Introduction
Materials and Methods
Preparation of Leaf Explants
Callus Induction
Subsequent Plant Regeneration
Direct Microtuberization by One-Step Culture
Growth and Propagation of Microtubers in Soil
Statistical Analysis
Results
Callus Induction
Subsequent Plant Regeneration
Direct Microtuberization by One-Step Culture
Growth and Propagation of Microtubers in Soil
Discussion
Introduction
Pinellia ternata (Thunb) Breit is an herbaceous perennial plant belonging to the Araceae family and widely native in Northeast Asia. It is an important medicinal herb in Korea, China, and Japan. The dried tuber of original P. ternata (Pinellia Rhizoma, Korean name “Banha”) has been used traditionally for curing coughing and dampness-phlegm, relaxing, dizziness, headache, and acute gastritis as a Korean herbal medicine. Pinellia Rhizoma has modern pharmacological effects, such as an anti-cancer effect, anti-obesity effect, emetic and anti-emetic effect, neuroprotective effect, and anti-stress effect (Kurata et al., 1998; Lim et al., 2009; Jie et al., 2015).
Recently, consumption of Pinellia Rhizoma has been increasing through mixed herbal formulas containing Pinellia Rhizoma extracts with the development of Asian medicine-derived systemic treatments (Park and Seo 2000; Lim et al., 2009). The artificially cultivated Pinellia Rhizoma is mainly circulating in herbal medicine markets because wild Pinellia Rhizoma is expensive. Nevertheless, products of Pinellia Rhizoma are insufficient compared to consumption in the Korean herbal medicine market. Most Pinellia Rhizoma is now being imported from China. Imports are increasing year by year. Also, the small rhizome of diverse plant species belonging to the other Arisaema species has been incorrectly used as Pinellia Rhizoma, which has greatly affected the quality of the herbal medicine (Lin et al., 2006; Jing et al., 2019).
P. ternata can be propagated both sexually seeds and asexually bulbils, but it is mainly depended on the bulbils in nature (Gu et al., 1994; Kim et al., 2005a; Luo et al., 2014). In natural conditions, the flowering rate is very low (Gu et al., 1994), because it is overlapping with the rainy season in Korea (Kim et al., 2005a). A mature plant of P. ternata produces only 7-8 bulbils per year (Peng et al., 2007). P. ternata is commercially cultivated using the small tubers without commercial value after harvesting. Tissue culture techniques has been used as an efficient protocol for vegetative propagation, because of poor reproduction in P. ternata. Callus induction and plant regeneration of P. ternata have been performed with explant sources, culture media, and combinations of plant growth regulators using a two- or three-step culture system by many researchers (Choi and Rha 1986; Kim et al., 1994; Kim et al., 2005a; Xu et al., 2005; Eun et al., 2015; Zhang et al., 2017). The two- or three-step protocol consists of as a first step, the induction of callus formation, the second step morphogenetic or embryogenetic induction and the third step organogenetic differentiation. Each phase require different hormone and takes long time to product in vitro organs (Komal, 2011). While the one-step protocol is that in vitro organs are directly induced from explant tissues. The one-step culture system reduces the cost and time of in vitrovegetative propagation. In vitro microtuber production of P. ternata has been accomplished with explant sources, gelling agents, and combinations of plant growth regulators by the one-step culture system (Xue et al., 2006; Kim et al., 2007; Peng et al., 2007). In particular, in vitro propagation of medicinal plants is essential to produce circularly without somaclonal variation. Somaclonal variation derived from tissue cultures can cause problems with the genetic uniformity of the regenerated plants and lead to subsequent negative efects on the use of tissue culture (Park et al., 2020). However, the protocols of previous researches were economically critical for use in the plant factory system. We designed a simple protocol to develop the new one-step culture system without medium renewal in the micropropagation of P. ternata.
The present work aimed to develop a simple and rapid micropropagation method for applying the plant factory system. Firstly, we evaluated the effects of growth regulators on the sustainable production of in vitro leaf explants of P. ternata. Secondly, the effect of growth regulators on direct microtuberization of whole leaf explants was evaluated in one-step culture protocol without medium renewal. Thirdly, the growth of microtubers produced through one-step culture was estimated in soil cultivation.
