g-1 compared to when PRC was used alone. The crude fat content in PRC increased significantly to 3.2 mg·g-1, a value approximately three times higher than NF (Fig. 4E). The total protein content of the tomatoes in the PRC treatment increased to 10.03 mg·g-1, higher than for NF and MFcL alone. The PRC+MFcL treatment also showed an increase in the protein content similar to that of the PRC treatment (Fig. 4F).
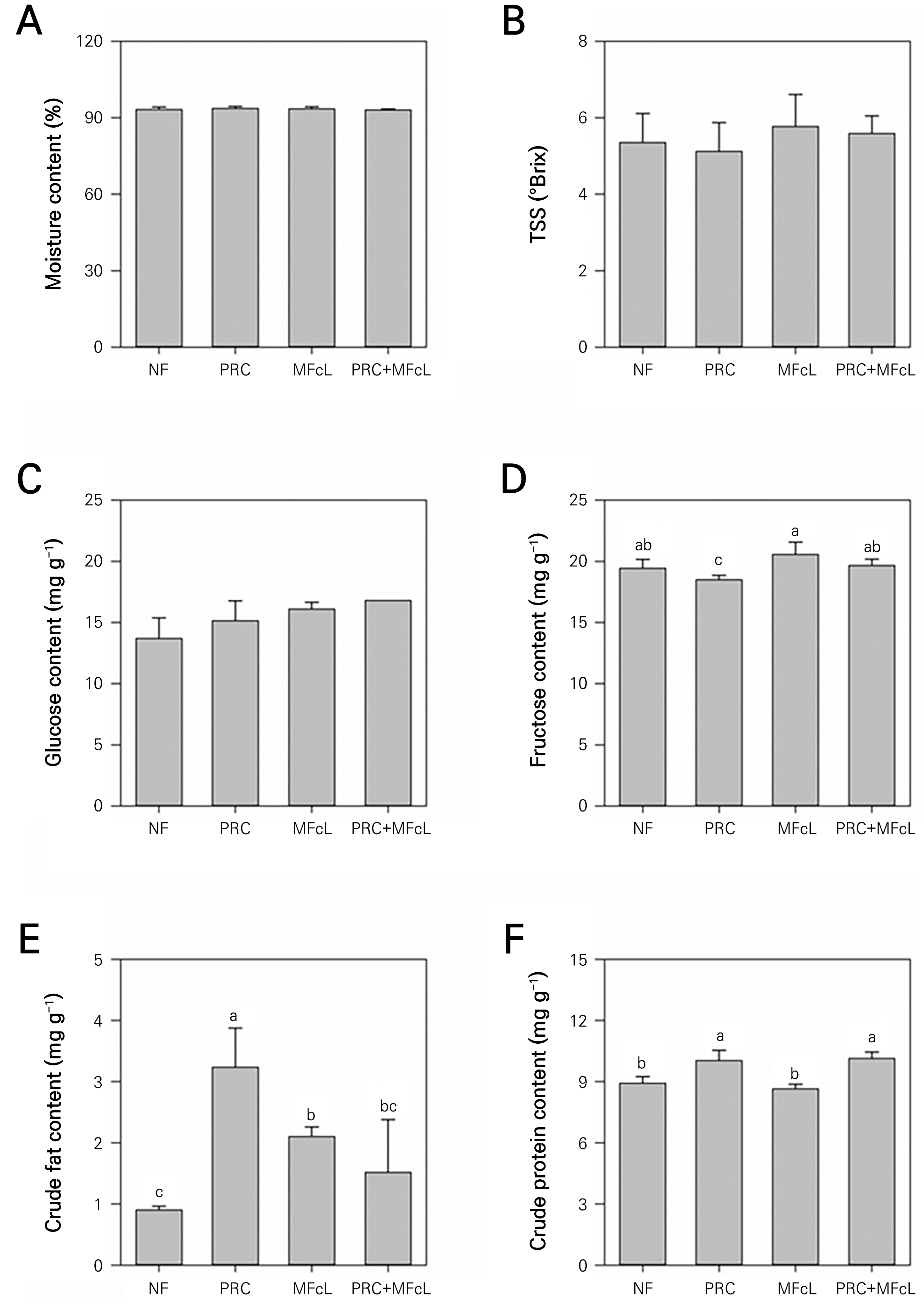
Fig. 4.
