Introduction
Materials and Methods
Phylogenetic Analysis and Co-localization of QTL with IQD genes
Full-length cDNA Cloning of Cla011257
Transcriptome Analysis of Cla011257
Analysis of Allelic Variation Between ClSUN8 (Cla011257) and CmSUN14 (MELO3C015418)
Morphological Analysis of Watermelon Fruit
Results and Discussion
Phylogeny of the IQD Family Genes in Cucurbits
Co-localization of Fruit-shape QTLs with the IQD Family Genes
Detection of Allelic Variations for Fruit Shape-related IQ Genes
Morphological Assay and Expression Analysis of ClSUN8
Introduction
Fruit shape is an important horticultural trait that is a parameter in sensory evaluation for breeding. The size and shape of the fruit determine yield, consumer preference, packaging, and transportation. The molecular basis for the diversity in fruit shapes remains unclear. Cucurbitaceae crops, including watermelon (Citrullus lanatus), melon(Cucumis melo), cucumber (Cucumis sativus),and squash plants(Cucurbita spp.) (Pitrat, 2008; Sadrnia et al., 2007), have a diverse array of fruit shapes and are ideal for studying fruit shape.
Intensive genetic studies of fruit morphology have been performed in melon, watermelon, and cucumber. At least five genetic maps have been constructed for melon fruit shape, and a total of 42 QTLs, demonstrating a range of R2 values from 10.0% to 52.2%, have been detected. Among these QTLs, three major QTLs with R2 values higher than 30% (FSQQ2.2, 52.2%; FSQS8.1, 41%; FSQA9.1, 33%) have been found in three different chromosomes (Chr. 2, 8, and 9, respectively) (Périn et al., 2002; Monforte et al., 2004; Eduardo et al., 2007; Paris et al., 2008; Harel-Beja et al., 2010; Díaz et al., 2014). Similarly, at least 13 QTLs for fruit shape have been detected in watermelon from five genetic maps. The R2 values for these QTLs ranged from 2.4% to 79.7% and three major QTLs located on Chr. 3 and 11 showed R2 values higher than 30% (fsi3.1, 79.7%; FSI3.2, 38.22%; fsi11.1, 56.6%) (Sandlin et al., 2012; Ren et al., 2014; Kim et al., 2015; Cheng et al., 2016; Liu et al., 2016). One major QTL (fsi3.1) in watermelon was highly correlated with a homolog (Cla011257) to the SUN gene responsible for elongated fruit shape in tomato (Rodríguez et al., 2011; Huang et al., 2013). This SUN homolog was reported as a candidate gene for the fruit shape index (FSI) trait in watermelon (Kim et al., 2015). With the exception of tomato, no genes identified for directly conferring fruit shape-related traits have been cloned in major fruit crops, including cucurbits (Xiao et al., 2008).
The four fruit-shape genes cloned in tomato include: SUN for elongated fruit shape (Xiao et al., 2008), OVATE for pear and ellipse fruit shape (Liu et al., 2002), and LC and FAS for locule number (Cong et al., 2008).Various fruit shapes found in tomato germplasm are highly related to the modification of these four cloned QTLs and their composition in individual genotypes (Huang et al., 2013). The SUN gene for fruit length elongation belongs to the IQ67 domain gene family. The IQ67 domain is a plant-specific domain of 67 conserved amino acids and is characterized by an IQ domain (IQD) (Abel et al., 2005). The IQD mediates calmodulin (CaM) retention in a Ca2+- independent manner (Bähler and Rhoads, 2002; Hoeflich and Ikura, 2002) and regulates the growth and metabolism of plants (Feng et al., 2014; Abel et al., 2005). The IQD gene family has been analyzed across the plant kingdom, including Arabidopsis thaliana (Bürstenbinder et al., 2013), Glycine max (Feng et al., 2014), Oryza sativa (Abel et al., 2005), Populous trichocarpa (Ma et al., 2014), and Moso bamboo (Wang et al., 2016).
In this study, we identified IQD gene homologs in major cucurbit crops and assessed the phylogenetic relationships among them. Further, we co-localized these homologs with the fruit shape QTLs to explore their roles in determining fruit morphology. Sequences and gene expression of two IQD genes in watermelon and melon were characterized, and the results are discussed herein.