Materials and Methods
Preparation of Leaf Explants
Tubers of Pinellia ternata were collected from the Medical Plant Garden at Wonkwang University in Korea, and tubers’ removed leaves were grown under greenhouse conditions with 50% shade in plastic pots. Newly tender leaves were taken as explant materials from regrown plants on May 25, 2018. The leaf explants were surface-sterilized for 10-15 second in 70% EtOH (v/v), then rinsed twice with sterile distilled water on a clean bench. Furthermore, the leaf explants were sterilized for 20 min. in 0.5% NaOCl (v/v, Samchun Chemical Co, Ltd., Seoul, Korea) with 2 drops of Tween-20 (Daejung Chemical and Metal Co, Ltd., Korea), followed by rinsing three times with sterile distilled water. Finally, leaf explants were transferred to sterile Petri dishes and cut into small pieces (5 × 5 mm) with the removal of the main vein.
Callus Induction
For callus induction and proliferation, leaf segments were cultured on MS (Murashige and Skoog, 1962) media, gelled 0.3% (w/v) Phytagel (Sigma Chemical Co. Ltd., USA), and supplemented with different levels of 2,4-D (2,4-dichlorophenoxy acetic acid, Sigma Chemical Co. Ltd., USA) and BA (Benzyl amino purine, Sigma Chemical Co. Ltd., USA). The media pH was adjusted to 5.8 before autoclaving at 121°C for 20 min. The leaf segments were plated in each Petri dish (100 × 15 mm), for a total of 50 explants per medium. The Petri dishes were sealed with parafilm (60 × 15 mm, Whatman Ltd.) and were incubated in the dark at 25 ± 1°C for 5 weeks. Callus induction frequency and fresh weight were investigated in media containing different concentrations and combinations of growth regulators at 5 weeks in the culture (Table 1).
Table 1.
Effects of 2,4-D and BA on callus induction and growth from leaf explants of P. ternata
Growth regulators (mg·L-1) | No. of explants | Callus induction (%) |
Fresh weight (mg·explant-1) | |
2,4-D | BA | |||
0.5 | 0.0 | 50 | 36.0 dz | 12.8 e |
0.2 | 50 | 70.0 c | 24.8 cd | |
0.5 | 50 | 84.0 b | 25.8 bcd | |
1.0 | 0.0 | 50 | 46.0 d | 20.6 d |
0.2 | 50 | 88.0 ab | 28.2 bc | |
0.5 | 50 | 92.0 ab | 30.4 b | |
2.0 | 0.0 | 50 | 68.0 c | 26.6 bc |
0.2 | 50 | 98.0 a | 40.8 a | |
0.5 | 50 | 94.0 ab | 38.2 a | |
LSD 0.05 | 13.85 | 5.31 |
Subsequent Plant Regeneration
For plant regeneration, the calluses propagated in the medium supplemented with 2 mg·L-1 2,4-D and 0.2 mg·L-1 BA were transferred to 500 mL glass vessels containing MS media gelled 0.3% (w/v) Phytagel supplemented with different levels of NAA (Napthalene acetic acid, Sigma Chemical Co. Ltd., USA) and KIN (kinetin, Sigma Chemical Co. Ltd., USA). Six calluses were plated in each bottle (500 mL) for a total of 30 calluses per medium. The culture was incubated under a 16-h light photoperiod provided by cool white fluorescent lamps at a photon flux density of 27 µmol·m-2·s-1, at 25 + 1°C. The number of calluses producing shoots and the total number of shoots per callus were counted for each treatment at 6 weeks in the culture (Table 2). Also, the nature of callus proliferation was investigated in eight plant-regeneration media for subsequent callus proliferation and plant regeneration at the same time by a combination of plant growth regulators.
Table 2.