Effects of different treatments on the moisture content (A), TSS (B) and glucose (C), fructose (p < 0.05) (D), crude fat (p < 0.001) (E), and crude protein (p < 0.001) (F) contents of tomatoes cultivated in an open field for 35 days after different treatments. Data represent the mean ± SD. Different letters (s) on a bar indicate significant differences according to DMRT (p < 0.05, A-B: n = 10; C-F: n = 3) NF: non-fertilizer (only water: 500 mL); PRC: processed red-clay (200-fold dilution: 500 mL); MFcL: microbial fertilizer containing L. fermentum (250-fold dilution: 500 mL), PRC+MFcL: PRC (200-fold dilution: 250 mL) + MFcL (250-fold dilution: 250 mL).
Amino acid
The total amino acid content in PRC was increased significantly by approximately 6% compared to NF and 9% compared to MFcL alone, with a total value of 7.93 mg·g-1 (Table 2). An analysis of 15 amino acids revealed that in the PRC case, the contents of threonine, leucine, aspartic acid, and glutamic acid showed significant increases compared to those in the NF case. Additionally, the PRC+MFcL treatment showed the highest proline content.
Table 2.
Free amino acid contents of tomatoes according to the different treatment unit: (mg·g-1)
Treatmentsz | NF | PRC | MFcL | PRC+MFcL |
Threonine | 0.26 aby | 0.27 a | 0.25 b | 0.27 a |
Valine | 0.15 a | 0.23 a | 0.22 a | 0.23 a |
Isoleucine | 0.21 a | 0.22 a | 0.21 a | 0.21 a |
Leucine | 0.33 b | 0.35 a | 0.34 b | 0.35 a |
Phenylalanine | 0.29 a | 0.31 a | 0.31 a | 0.31 a |
Lysine | 0.27 a | 0.29 a | 0.27 a | 0.24 a |
Histidine | 0.11 b | 0.11 a | 0.11 b | 0.11 a |
Arginine | 0.16 a | 0.17 a | 0.16 a | 0.17 a |
Aspartic acid | 0.96 b | 1.00 a | 0.91 c | 1.02 a |
Serine | 0.36 a | 0.38 a | 0.36 a | 0.38 a |
Glutamic acid | 3.48 b | 3.69 a | 3.30 c | 3.65 a |
Glycine | 0.17 a | 0.21 a | 0.20 a | 0.21 a |
Alanine | 0.23 ab | 0.24 ab | 0.22 b | 0.25 a |
Tyrosine | 0.37 a | 0.40 a | 0.39 a | 0.39 a |
Proline | 0.05 b | 0.05 b | 0.05 b | 0.07 a |
Total | 7.51 b | 7.93 a | 7.30 b | 7.85 a |
Soil chemical and microbial properties
In the comparison of NF, the treated soil samples exhibited higher levels of OM, Av. P2O5, and K+ and lower levels of EC, Av. SiO2, TN, Ca2+, and Mg2+ (Table 3). NF had a higher pH compared to MFcL. However, PRC+MFcL showed no significant difference in the pH compared to NF. The pH increased with the combined application of MFcL and PRC as opposed to that with MFcL alone. Notably, the changes in Av. P2O5 and Av. SiO2 content between NF and the treatments were pronounced in this study. In NF, Av. P2O5 and Av. SiO2 contents were 94.00 mg·kg-1 and 289.67 mg·kg-1, respectively. Additionally, the Av. P2O5 content in MFcL increased by 49.7% compared to the NF case, reaching 140 mg·kg-1, whereas the Av. SiO2 content decreased by 26.8%. The exchangeable cation outcomes also exhibited differences between PRC and MFcL. Both showed a tendency to decrease in the Ca2+ and Mg2+ contents compared to NF, while K+ content in MFcL showed a slight increase of approximately 0.04 compared to that of NF. The application of MFcL alone or in combination with PRC resulted in bacteria populations 1.6 times higher than those in NF. In contrast, the quantities of actinomycetes were highest in NF at 32.00 CFU·g-1, while MFcL decreased them by 1.3 times and PRC by 1.8 times compared to NF. The combined application of MFcL and PRC resulted in a synergistic increase in the quantities of both bacteria and actinomycetes.