Materials and Methods
Phylogenetic Analysis and Co-localization of QTL with IQD genes
The sequences of Arabidopsis IQD genes were obtained from The Arabidopsis Information Resource (TAIR) (http:// www.arabidopsis.org). Homologs of these IQD genes in other species were found using BLASTP searches (E-value of 10-5) in the genome databases of the International Cucurbit Genomics Initiative (ICuGI) (http://www.icugi.org) for watermelon and cucumber, Melonomics (http://www.melonomics.net) for melon, and Sol Genomics Network (http://www.solgeno mics.net) for tomato. The pairwise genetic similarity coefficients among the genes were estimated based on the protein sequences using the Jones-Thornton-Taylor (JTT) method (Jones et al., 1992). The phylogenetic dendrogram was constructed for IQD proteins using MEGA 5.0 (Tamura et al., 2011) based on the neighbor-joining method and bootstrap analysis was conducted using 1,000 replicates.
For co-localization of the IQD genes, we searched for fruit shape QTLs based on previous QTL mapping studies in watermelon (Sandlin et al., 2012; Kim et al., 2015; Cheng et al., 2016; Liu et al., 2016), melon (Díaz et al., 2011; Díaz et al., 2014), and cucumber (Weng et al., 2015). PCR primer sequences flanking the fruit shape index (FSI) QTLs at the closest vicinity were obtained from previous publications (Périn et al., 2002; Monforte et al., 2004; Eduardo et al., 2007; Paris et al., 2008; Harel-Beja et al., 2010; Sandlin et al., 2012; Díaz et al., 2014; Ren et al., 2014; Kim et al., 2015; Cheng et al., 2016; Liu et al., 2016) and BLAST searches to the watermelon (97103 genome version 1), melon (DHL92 genome version CM3.5), and cucumber (Chinese long genome version 2) reference genomes to identify their physical genomic locations. Similarly, the physical locations of the IQD homologs identified as described above were compared for co-localization with the QTLs for FSI in the three cucurbit crops.
Full-length cDNA Cloning of Cla011257
Transcript sequence characterization of the candidate IQD gene Cla011257 for fruit shape was carried out with two watermelon accessions ‘Arka Manik’ (AM, round Crimson-type) and ‘TS34’ (TS, elongated Jubilee-type) that were the parental lines in our previous QTL mapping study (Kim et al., 2015). Each plant was grown in a growth chamber and fully expanded first true leaves were collected, directly frozen in liquid nitrogen, ground into powder, and stored at -80°C until use. Total RNA was extracted using the RNeasy Plant Mini Kit (QIAGEN, Germantown, MD, USA) and checked for quality using the Agilent 2100 Bioanalyzer (Agilent Technologies Inc., Santa Clara, CA, USA). The full-length cDNA of Cla011257 was cloned by rapid amplification of cDNA ends (RACE) PCR using the Smarter RACE 5ʹ/3ʹ Kit (Clontech Laboratories, Mountain View, CA, USA) following the user manual. Plasmids were purified using the Exprep Plasmid kit (GeneAll, Seoul, Korea). Purified plasmids were digested by EcoRI and HindⅢ (10,000 U·mL-1, New England BioLabs Inc., Ipswich, MA, USA) and the insert DNA was checked on a 1.0% agarose gel. The insert DNAs were sequenced based on the dye-termination method by Genotech (Daejeon, Korea). Full-length cDNA sequences obtained were aligned using ClustalX 1.83 (Thompson et al., 1997).
Transcriptome Analysis of Cla011257
Seedlings of four watermelon accessions, SGR (highly elongated), TS (elongated), AM (round), and SBA (round), were grown in a growth chamber under the conditions 35°C / 14 h day and 22°C / 10 h night. Seedlings at the third or fourth true-leaf stage were transplanted in a plastic greenhouse at Pusan National University (Miryang, Korea) until their fruit was harvested. Immature fruits (ovaries) were harvested at different developmental stages: -4 and -2 (four and two days before fertilization), and 0, 2, 4, and 6 days after fertilization (DAF). Due to the difficulties in determining the precise time of flowering, -4 and -2 DAF were determined based on the green and canary-yellow buds on the ovary, respectively, whereas the time of hand pollination was designated as 0 DAF. Immature fruits harvested at each developmental stage were immediately frozen in liquid nitrogen and stored at -75°C until use. Total RNA extraction was conducted as described in the previous section. cDNA syntheses from the RNA samples was conducted using the Hyperscript RT premix kit (GeneAll, Seoul, Korea) following the user manual.