Effect of NAA and kinetin on plant regeneration from the callus derived from leaf explants of P. ternata
Growth regulators (mg·L-1) |
No. of cultured calluses | % of explants with shoots |
No. of plantlets per callus |
Nature of callus propagation | |
NAA | Kinetin | ||||
0.2 | 0.2 | 30 | 100 | 5.8 bcdz | -y |
0.5 | 30 | 100 | 6.4 bc | - | |
0.5 | 0.2 | 30 | 100 | 7.2 abc | + |
0.5 | 30 | 100 | 6.1 bcd | + | |
1 | 0.2 | 30 | 100 | 7.7 ab | + |
0.5 | 30 | 100 | 5.2 cd | ++ | |
2 | 0.2 | 30 | 100 | 8.8 a | +++ |
0.5 | 30 | 100 | 4.2 d | +++ | |
LSD 0.05 | 2.07 |
Direct Microtuberization by One-Step Culture
The leaves derived from the regeneration medium containing 2.0 mg·L-1 NAA, 0.2 mg·L-1 KIN were used as explant sources for mass production of microtubers by a one-step culture. Leaves of 10-15 mm in size were excised and immediately cultured on MS media gelled 0.3% (w/v) Phytagel supplemented with different levels of NAA and BA (Table 3). Twenty leaf explants were plated in each Petri dish (100 × 15 mm) for a total of 60 leaves per medium. The culture was incubated under the same condition in a plant regeneration environment for 10 weeks until the media were naturally dried without a subculture. The number of explants producing microtubers and the total number of microtubers per leaf explant were counted for each treatment at 10 weeks in culture.
Table 3.
Effect of NAA and BA on direct microtuberization from in vitro leaf explants of P. ternata
Growth regulators (mg·L-1) | No. of cultured explants |
Microtuberization (%) |
No. of teberlets (no.·explant-1) | |
NAA | BA | |||
0.5 | 0.2 | 60 | 27.0 dez | 1.0 e |
0.5 | 60 | 22.0 ef | 0.8 e | |
1.0 | 60 | 14.0 f | 0.7 e | |
1.0 | 0.2 | 60 | 62.0 b | 4.1 b |
0.5 | 60 | 41.0 c | 3.2 c | |
1.0 | 60 | 36.0 cd | 2.8 d | |
2.0 | 0.2 | 60 | 93.0 a | 6.1 a |
0.5 | 60 | 86.0 a | 4.3 b | |
1.0 | 60 | 63.0 b | 3.5 c | |
LSD 0.05 | 10.64 | 0.38 |
Growth and Propagation of Microtubers in Soil
Microtubers produced by the one-step culture were overwintered at room temperature at around 10-15°C. Fifteen microtubers (3 explants with 5 microtubers) were planted in plastic pots (Ø 110 × 100 mm) containing a commercial soil (TKS2, N-P-K : 330-220-400 mg·L-1, Korea Horticulture Materials Co. Ltd., Seoul, Korea) on April 20. In the greenhouse, the pots were cultivated under shade cloth (50% light reduction) with bottom watering every 3 days. Fertilizer was applied as a base dressing Urea (217 mg·L-1), fused superphosphate (500 mg·L-1), and potassium chloride (200 mg·L-1). After 6 months, the plant height, number of tubers per pot, and tuber size were investigated using three size ranges (> 7, 4-7, and < 4 mm diameter) to evaluate the production of seed tubers for application in a plant factory system.
Statistical Analysis
Each experiment was conducted in a completely randomized design with 5 replications. The data were calculated using SAS software Version 9.1 (statistical analysis system, Version 9.1, SAS Institute Inc., USA). Significant differences between means were determined using Duncan’s multiple range test (p < 0.05).
Results
Callus Induction
In plant tissue culture, the combination of auxin and cytokinin in the medium is vital for the commercial vegetative propagation of plants. Callus induction of P. ternata leaf explants was greatly influenced by growth regulators. After 2 weeks, inducing calluses could be observed at cross-sections of the leaf explants, and induced calluses were rapidly grown around 3 weeks after the culture. Thereafter, the growth of the calluses was affected by the combination of auxin and cytokinin. After 5 weeks, the percentage of callus induction was promoted in higher 2,4-D levels, and it was significantly higher in the combination media of 2,4-D and BA than with the application of 2,4-D alone (Table 1). The highest callus induction and propagation were produced in the MS medium supplemented with 2.0 mg·L-1 2,4-D and 0.2 mg·L-1 BA. MS media added with other growth regulator combinations also showed excellent callus induction capacities, such as 2.0 mg·L-1 2,4-D and 0.5 mg·L-1 BA as well as 1.0 mg·L-1 2,4-D and 0.5 mg·L-1 BA. The highest callus fresh weights were obtained in media supplemented with 2.0 mg·L-1 2,4-D and 0.2-0.5 mg·L-1 BA. A combination of 2,4-D and BA was also had a significantly higher fresh weight than 2,4-D alone. Further calluses were subcultured in the same MS media with 2.0 mg·L-1 2,4-D and 0.2 mg·L-1 BA for increasing the number of calluses for uses in the experiment of plant regeneration. Thus, callus induction from leaf explants of P. ternata was achieved using MS medium with combinations of auxin (2,4-D) and cytokinin (BA).