Table 3.
Soil chemical properties and effects on the soil microbial quantity after different treatments in open field tomatoes
Treatmentsz | pH | EC | OM |
Avail. P2O5 |
Avail. SiO2 | TN |
Exchangeable cations (cmolc·kg-1) |
Bacteria (×106) |
Actinomycetes (×105) | ||||||
(1:5) | (dS·m-1) | (g·kg-1) | (mg·kg-1) | (%) | Ca | Mg | K | (CFU·g-1) | |||||||
NF | 6.60 ay | 0.86 a | 6.67 c | 94.00 c | 289.67 a | 0.08 a | 6.29 a | 2.80 a | 0.32 c | 16.33 b | 32.00 a | ||||
PRC | 6.43 b | 0.68 b | 8.00 b | 95.67 b | 254.33 b | 0.07 c | 5.56 b | 2.60 b | 0.30 d | 21.33 ab | 17.33 c | ||||
MFcL | 6.00 c | 0.69 b | 8.00 b | 140.67 a | 212.00 d | 0.06 c | 4.53 d | 1.90 d | 0.36 a | 26.33 a | 24.33 b | ||||
PRC+MFcL | 6.57 a | 0.62 c | 9.00 a | 141.33 a | 243.67 c | 0.07 b | 5.23 c | 2.07 c | 0.35 b | 25.33 a | 28.67 ab |
Discussion
Chlorophyll content and photosynthetic efficiency of leaves
In this study, MFcL increased the chlorophyll content, which indicates a microbial influence. Notably, the inclusion of PRC was responsible for the lower chlorophyll content. This study found that MFcL increased the levels of both chlorophyll and carotenoids compared to NF. However, PRC had a limited influence on the chlorophyll content. Conversely, in this study, the combination of PRC and MFcL increased both the chlorophyll and carotenoid contents. According to Zhang et al. (2018), under non-stress conditions, a treatment with K2SiO3 showed no significant difference compared to a control group of tomatoes. However, a K2SiO3 treatment at a high concentration actually led to a decrease in the chlorophyll content (Cao et al., 2013). Vu et al. (2017) also reported that a silicate fertilizer treatment increased the chlorophyll content up to a certain concentration, noting also a decrease at high concentrations. Additionally, Cumplido-Nájera et al. (2019) observed a decrease in the chlorophyll content at high concentrations of K2SiO3. Such decreases in the chlorophyll content and increases in the carotenoid content are associated with plant tolerance and the physiological status of the plant under stress conditions (Abdel Latef and Chaoxing, 2011), with such phenomena similar to low-temperature stress and salinity conditions (Vasil’eva et al., 2003; Abdel Latef and Chaoxing, 2011; Gharbi et al., 2018). The developmental stage of leaves and the environmental conditions influence carotenoid metabolism, and substances related to carotenoids support photosynthesis, photoprotection, and stress adaptation in leaves. Additionally, when Piriformospora indica is inoculated in tomatoes, there is an increase in the photosynthetic pigment content (Ghorbani et al., 2018). Similarly, an increase in the photosynthetic pigment content has been reported in mung beans when treated with a Si solution and rhizobacteria inoculation (Mahmood et al., 2016). Beneficial bacteria that become established in a plant’s rhizosphere interact with the plant, aiding in the maintenance of chlorophyll and carotenoid pigments in crops during stressful conditions. These bacteria also regulate the levels of osmolytes, such as proline, soluble sugars, proteins, and amino acids, which improve the stability of the cell membranes, meaning that the bacteria assist plants in adapting to stressful environments (Qu et al., 2016; El-Esawi et al., 2018; Zhang et al., 2020).