For reverse transcription quantitative PCR (RT-qPCR), primers were designed from the coding sequence of Cla011257 (Table 1). The primers for the reference gene [β-Actin (Actin)] were obtained from Kong et al. (2014). RT-qPCR analysis was carried out using a Light Cycler 480 Real-Time PCR System (Roche Life Science, Basel, Switzerland) with the Light Cycler 480 SYBR Green I Master kit. All RT-qPCRs were performed in a total volume of 5 μL containing 1.25 μL of cDNA, 0.5 μL of forward and reverse primers (10 pm·μL-1), 2.5 μL master mix buffer, and 0.75 μL ddH2O. RT-qPCR conditions were: 1 cycle for pre-incubation at 95°C for 5 min; 45 cycles for amplification at 95°C for 10 s, annealing temperature of each primer set for 20 s, and extension at 72°C for 1 min; and 1 cycle for melting curve analysis at 95°C for 5 s, 65°C for 1 min, and continuous 97°C, followed by cooling at 40°C for 10 s. All RT-qPCRs were conducted with three replications and the average values were used for further analysis.
Analysis of Allelic Variation Between ClSUN8 (Cla011257) and CmSUN14 (MELO3C015418)
Partial genomic DNA sequencing of ClSUN8 (Cla011257) was carried out for four watermelon accessions “11-3” (elongated), “12-6” (elongated), “12-7” (round), and “42-2” (elongated). In our previous study (Jin et al., 2017), these inbred accessions showed failed PCR amplifications when tested by the Cla011257 gene-based dCAPS marker (Kim et al., 2015) and were speculated to carry another mutant allele (s) for Cla011257. For partial sequencing of Cla011257, new PCR primers (Table 1) were designed to encompass the primer locations of the dCAPS marker. In addition, genomic DNA sequencing of MELO3C015418 in melon was conducted from two melon accessions CM-P01 (oval cantaloupe melon) and MM-P02 (round musk-net melon). To clone MELO3C015418, the genomic DNA sequence was retrieved from the Melonomics database and PCR primers (Table 1) were designed at approximately 500 bp upstream and downstream of the gene.
All PCRs were performed in atotal volumeof 50 μL containing5 μL of genomic DNA (10 ng·μL-1), 2.5 μL each of forward and reverse primers (10 pm·μL-1), 5 μL of 10X PCR buffer, 1 μL of dNTPs (10 mM each), and 0.5 μL of Taq polymerase (5 U·μL-1) (SolGent, Daejeon, Korea). For plasmid vector cloning, the PCR amplicons were agarose-gel electrophoresed, Table 1. Primers used for gene expression, genotyping, RACE-PCR, and PCR cloning of IQD gene homologs in this study
Table 1. Primers used for gene expression, genotyping, RACE-PCR, and PCR cloning of IQD gene homologs in this study
![]() |
gel-purified using the Expin Gel kit (GeneAll, Seoul, Korea), and ligated using pGEM-T Easy Vector System I (Promega, Madison, WI, USA) following the manufacturers’ instructions. Cell transformation of the recombined plasmid vectors was carried out using HIT competent cells-DH5α (RBC, Taiwan, China) following the manufacturer’s instructions. Transformed cell colonies were amplified in liquid LB medium. Plasmid purification and subsequent detection and sequencing of the insert DNA were carried out as described in the section ‘Full-length cDNA cloning of Cla011257’ except that purified plasmids were digested by EcoRI (10,000 U·mL-1, New England BioLabs Inc., Ipswich, MA, USA).
Morphological Analysis of Watermelon Fruit
The immature fruit samples were harvested from the same four watermelon accessions [SGR (highly elongated), TS (elongated), AM (round), and SBA (round)] at -4, -2, 0, 2, and 4 DAF. Harvested fruit samples (three fruits per accession) were scanned using a SAMSUNG SL-C483W printer and the length and diameter of the fruits were measured using a vernier caliper (Mitutoyo, Kawasaki, Japan).