Subsequent Plant Regeneration
The calluses propagated in MS media containing 2.0 mg·L-1 2,4-D and 0.2 mg·L-1 BA were used as experimental materials for plant regeneration. Calluses were cultured in MS media containing the combinations of NAA and KIN under a 16-hour light photoperiod. After 1 week, many green spots appeared on the surface of light yellow- to light green-colored calluses, and nodular structures emerged soon from the green spots. Shoots were subsequently regenerated from the green nodules in 2-3 weeks, and leaves developed soon after shoot emergence. One to three roots per shoot were initiated in the media containing over 0.5 mg·L-1 NAA, and then shoots grew more vigorously. The percentage of shoot regeneration and number of shoots were estimated at 6 weeks after the culture (Table 2). Most of the cultured calluses showed well-developed shoots within 3 weeks. The combinations of NAA and KIN showed excellent shoot initiation in all tested regeneration media. The percentage of explants with shoots was 100% in all tested media. The number of plantlets per callus was affected by plant growth regulators, and significant differences in the number of plantlets per callus were found among the regeneration media tested.
The highest number of shoots (8.8 per callus) was regenerated from calluses cultured on MS medium containing 2.0 mg․L-1 NAA and 0.2 mg․L-1 KIN. Other regeneration media also showed good shoot regeneration without significant differences, such as 1.0 mg·L-1 NAA and 0.2 mg·L-1 BA as well as 0.5 mg·L-1 NAA and 0.2 mg·L-1 BA. In vitro regenerated plants grew about 7 cm in height after 6 weeks (Fig. 1A). Shoot growth was similar in all media, but the proliferation and quality of calluses varied, as shown in Table 2. The proliferation and quality of calluses as well as plant regeneration were excellent in the medium containing 2.0 mg․L-1 NAA and 0.2 mg․L-1 KIN. Accordingly, the calluses proliferated in the regeneration medium were replaced in a fresh regeneration medium supplemented with NAA 2 mg·L-1 and KIN 0.2 mg·L-1 for using as the leaf explant source for a subsequent plant regeneration system.
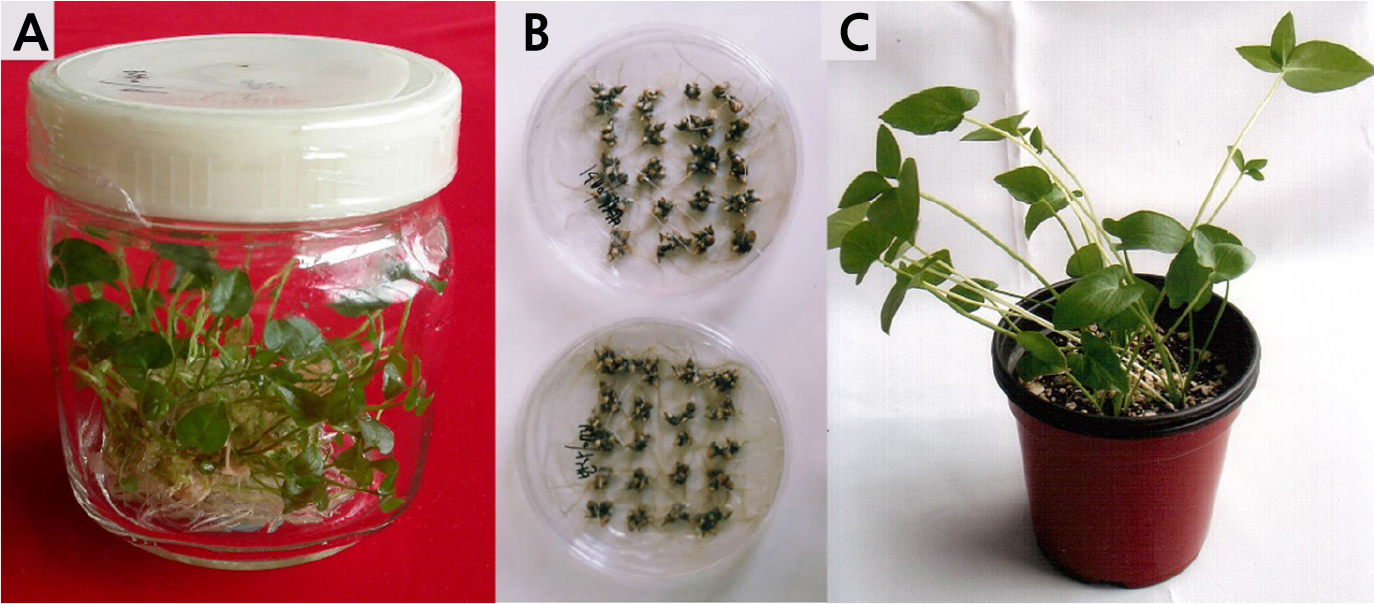
Fig. 1.
