Introduction
Materials and Methods
Test strains
Experiment 1. Establishment of early diagnosis testing for leaf blight resistance
Experiment 2. Histological characteristics of leaves
Experiment 3. Disease progression after artificial inoculation in the shaded field
Experiment 4. Correlation between disease progression and microclimate conditions after disease development in the shaded field
Experiment 5. Yield reduction resulting from reduced pesticide application
Statistical analysis
Results
Development of early diagnosis testing for determining resistance to leaf blight
Difference in the histological characteristics of leaves between resistant and susceptible cultivar
Correlation between leaf blight development and weather conditions in the shaded field: Comparison between natural development and artificial development after inoculation
Yield discrepancies resulting from reduced pesticide applications
Discussion
Development of early diagnosis testing for leaf blight resistance
Relationship between histological characteristics of the leaves and resistance
Correlation between leaf blight progression and microclimate conditions in a shaded field: Comparison between natural development and artificial development after inoculation
Yield reductions due to decreased pesticide applications
Conclusion
Introduction
After being seeded, Korean ginseng (Panax ginseng C.A. Meyer) is cultivated in the same location for over four years. If a disease develops even once within this period, it will affect the crop year after year, requiring significant effort and expense to address.
Diseases that harm the above-ground part of ginseng include Alternaria leaf blight (Alternaria panax), anthracnose (Colletotrichum gloeosporioides), and gray mold (Botrytis cinerea). Among them, Alternaria leaf blight affects the stems, leaves, and fruits from April to late August, although it rarely harms the roots (David, 1988; Kim et al., 2010; Kawakatsu and Fukuda, 2023). It has been reported that the occurrence of Alternaria leaf blight reduces the yield of Korean ginseng by 30% and American ginseng (American quinquefolius L.) by 10 to 20% (Hill and Hausbeck, 2008; Cho, 2009; Kim et al., 2010).
Meanwhile, the most significant threat associated with the application of pesticides is the emergence of chemical-resistant strains. In the US, the emergence of iprodione (Ipr)-resistant Alternaria isolates was first reported in 1987 (Rahimian, 1987). According to earlier work, among the pesticides used for leaf blight, including pyraclostrobin (Pyra), fludioxonil (Flu), cyprodinil (Cyp), and difenoconazole (Dif), Flu and Cyp achieve half the maximal effective concentration (EC50) at a dose of 10 μg/mL or higher against five pathogens and against one pathogen, respectively (Rahimian, 1987; Avenot and Michailides, 2015). Additionally, in Korea and China, there have been reports on the emergence of bacteria resistant to Carbendazim (Car), Ipr, Pyrimethanil (Pyri), and Azoxystrobin (Azo), which are pesticides for gray mold in ginseng (Kim et al., 2009; Lu et al., 2016).
To address these challenges, various agronomic control approaches have been proposed, including environmentally friendly control methods using lime Bordeaux mixtures and Bacillus suspensions, strategies to enhance ventilation in shaded areas, and the removal of plants affected by leaf blight. However, these methods are generally considered less efficient compared to chemical agricultural pesticides approaches (Cheon et al., 2003; Cho, 2009; Kim et al., 2010; Kim and Park, 2013; Yoon et al., 2018).
Moreover, with the growing recognition of carbon neutrality across the globe, there have been increased efforts to reduce carbon emissions in the agricultural sector. Producing 1 kg of ginseng results in carbon emissions of 4.23E+00 kgCO2 eq. kg-1. Notably, 25% of this carbon footprint is attributed to pesticide use (Jung et al., 2011). It has recently been recommended to use cultivars with enhanced resistance in potato production as a measure to mitigate carbon emissions (So et al., 2010a).
On the domestic front, the positive list system (PLS) has been implemented to prevent the excessive application of pesticides (Song et al., 2021). With the implementation of the PLS, any agricultural produce that exceeds the established pesticide residue limits or is found to contain non-registered pesticides due to unintended dispersed contamination should be prohibited from distribution (Wang et al., 2018).
Given these environmental and societal conditions, it is now crucial to develop new ginseng cultivars with enhanced resistance to leaf blight. In the development of disease-resistant cultivars, early diagnosis testing has a critical role in reducing both the costs and time required. The development of early diagnosis testing, specifically tailored to address grape phomopsis blight and rice blight, has contributed to reducing the time required for developing new cultivars with enhanced resistance (Park et al., 2006; Challagulla et al., 2015).
Ginseng is a perennial herb, requiring four years from sowing to seed production. Thus, using early diagnosis testing is critical when seeking to reduce the time required to develop disease-resistant cultivars. However, limited research has been reported thus far on the design of early diagnosis testing customized for the development of ginseng cultivars with leaf blight resistance.
When affected by disease and pest attacks, crops are known to increase the release of phenolic substances or 2, 4-dihydroxy-7-methoxy-1, 4-benzoxazin-3-one (DIMBOA) as secondary metabolites or to enhance their leaf cuticles to prevent the infiltration of pathogens (Mukanganyama et al., 2003; So et al., 2010b; Kim et al., 2012). While, previous studies have reported that leaf cuticles do contribute to preventing high-temperature damage, there have been no studies of their effects on preventing pathogen infiltration (Lee et al., 2011).