Paraffin sectioning was used for histological analysis of the fruits at different developmental stages. Harvested fruit tissues were fixed in FAA (5 mL formaldehyde, 5 mL acetic acid, 90 mL 50% ethanol, and 5 mL glycerol) for 24 h and then cleaned in 50% ethanol for 10 min. Cleaned samples were then sequentially soaked in 35%, 50%, 70%, 85%, 95%, and 100% (three times) ethanol and then finally in 100% xylene (three times) for 3 h each. The samples were then incubated in paraffin (Paraplast, Leica Biosystems, Solms, Germany) at 61°C for 3 h for three times, embedded in paraffin, and then sliced into 6μm sections using a Leica RM2235 microtome (Leica, Solms, Germany). The paraffin was removed using xylene and hydrated with 100%, 70%, and 35% ethanol, and then ddH2O for 3 min, and the tissues were stained using 0.1% toluidine blue solution
(0.1 g toluidine blue, 0.5 mL acetic acid, and ddH2O in a total volume of 100 mL). Finally, the tissues were dehydrated and sealed with Canada balsam (Sigma, Steinheim, Germany). A DM2500 microscope with a DFC295 camera (Leica, Solms, Germany) was used to observe the sections.
Results and Discussion
Phylogeny of the IQD Family Genes in Cucurbits
The IQD gene homologs in the three cucurbits and tomato were searched in BLASTP using protein sequences of 33 IQD genes of Arabidopsis. A total of 31, 29, 28, and 30 genes containing the IQ67 domain were retrieved for watermelon, cucumber, melon, and tomato, respectively. Previously reported IQD genes reported to be putatively responsible for fruit shape [Solyc10g079240.1.1 (SUN) for tomato (Xiao et al., 2008); Cla011257 for watermelon (Kim et al., 2015); and Csa1G575000 for cucumber (Pan et al., 2016)] were also confirmed to be among this gene family.
A phylogenetic dendrogram of the IQD genes was constructed by MEGA (Fig. 1). Each group showed a tendency to be composed of the IQD genes of different plant species. Considering that there is a high level of synteny among the genomes of cucurbit crops (Garcia-Mas et al., 2012), the genes of different species in the same clade may be orthologous and may have evolved from a common ancestral origin. In addition, a possible paralogous relationship was shown among the genes of the same species clustered under the same node. Further studies are necessary to explain the detailed evolutionary relationships among these IQD genes. Importantly, we identified from the dendrogram that two genes proposed as fruit-shape genes in
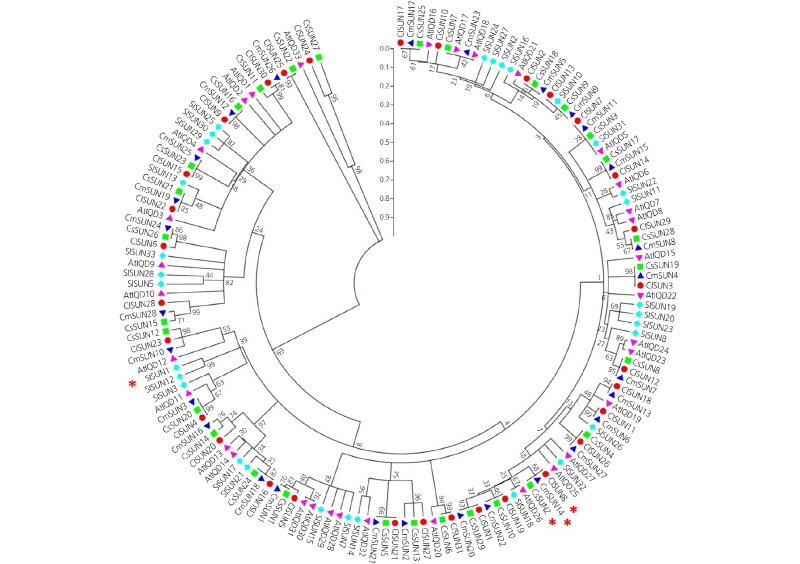
Fig. 1.
A phylogenetic tree of the IQD genes (SUN homologs) in Arabidopsis(purple triangle), watermelon (red circle), cucumber (green square), melon (blue triangle), and tomato (light blue diamond). The unrooted tree was constructed fromfull-lengthaminoacidsequences usingMEGA5.0basedonthe neighbor-joiningmethod.Theredasterisksindicate candidate IQD genes for fruit shape revealed by QTL mapping in tomato, watermelon, melon, and cucumber.
watermelon [ClSUN8 (Cla011257)] and cucumber [CsSUN2 (Csa1G575000)], and one melon IQD gene [CmSUN14 (MELO3C014258)] were grouped at the same node with a similarity of 0.97 (Fig. 1). However, the tomato fruit-shape gene [SUN (Solyc10g079240.1.1)] was distantly located from the genes in cucurbits. Subsequently, the molecular characteristics were analyzed for these four proteins.