RT-PCR analysis using NeLV-specific primers to identify NeLV infection in the bulbs of Hippeastrum hybridum (upper panel); 18S rDNA amplification was used as an internal control (lower panel). M, 100-bp DNA ladder marker; 1-10, H. hybridum ‘Red Lion’; 11-15, H. hybridum ‘Alfresco’; (+), positive control; (‒), sterile distilled water instead of cDNA.
Direct Microtuberization by One-Step Culture
Direct microtuberization of P. ternata by the one-step culture was conducted to develop a new micropropagation system. The leaf explants regenerated in vitro (more than 1cm length) were placed on MS media gelled 0.3% (w/v) Phytagel containing different levels of NAA and BA without cutting into a small size. Microtubers with pale yellow to greenish color were initiated around 3 weeks after the culture, and those were developed to about 3-4 mm in diameter at 6 weeks after culture. The percentage of microtuber formation and the number of microtubers per explant were estimated at 6 weeks after culture (Table 3). The highest frequency of microtuber formation (93.0%) was achieved in the medium with a combination of 2.0 mg·L-1 NAA and 0.2 mg·L-1 BA. There was no callusing or sprouting in the microtubers derived from whole-leaf explants. The number of microtubers per explant was highest in the same medium (6.1 per explant). At the higher concentrations of BA media, there were significantly fewer of both the frequency of microtuber formation and the number of microtubers per explant.
The hormonal combination of BA and NAA was crucial for the direct microtuberization of whole -leaf explants of P. ternata. Whole-leaf explants were economically remarkable as an explant source for in vitro microtuber production of P. ternata. After 7 weeks, microtubers placed on the medium were spontaneously dried in the Petri dish removed Parafilm for 2 weeks and then preserved under room temperature for 4 months (Fig. 1B). Microtubers preserved in a Petri dish were used in the soil cultivation experiment.
Growth and Propagation of Microtubers in Soil
Microtubers produced in vitro were planted on April 20 in pots (Ø 110 × 100 mm), and the growth and propagation of microtubers after 6 months were investigated to evaluate the production of seed tuber for using in the plant factory system. Most microtubers were sprouted in 2-3 weeks after planting, and the average emergence rate was 81.5% after 1 month (Table 4). Plants sprouted in pots were vigorously grown without summer depression from July to August. The average plant height was 11.4 cm after six months (Fig. 1C). All plants had one to three leaves, and most of them developed one or two bulbils on the bases or tops of petioles in late September to early October. After 6 months, the average number of harvested tubers was 26.8 per pot, and the average number of tubers with a diameter of below 4 mm was 67.2%. The total number of tubers propagated was about 1.5 times for 6 months in the pot culture. The mean fresh weight of tubers harvested was 165.5 mg per tuber, and the mean fresh weight was higher by over 3 times in tubers of 4-7 mm than in tubers below 4 mm. Thus, tubers harvested in soil cultivation could be used as seed tubers in the field or plant factory.
Table 4.