Fluorescence efficiency (Fv/Fm) is an indicator of various environmental forms of stress that can affect the physiological aspects of photosynthesis (Hong and Xu, 1999). Generally, a range of 0.78–0.84 indicates the healthiest state of plant growth (Kitajima and Butler, 1975), and this study found that the fluorescence efficiency ranged from 0.76 to 0.81. It was also found here that treating the rhizosphere with MFcL enhanced plant pigments compared to other treatments, stemming from the chlorophyll content and fluorescence efficiency. At 35 DAT, the Fv/Fm value in the PRC+MFcL case was significantly lower (0.78) compared to the other treatments, which can be assumed to act as a stress factor related to the increased carotenoid content. Based on the research findings of Strzałka et al. (2003), MFcL can cause increases in photosynthetic pigments, and the addition of MFcL in combination with PRC shows a more significant effect compared to that by PRC alone. However, this response was not observed in the single applications of PRC and MFcL, indicating that further research is needed to understand the synergistic effect of PRC and MFcL in tomato plants.
Growth characteristics and fruit cracking (%)
The analysis of the tomato fruit growth parameters revealed that the individual treatments with PRC and MFcL had no significant effects. However, in the combined treatment of PRC+MFcL, a decreasing trend in growth traits was found compared to NF. Additionally, the quantity and cracking percentage decreased in the PRC and MFcL treatments compared to the NF case, but the PRC+MFcL application increased the number of fruits per plant and the fruit cracking percentage. This result is similar to the findings of Kaloterakis et al. (2021), who reported that the application of silicon and Bacillus spp. or their combination during the cultivation of Phaseolus vulgaris did not have a significant effect on plants under non-stress conditions. These results were also in line with the findings of Mayak et al. (2004), who observed that inoculation with A. piechaudii ARV8 did not have a substantial effect on tomato plant growth under non-stress conditions. Kumar et al. (2020) found that under non-stress conditions, the application of plant-growth-promoting bacteria (PGPR) and silicon fertilization did not have significant effects. However, optimizing the interaction between these factors at specific concentrations may enhance plant growth. Weerahewa and David (2015) suggested that varying silicon fertilizer concentrations during different growth stages could impact tomato traits. Additionally, the growth conditions of the plants could affect the quality and metabolism of tomato fruit (Diouf et al., 2018). A FSI score close to 1.0 indicates a round shape, and PRC exhibited round-shaped fruits. Besides the genetic traits, the shape of a tomato is also influenced by its cultivation environment (Lohar and Peat, 1998; Moon et al., 2015; Park et al., 2017). In particular, variations in the fruit quantity and biomass can affect the fruit shape in open-field cultivation (Le et al., 2018). Clay minerals are recognized for their ability to improve the physical and chemical properties of soils. Silicon contributes to the mechanical strengthening of plants by forming silicified elongated and short epidermal cells, a thick layer of silica beneath the cuticle, and complexes with organic compounds on the epidermal cell walls (Wang et al., 2017). The lowest number of cracked fruits in the PRC stems from the high absorption of minerals such as SiO2, NaO, and CaO by the plants. Various factors, such as the genetic makeup, cultivation method used, and climatic conditions influence the occurrence of cracked tomatoes, leading to a decline in quality (Peet, 1992; Khadivi-Khub, 2015; Yang et al., 2016; Zhang et al., 2020). Pinedo-Guerrero et al. (2020) reported that a co-treatment with silicon nanoparticles (SiO2 NPs) and NaCl increases fruit firmness, thereby preventing fruit cracking. Additionally, the provision of Ca2+ can decrease the incidence of cracked fruits and other aspects of quality deterioration (Savvas et al., 2017; Bae et al., 2023). Mineral nutrition and cracking are closely related, especially with regard to the Ca2+ and B levels, as they are associated with pectin, a substance that contributes to the firmness of the tomato surface (Yang et al., 2016).
In this study, we observed a decrease in the fruit size but an overall increase in the fruit yield in the PRC+MFcL treatment, suggesting its potential use in relation to these interactions in tomato plants. Thus, it is necessary to determine the optimal concentration levels for these types of interactions during PRC and MFcL applications. The results here suggest the need to conduct molecular research on the combined application of PRC and MFcL in tomato plants.