Co-localization of Fruit-shape QTLs with the IQD Family Genes
The physical genomic locations of the IQD homologs in watermelon, melon, and cucumber are displayed on a schematized chromosome map (Fig. 2). The positions of watermelon IQD homologs were scattered on all chromosomes except for Chr. 11. The number of genes on each chromosome ranged from 1 for Chr. 3 to 7 for Chr. 5. Similarly, in melon, the genes were spread across all 12 chromosomes, with the number of genes ranging from 1 for Chr. 8 and Chr. 10 to 6 for Chr. 6.
The QTLs for fruit shape were found in previous QTL mapping studies on watermelon (Sandlin et al., 2012; Kim et al., 2015; Cheng et al., 2016; Liu et al., 2016), melon (Diaz et al., 2011; Díaz et al., 2014), and cucumber (Yuan et al., 2008; Bo
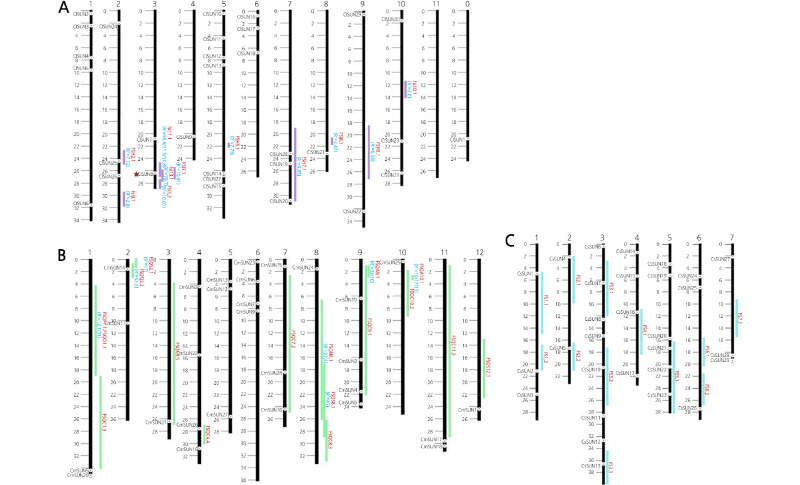
Fig. 2.
Schematicdiagramsofchromosomesshowingco-localizationoffruit-shapeQTLsandIQDgenes(SUNhomologs)in watermelon(A),melon(B),andcucumber(C).Physicaldistances onthe chromosomes are markedby1Mbintervals.The namesoftheSUN homologs(blacklettersanddotson thechromosomes)areshownon theleftsideofthechromosomes, while fruit-shape QTLs (red letters) are shown on the right side with their R2(%) values and genomic regions (flanking marker intervals).
et al., 2015) (Table 2). In watermelon, a total of 11 QTLs have been reported for fruit shape index, including the major QTLs fsi11.1 (Sandlin et al., 2012) and fsi3.1 (Kim et al., 2015) that were detected on Chr. 3. The IQD gene Cla011257 (named ClSUN8 in this study) (Kim et al., 2015) was physically located in the vicinity of both QTLs. In melon, a total of 16 QTLs have been reported for fruit shape index, including the major QTLs FSQQ2.2 (Périn et al., 2002) on Chr. 2 and FSQS8.1 on Chr. 8 (Díaz et al., 2014), and we found that the melon IQD homolog MELO3C015418 was located in the vicinity of FSQQ2.2. In cucumber, the length and diameter of ovaries and fruits were measured at anthesis (OvL and OvD), immature (FL and FD), and mature (MFL and MFD) stages and 22 QTLs were detected for the six traits (Weng et al., 2015). These fruit-shape (FS) QTLs were further integrated into 12 QTLs based on their location on the same chromosomal blocks (Weng et al., 2015). We confirmed that one of the cucumber IQD homologs, Csa1G575000 (CsSUN2), was located in the vicinity of FS1.2.
Co-localization of these major QTLs with IQD genes showed that Cla011257 was tightly linked to fsi11.1 and fsi3.1 in watermelon, MELO3C015418 to FSQQ2.2 in melon, and Csa1G575000 to FS1.2 in cucumber. In our phylogenetic dendrogram, these three IQD genes were clustered in the same subgroup, with a genetic similarity of 0.97 (Fig. 1). These results implied that IQD genes are not only associated with the fruit shape of cucurbit crops, but also show close orthologous relationships in these plant species.