Growth and propagation of plants grown from in vitro microtubers of P. ternata in pot culture
Discussion
In vitro micropropagation is an effective propagation method for large-scale industrial or commercial needs in asexual or vegetative propagation of plants. Micropropagation of P. ternate has been studied by many researchers (Choi and Rha, 1986; Kim et al., 1994; Kim et al., 2005a; Xu et al., 2005; Eun et al., 2015; Zhang et al., 2017). In vitro plant regeneration is very important as a key target in the process of micropropagation. Plant regeneration from explants can be achieved by direct or indirect organogenesis and embryogenesis. In the case of direct plant regeneration, in vitro organs are directly induced from explant tissues by one-step; in the case of indirect regeneration, in vitro organs are typically formed via callus induction by a two- or three-step process. One-step process of plant regeneration seemed to be a cost effective and shorter protocol (Tsay et al., 1989; Xue et al., 2006; Komal, 2011). One-step culture are widely used for spore germination, gametophyte proliferation, and sporophyte formation in Reridophyta (Jang et al, 2019a, 2019b). So we experimented to develop the simple protocol for direct microtuberization from leaf explants of P. ternata by one-step process without medium renewal.
Firstly, we evaluated the effects of growth regulators on the sustainable mass-production of in vitro leaf explants of P. ternata. We also tried to select the medium for subsequent callus proliferation and plant regeneration in the same medium. Callus induction was greatly influenced by explant sources and growth regulators. The callus induction of P. ternata was successfully achieved from various explants such as leaf, petiole, root, and tuber (Kim et al., 2005a; Xu et al., 2005; Jie et al., 2015). Callus induction from leaf explants was also higher than that from petiole (Kim et al., 2005b; Xu et al., 2005). Although Tsay et al. (1989) and Xu et al. (2005) reported that the best explant of P. ternata for promoting tuberization or callus induction was a tuber segment among leaf, petiole, and tuber, we found the leaf explant was more economic as an explant source because leaves can be cut in many segments and more readily collect in nature and in vitro. In this work, the callus induction and proliferation of P. ternata were investigated on several MS basal media containing different concentrations and combinations of 2,4-D and BA. Callus induction from leaf explants of P. ternata was associated with the best combination in the medium containing 2.0 mg·L-1 2,4-D and 0.2 mg·L-1 BA. Similar results had been reported in P. ternata by other researchers (Choi and Rha 1986; Kim et al., 2005a; Xu et al., 2005; Kim et al., 2007). Kim et al. (1994) reported that callus induction of the leaf explant was better in the media containing 2,4-D than NAA or IAA. Peng et al. (2007) also reported that no visible callus appeared in a medium without 2,4-D. The effective induction and proliferation of callus in P. ternata was achieved in the presence of 2,4-D, and showed more excellent results in the combination of 2,4-D and BA than 2,4-D alone. Thus, a suitable combination of 2,4-D and BA is required for callus induction and proliferation from leaf explants of P. ternata.
In the plant regeneration medium, a suitable combination of auxin and cytokinin was also required for organogenesis of P. ternata. This combination medium was important for cycling a subsequent callus proliferation and plant regeneration at the same time. BA, KIN, or zeatin added to the nutrient medium might stimulate shoot formation (Kim et al., 2005b; Xu et al., 2005; Jie et al., 2015). In this work, the subsequent callus proliferation and plant regeneration of P. ternata was associated with the best combination in the medium containing 2.0 mg·L-1 NAA and 0.2 mg·L-1 KIN (Table 2). In another study, the addition of NAA alone in the regeneration medium was found to inhibit regeneration (Jie et al., 2015). Also, 2,4-D was ineffective for induction of embryogenesis from leaf and petiole explants P. ternata, but a combination of NAA and BA promoted the frequency of embryogenesis (Kim et al., 2005b). We tried to establish the subsequent callus proliferation and plant regeneration system at the same time, and we successfully gained the leaf explants of plants regenerated in vitro year-round. Propagated calluses were possible maintaining the plant regenerating potential over 18 months after the subculture by Xu et al. (2005). In this study, high-frequency shoot regeneration and the maximum number of shoots per callus were obtained on MS medium containing 2.0 mg․L-1 NAA and 0.2 mg․L-1 KIN. The regenerated plants were used as the source of disease-free explants for direct microtuberization.