Quality compositions of the tomato fruits
In this study, no significant differences were observed in the moisture contents of the tomato fruits. However, MFcL resulted in increased TSS, glucose, and fructose contents of the fruits compared to the NF and PRC treatments, while PRC+MFcL showed a tendency toward higher sugar content compared to PRC alone. The enhanced efficiency of potassium and phosphorus utilization in the roots resulted in phosphorus accumulation in the fruits, which likely contributed to the improvement of the fruits’ sugar levels (Go et al., 2023). Bona et al. (2018) observed that the glucose and fructose contents of tomato fruits increased when a mixture of mycorrhiza inoculum and bacterial suspension was used. Glucose and fructose contents significantly increase during the early stages of fruit development (Quinet et al., 2019) and occur in equal quantities during fruit ripening (Oms-Oliu et al., 2011). The primary sugars that influence the sweetness of tomatoes are fructose, glucose, and sucrose, along with a small amount of starch. Kurina et al. (2021) noted that changes in the sugar content in cultivated tomatoes can vary based on genetic differences and growing conditions. Additionally, it has been observed that a salinity stress treatment leads to an increase in the sugar content in tomatoes (Sato et al., 2006; Flores et al., 2016; Marsic et al., 2018). The application of silicon alongside stress leads to an increase in both the TSS and sugar contents (Costan et al., 2020; Hu et al., 2023). Conversely, elevated silicon concentrations result in decreased glucose and fructose levels, even under conditions of water stress (Sánchez‐Rodríguez et al., 2012). From this evidence, it can be inferred that PRC influences the sugar content during the early stages of tomato development, with the potential effect of high concentrations persisting even with a 200-fold dilution of PRC. According to Pinedo-Guerrero et al. (2020), individual treatments of K2SiO3 and SiO2 NPs did not show significant effect on TSS. Likewise, Hu et al. (2023) reported no significant differences in glucose and fructose contents in tomatoes treated with 1.0 mM Na2SiO3. However, both studies observed an increase in the total protein content compared to the control, consistent with the findings here. An application of silicon to strawberry roots increased the protein content (Peris-Felipo et al., 2020), and in Zea mays, the protein content also increased with a nano-silica fertilizer treatment (Prihastanti et al., 2018). Application of PRC to radish and cabbage also resulted in a significant increase in the crude fat content in previous studies (Seo et al., 2014b, 2015). In our study, the application of PRC had a limited effect on the sugar content, but its positive effects on crude protein and crude fat synthesis are associated with the silicon and mineral components present in PRC.
Amino acid
The volatile compounds in tomatoes are derived from amino acids (Rambla et al., 2013), which are also associated with the plant’s defense response, and plants accumulate amino acids under stress conditions to enhance their stress resistance (Hayat et al., 2012; Sheng et al., 2017). Proline, in particular, is considered to be a stress indicator in plants and is primarily synthesized from glutamate during stress conditions (Delauney and Verma, 1993). Regulation of the proline content in plants by incorporating bacterial strains has been reported (Yoo et al., 2021). Additionally, Ullah et al. (2016) reported the protective effects of silicon and a mycorrhizal treatment on tomato plants. Salvioli et al. (2012) reported that the amino acid content can vary in tomato fruits during ripening and mycorrhizal colonization, also reporting the highest amino acid content in fruits treated with mycorrhizal. The increased levels of glutamic acid and aspartic acid in PRC are important for these amino acids in ripe fruits, while alanine and glycine contribute to sweetness, and phenylalanine and leucine enhance the bitterness of fruits (Solms, 1969; Zhu et al., 2018). Protein and amino acids have a significant impact on the taste and texture of fruits. Therefore, to improve the nutritional composition and quality of tomato fruits, the role of amino acids is crucial (Gremli, 1974; Snowden et al., 2015).