Table 2. Fruit-shape QTL information that was used for colocalization of IQD gene homologs in cucurbits
![]() |
Watermelon FSH2.12 7.72 garden female parent x LSW-177 WCI02-7 WCI02-31 Cheng et al. 2016 fsi9.12 2.8 KBS x NHM NW0248574 NW0249974 Sandlin et al. 2012 fsi11.13 56.6/21.5 KBS x NHM NW0248107 NW0250956 Sandlin et al. 2012 fsi11.13 31.8 ZWRM x Citroides NW0248107 NW0250956 Ren et al. 2014 fsi3.13 79.7 Arka Manik x TS34 wsbin3-9 wsb3-24 Kim et al. 2015 FSI3.13 10.01 LSW-177 x COS WII03E02-87 WII03E09-92 Liu et al. 2016 FSI3.23 38.22 LSW-177 x COS WII03E09-92 WII03EXhoI-1 Liu et al. 2016 FSH5.15 7.75 garden female parent x LSW-177 WCI05-13 WCI05-14 Cheng et al. 2016 FSH7.17 9.85 garden female parent x LSW-177 WCI07-13 SSRW1-86 Cheng et al. 2016 FSI8.18 2.47 LSW-177 x COS WII08EKpnI-5 WII08EXhoI-5 Liu et al. 2016 FSH9.19 6.58 garden female parent x LSW-177 WCI09-8 WCI09-13 Cheng et al. 2016 fsi10.110 3.0 KBS x NHM NW0250299 NW0249853 Sandlin et al. 2012 Melon FSQP1.11 12.1 Ved x PI161 RIL CMCTN86 CMCCA145 Périn et al. 2002 FSQQ1.11 31 Ved x PI414723 RIL CMCTN86 CMCCA145 Périn et al. 2002 FSQC1.31 Piel de Sapo x PI161375 NIL CMCCA145 CMCTN4 Eduardo et al. 2007 FSQS2.12 17 Piel de Sapo x PI124112 NIL CMPSNP431 AluICAPS Díaz et al. 2014 FSQQ2.22 52.2 Ved x PI414723 RIL CMGA36 CmSUS1 Périn et al. 2002 FSQC3.53 Piel de Sapo x PI161375 NIL CSWCT10 TJ10 Eduardo et al. 2007 FSQC4.44 Piel de Sapo x PI161375 NIL CMAGN73 CMTC168 Eduardo et al. 2007 FSQC7.27 Piel de Sapo x PI161375 NIL CMAGN75 CMGA15 Eduardo et al. 2007 FSQN8.18 22.4 PI414723 x Dulce RIL CMCTTN232 CMAT141 Harel-Beja et al. 2010 FSQS8.18 41 Piel de Sapo x PI124112 NIL GCM241 PSI_25-H03 Díaz et al. 2014 FSQC8.38 Piel de Sapo x PI161375 NIL CMAT141 CMTCN56 Eduardo et al. 2007 FSQA9.19 33/10 Piel de Sapo x PI161375 DHL CMTC47 CM98 Monforte et al. 2004 FSQC9.19 Piel de Sapo x PI161375 NIL CMTC47 CMCTN7 Eduardo et al. 2007 FSQA10.110 10/17/13 Piel de Sapo x PI161375 DHL CMGA172 CMTCN67 Monforte et al. 2004 FSQC10.210 Piel de Sapo x PI161375 NIL CMCTN19 CMTCN8 Eduardo et al. 2007 FSQC11.211 Piel de Sapo x PI161375 NIL CMCT160a CMGA104 Eduardo et al. 2007 FSQS12.112 Piel de Sapo x PI124112 NIL AI_35-A08 CMPSNP361 Díaz et al. 2014 Cucumber FS1.11 Gy14 x9930 SNP.3689 SNP.118881 Weng et al. 2015 FS1.21 Gy14 x9930 SNP.11145 SNP.12981 Weng et al. 2015 FS2.12 Gy14 x9930 SNP.134265 SNP.121897 Weng et al. 2015 FS2.22 Gy14 x9930 SNP.149645 SNP.107609 Weng et al. 2015 FS3.13 Gy14 x9930 SNP.122373 SNP.35965 Weng et al. 2015 FS3.23 Gy14 x9930 SNP.37745 SNP.45557 Weng et al. 2015 FS3.33 Gy14 x9930 SNP.22341 SNP.51329 Weng et al. 2015 FS4.14 Gy14 x9930 SNP.58133 SNP.61745 Weng et al. 2015 FS5.15 Gy14 x9930 SNP.70369 SNP.77021 Weng et al. 2015 FS6.16 Gy14 x9930 SNP.143873 SNP.91177 Weng et al. 2015 FS6.26 Gy14 x9930 SNP.168061 SNP.94685 Weng et al. 2015 FS7.17 Gy14 x9930 SNP.166749 SNP.106789 Weng et al. 2015
Detection of Allelic Variations for Fruit Shape-related IQ Genes