Secondly, we tried to establish the simple and rapid propagation method using direct tuberization of P. ternata by a one-step culture system. In vitro microtuberization of P. ternata has also been done with explant sources, gelling agents, and combinations of plant growth regulators by many researchers (Xue et al., 2006; Kim et al., 2007; Peng et al., 2007; Jie et al., 2015; Kim et al., 2020). The results of previous research were, however, transplanted the plants regenerated from in vitro microtubers into the soil. The regenerated plantlets demand a long time for acclimatization and growth in soil. We were established the production protocol of in vitro dried microtubers like an artificial seed by one-step culture without a renewal culture medium. The direct tuberization from whole-leaf explants of P. ternata was successfully achieved in a combination media of BA and NAA. In nature, the bulbil of P. ternata was formed in the lower part of petiole, and it started from the parenchyma cells in young leaves (Luo et al., 2014). The highest frequencies of microtuber formation (93.0%) and microtubers per explant (6.1) were achieved in the medium with a combination of 2.0 mg·L-1 NAA and 0.2 mg·L-1 BA. Although the direct organogenesis of P. ternata was possible in a medium containing NAA alone (Peng et al., 2007), the direct tuberization from explants without callus induction was largely affected by a higher cytokinin concentration than auxin (Kim et al., 2007; Eun et al., 2015). Jie et al. (2015) explained that cytokinins are the primary effector, and auxin plays a secondary role. In this work, the microtuberization from whole-leaf explants occurred through direct organogenesis without sprouting or callusing in the medium containing higher NAA concentration (2.0 mg·L-1) than BA (0.2 mg·L-1). We assumed that this result was affected by the endogenous hormones of leaf explants derived from the plant regeneration medium (containing 2.0 mg·L-1 NAA and 0.2 mg·L-1 KIN). The number of microtubers per explant was high at 8.8 per explant in the same medium (2.0 mg·L-1 NAA and 0.2 mg·L-1 BA), and the efficiency of the microtuber production was able to produce about 150-170 microtubers per Petri dish during 7 weeks (Fig. 1). Tsay et al. (1989) reported that the explants directly produced adventitious buds in a solid medium containing 2 mg·L-1 BA and 0.2 mg·L-1 NAA, and claimed that 1.7 × 1027 plantlets in one year could be produced from a single bulbil through protocom-like bodies and adventitious shoots without callus induction. Microtubers induced in vitro did not sprout for up to 6 weeks. Microtubers were spontaneously dried from 7 weeks, and the adequate dried microtubers were used as a seed tuber in soil cultivation.
Thirdly, we tried the soil cultivation of the dried microtubers produced by one-step culture like an artificial seed. The dried microtubers were seeded in plastic pots (Ø 110 × 100 mm) containing a commercial bed soils. The microtubers sprouted in 2-3 weeks after planting in soil, and the average emergence rate was 81.5% after 1 month (Table 4). After 6 months, the total number of tubers propagated was about 1.5 times that in the pot culture, and harvested tubers could be used as seed tubers for field cultivation in the next year. Although the fresh weight of harvested tubers was low in the pot culture (mean 165.5 mg), we assumed this was because of the small size of the pot (Ø 110 × 100 mm) culture and the high temperature in the greenhouse. If in vitro dried microtubers were cultivated in the plant factory, and this protocol could be produced the high-quality seed tubers of P. ternata for commercial applications.
In a typical tissue culture experiment, the protocol consists of, as the first step, the callus induction, the second step, morphogenetic/embryogenetic induction, and the third step, plant differentiation and transplanting into soil. Each step requires different growth regulators and takes about 8 weeks to achieve a sufficient result (Komal, 2011). We guessed that this one-step protocol was more simple and economic results for the commercial applications of P. ternate.
In conclusion, in vitro micropropagation by one-step culture could reduce the cost and time in the seed tuber production of P. ternata with low propagation in nature. We conclude that the one-step microtuberization protocol without medium renewal by in vitro cycling system was established for the mass production of seed tubers from P. ternata. In this cycling system, the main step was to establish the direct microtuberization system without medium renewal during a short period of time. The direct microtuberization from whole-leaf explants of P. ternata could be successfully achieved in the combination media of BA and NAA. This system needed a process of callus proliferation and plant regeneration to achieve a sustainable supply of disease-free leaf explants. The sustainable supplying of in vitro leaf explants was possible in the plant regeneration medium containing NAA and KIN. Thus, we established the subsequent callus proliferation and plant regeneration system in the same medium, and the in vitro whole leaf explants with no disease were successfully supplied for microtuberization all year-round. Furthermore, the direct microtuberization by the one-step culture without medium renewal is suitable for mass producing seed tubers of P. ternata in the plant factory system. The results could lead to reduced cost and time to farmers in the commercial production of P. ternata.