Soil chemical and microbial properties
Phosphate solubilization mechanisms by microorganisms typically involve acidification, the production of chelating metabolites, and redox activities (Lee et al., 2012). Phosphate solubility tends to increase with a decrease in the soil pH, as microbial activity coupled with the production of organic acids results in phosphorus solubilization (Satyaprakash et al., 2017; Kalayu, 2019). In a study by Kang et al. (2001), when phosphate-solubilizing microorganisms (Penicillium sp.) were cultured in rock phosphate media, the pH of the medium decreased and the addition of red clay reduced the pH further. However, the results of this study were different. These variations in phosphate solubilization mechanisms among microbial species can account for the differences observed (Lee et al., 2012). Although the exact mechanisms of phosphate solubilization between PRC and microorganisms are not well understood in this study, it is suggested that the co-application of PRC acted as a buffering agent to maintain a stable pH level. Phosphorus is an essential nutrient that plays a crucial role in various plant functions and in plant metabolism (Malhotra et al., 2018). Thus, ensuring an adequate supply of phosphorus is crucial for plants. An increase in the available phosphorus content in the soil is highly beneficial for plants and can positively impact their growth and productivity (Kumar et al., 2018).
Therefore, when treated with PRC, phosphorus acts as a buffer to maintain a stable pH level, which can positively impact crop growth and nutrient uptake in the soil (Schmidt et al., 2010; Dudás et al., 2017). In typical soils, phosphorus is fixed by cations such as Ca2+ and exists as complexes of calcium phosphate (Ca3PO4). In acidic soils, it forms aluminum phosphate (AlPO) and iron phosphate (FePO) by binding with Al3+ and Fe3+, respectively, resulting in their accumulation (Oliveira et al., 2009; Walpola and Yoon, 2012; Satyaprakash et al., 2017). Therefore, despite the abundance of inorganic and organic forms of phosphorus in the soil, they are mostly unavailable for plant uptake (Kalayu, 2019). The amount of phosphate that plants can utilize from applied phosphate fertilizers in the soil is typically low (Lee et al., 2012). In this study, the enhancement of Av. P2O5 in MFcL and PRC+MFcL can be attributed to the increased microbial influence. Soil microorganisms play a vital role in the transformation of insoluble phosphorus into soluble forms that can be readily utilized by plants, offering an environmentally friendly approach to improve phosphorus availability in the soil (Khan et al., 2014). These findings suggest that the combined application of PRC and MFcL during the cultivation of tomatoes can effectively benefit plants during their growth and development. In our study, a soil analysis after harvesting the tomatoes showed the highest content of Av. P2O5 in the MFcL and PRC+MFcL treated plots. In contrast, the NF soil showed a higher Av. SiO2 content, suggesting the potential absorption of Av.SiO2 by the plants. Therefore, further research is needed to understand the detailed mechanisms of how SiO2 functions physiologically during tomato cultivation (Sun et al., 2023).
Jung et al. (2014) reported that PRC promotes bacterial growth, which contradicts our findings. Indeed, PRC with a biofertilizer may have a synergistic effect to maximize the role of the biofertilizer to produce an environmentally friendly and sustainable crop (Shanthi, 2021). According to Jung et al. (2015), PRC, which contains only inorganic mineral compounds (i.e., SiO2, Al2O3, Fe2O3), exhibits unique characteristics as a biostimulant for bioremediation.
In conclusion, the PRC and MFcL treatments here had limited effects on the growth of tomato leaves and fruits. Both PRC and MFcL were found to be effective in reducing fruit cracking and producing more marketable fruits. The combined application of PRC and MFcL tended to increase the chlorophyll content. Additionally, PRC was found to increase the crude fat, crude protein and amino acid contents, which is beneficial considering that these components serve to produce high-quality tomato fruits. The soil analysis showed the highest content of available phosphorous and sufficient quantities of beneficial bacteria in the MFcL-treated plots; hence, the combined treatment of PRC and MFcL was considered to be effective for growing tomatoes in an open-field condition. This study provides a foundation for the utilization of microbial agents during tomato cultivation, though further research is recommended to explore the beneficial effects of PRC when used in conjunction with other microorganisms during the production of vegetable crops.