In our previous study (Kim et al., 2015), the genomic DNA sequence of CISUN8 (Cla011257) revealed two SNPs (T to C and A to G) between lines TS (elongated fruit) and AM (round fruit), and a dCAPS marker was developed from one of these SNPs (A to G). In the present study using RACE PCR, we sequenced the full-length cDNA of Cla011257 to identify any mutations at the transcription or post-transcription levels that may relate to the function of this gene (Fig. 3, SM. 1). Sequence alignment of the cDNA sequences from the TS and AM lines revealed a 24-bp insertion/deletion (InDel) at the 3ʹ-UTR and two SNPs at the 995th (T to C) and 1,179th (A to G) nucleotides in the third exon of the open reading frame (ORF). The SNP at the 995th nucleotide was synonymous (Ser203), whereas the SNP at the 1,179th nucleotide was non-synonymous, switching Lys (basic) acid in the TS line (Lys265) to Glu (acidic) in the AM line (Glu265) (Fig. 3, SM. 1), as reported by Kim et al. (2015). No significant changes at the transcriptional level, such as alternative splicing, were observed.

Fig. 3.
The full-length cDNA sequences of ClSUN8 (Cla011257), a candidate IQD gene for fruit shape in watermelon accessions ‘TS34’ (TS, elongated) and ‘Aarka Marnik’ (AM, round). The 5’UTR and 3’UTR are shown in gray text. The nucleotide positions in the cDNA are marked by red asterisks. Mutation sites in the AM line [two SNPs in the coding sequence(CDS)and a25-bp deletionin the3’UTR]areshownin redtext.A deletionregionobservedinseveralwatermelon accessions,which resulted in failedPCR amplification of the Cla011257-dCAPS marker, isshadedina graybox.The information for the complete sequence of the full-length cDNA sequences of ClSUN8 (Cla011257) is provided in Supplementary material 1.
In our previous report (Jin et al., 2017), allelic variations for ClSUN8 (Cla011257) that may exist in the watermelon population were evaluated by genotyping 85 watermelon accessions using the dCAPS marker developed for the non-synonymous SNP (A to G). We found that 81% of the accessions showed a marker-phenotype match, whereas failed PCR amplification was observed for 10 accessions. Those results indicated that there are allelic variations in Cla011257. Therefore, in the present study, DNA sequences of the PCR primer region for the dCAPS marker were verified by partially cloning Cla011257 from the four accessions. The new primer set [ClSUN8 (SCAR)] (Table 1) flanking the primers of the dCAPS marker amplified DNA fragments of expected size from all four accessions, and sequencing of the PCR fragments indicated that there was a 159-bp deletion that included the forward primer site of the dCAPS marker (SM. 1). Because the accessions showed a deletion mutation in Cla011257 regardless of fruit shape, fruit shape of these accessions may be controlled by another locus (or other loci), that is (are) possibly related to another IQD gene member (s) on different genomic region (s). This supposition may also apply to other watermelon accessions that showed mismatches between the dCAPS marker genotype and fruit shape (Kim et al., 2015).
In this study, we also cloned the genomic sequences of MELO3C015418 from the melon inbred lines CM-P01 (oval) and MM-P02 (round) to identify allelic mutations. However, sequence alignment revealed no polymorphisms (data not shown). Although we cannot rule out the possibility that there may be mutations in the untranslated regions (UTR) and / or the promoter region of MELO3C015418, this sequencing result indicateed that a direct allelic comparison of the MELO3 C015418 gene between the parents (Ved × PI414723) that were used for QTL mapping (Périn et al., 2002) must be examined in order to elucidate the relationship between the IQD gene and fruit shape in melon.
The diversity of fruit shapes in cucurbits reflects genetic complexity and involvement of multiple genes in determining the trait. In addition, the loci conferring fruit shape may be different depending on the genetic background of the specific cultivar, as pointed out by Rodríguez et al. (2011). For example, different major QTLs were found in different mapping populations in melon [CMFSI2.2 on Chr. 2 from ‘Ved ×PI414723’ (Périn et al., 2002) and CMFSI8.2 on Chr. 8 from ‘Piel de Sapo × PI124112’ (Díaz et al., 2014)].
Morphological Assay and Expression Analysis of ClSUN8
The immature fruits (ovaries) at -4, -2, 0, 2, and 4 DAF were obtained from four watermelon inbred lines, SGR (highly elongated), TS (elongated), SBA (round), and AM (round), and their longitudinal length and diameter were measured (Fig. 4). At -4 DAF, the fruit shape index (FSI, length to diameter ratio) were SGR (FSI = 2.40), TS (1.89), AM (1.45), and SBA (1.37). All fruits rapidly expanded at 2 and 4 DAF (Fig. 4A). The FSI of each accession did not change significantly depending on the number of DAF, remaining constant from -4 to 4 DAF (Fig. 4B), implying that fruit shape had already been determined at the early stage of ovary formation.
Three different regions (proximal, middle, and distal) of the dissected fruit were observed (Fig. 5A). The size of the cells in these regions gradually expanded from -4 to -2 DAF (Fig. 5B). However, the cell sizes at any stage were very similar, indicating that the elongated fruit shape of SGR and TS was mainly due to a higher number of cells with increased cell division in the longitudinal direction (Fig. 5B). Extensive longitudinal growth during the early fruit developmental stages resulted in an elongated fruit shape of a mature tomato (Wu et al., 2011). In tomato, overexpression of the SUN gene ledto the appearance of extremely long fruit and an increased FSI. A morphological analysis of SUN-overexpressing transformants
showed that accelerated cell division in the longitudinal direction and decreased cell division in the transverse direction was responsible for the elongated tomato fruits (Wu et al., 2011).
To investigate a possible association between the IQD gene expression level and fruit shape in watermelon, the mRNA transcript levels of ClSUN8 were examined at different periods of fruit development (-4, -2, 0, 2, 4, and 6 DAF) for the SGR, TS, SBA, and AM lines (Fig. 6). The expression level of ClSUN8 gradually decreased in all accessions, indicating that the
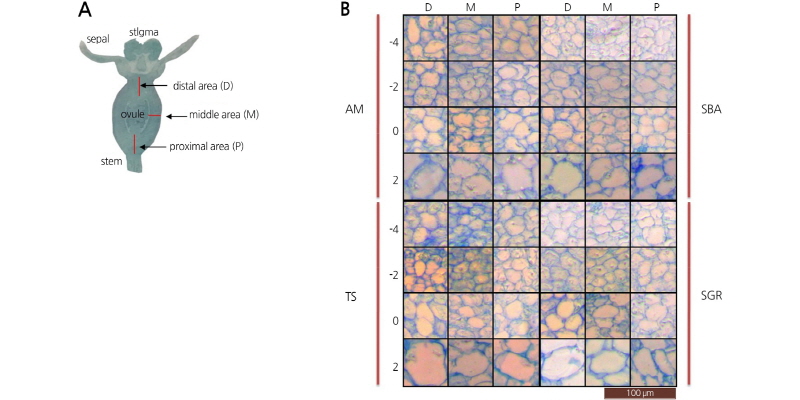
Fig. 5.
Longitudinal section diagram of immature watermelon fruit (A) and histological analysis of flesh cells at three different areas [distal (D), middle (M), and proximal (P)] of immature fruits harvested at -4, -2, 0, 2, and 4 days after fertilization (DAF) in four watermelon accessions (AM, TS, SBA, and SGR).
regulation of gene expression for fruit shape determination occurs in the early developmental stages of ovary formation. In addition, there was no direct relationship between the gene expression level and fruit shape (Fig. 6). If ClSUN8 is the gene responsible for fruit shape, our results imply that the elongated fruit shape of the TS line and the round shape of the AM line is not due to variations in the regulation of gene expression, but to the non-synonymous SNP and its effect on the molecular function of the IQD gene. To verify this hypothesis, a transformation confirmation test for functional characterization of ClSUN8 will be necessary.