Research on ginseng has primarily focused on optimal light intensity levels (Lee et al., 2022a), high-temperature damage (Lee et al., 2010; Lee et al., 2021), and the development of new cultivars with enhanced resistance to physiological disorders, including lodging (Lee et al., 2021; Seo et al., 2023) and rusty roots (Seo et al., 2023). Kowon is the first cultivar developed to be resistant to leaf blight in ginseng (Kim et al., 2017). The occurrence of leaf blight is closely related to rainfall and temperature factors. However, few studies have explored the correlation between weather conditions and leaf blight resistance, especially in the cultivation of Kowon. Given this lack of associated research, there is no guarantee that Kowon will not lose its resistance to leaf blight in the face of critical changes in environmental conditions.
This study used two ginseng cultivars, Chungjin, a cultivar resistant to leaf blight, and Sunone, a cultivar susceptible to the disease, and focused on the following topics: (1) the establishment of early diagnosis testing for leaf blight, (2) a histological examination of leaf cuticles to identify the origin of resistance, (3) the determination of any correlation between microclimate conditions and disease progression after artificial inoculation, and the natural development of leaf blight in shaded fields, and (4) an investigation of the effect of fewer pesticide applications on the occurrence rate of leaf blight and the yield of ginseng produced in Chungjin.
Materials and Methods
Test strains
The inoculum (KGC-AP040) was injected into an agar medium of V-8 juice and cultivated at a temperature of 20°C for seven days under alternating light and dark conditions (12 h/12 h). As a result, spores were formed, and these spores were used to produce a spore suspension (1 × 105 spores/mL) for the subsequent injections.
Experiment 1. Establishment of early diagnosis testing for leaf blight resistance
The leaves of four-year-old Chungjin and Sunone were washed three times with distilled water for five minutes. Filter paper, soaked with 100 mL of distilled water, was placed on a tray. On top of the paper, a petri dish was positioned upside down to prevent the test leaves from softening due to moisture. On top of the dish, each ginseng leaf was placed. In an attempt to identify the optimal locations for determining the resistance to the disease, 0.2 mL of a spore suspension (1 × 105 spores/ mL) was inoculated on ten spots on both the adaxial and abaxial surface of the leaf, excluding the veins (Artificial Inoculation Test) (Lee et al., 2022b).
After the inoculation of the pathogen, the test leaves were placed in a thermos-hygrostat (DS 50CH, Dasol Sci., Kyungki, Korea) with a humidity level of 70% and radiation level of 23.0 μmol·m-2·s-2 (Li et al., 2020), under varying temperature conditions of 15, 20, 25, and 30°C to find the optimal temperature for disease development. For each temperature, three leaves were tested with a completely randomized design; i.e., each treatment was repeated three times. Eight days after inoculation, the diameters of the gangrene lesions were measured, and the corresponding index was recorded (Deng et al., 2012).
Experiment 2. Histological characteristics of leaves
Four-year-old leaves were collected, washed with water, and then cut into 7 mm × 7 mm pieces. Each cut piece was fixed and immersed in formalin-acetic acid-ethyl alcohol for 12 h. Each fixed leaf was subjected to dehydration in a series of increasing ethanol concentrations (50%, 70%, 80%, 90%, 95%, and 100%) for 50 min at each concentration. The hydrated leaves were then embedded in paraffin.
Afterward, each specimen was sliced using a rotary microtome (Leica 820, Leica Biosystems, Leitz Park, Wetzlar, Germany) into a series of continuous thin sections, each with a thickness of 8 μm. The thin sections were dual-dyed with safranin and fast green FCF and then covered with curved glass to observe the tissue cells via optical microscopy (Axiphot 2, Carl Zeiss, Oberkochen, Germany).
Meanwhile, in an attempt to observe their cuticle layers, each leaf was cut into 2 mm х 2 mm pieces under a dissecting microscope (M205 A, Leica Biosystems, Leitz Park, Wetzlar, Germany). The cut pieces were then fixed and immersed in a 4% glutaraldehyde solution (25 mM phosphate buffer) at 25°C for two hours. Subsequently, they were washed three times with a buffer solution, for 20 min each. Afterward, the washed pieces were fixed and immersed in a 2% osmic acid solution at 2°C for one hour before being washed three times with distilled water, each for 20 min. Dehydration was conducted in a series of increasing ethanol concentrations (50%, 70%, 80%, 90%, 95%, and 100%) for one hour at each concentration.
The dehydrated specimens were stored in isoamyl acetate and then dried in a critical point dryer (BioRadical E3000 dryer, Nakahara, Tokyo, Japan) at a critical temperature under 1,000 psi. Subsequently, the dried specimens were mounted on a stub and then underwent gold coating for 10 min using an ion coater (JFC 1110E, JEOL Ltd., Tokyo, Japan) before being observed under a scanning electron microscope (Hitachi S-3500N, Instruments, Hitachi, Ltd., Tokyo, Japan) (SEM) at 20 kV.
The number of pores per unit area of the leaf was counted as follows. Five spots were randomly chosen in each specimen, and the number of pores in each spot at a magnification of 150× was measured using an optical microscope (Axiphot 2, Carl Zeiss, Oberkochen, Germany). The size of pores was estimated by measuring the major-axis length of 50 randomly chosen pores observed in the SEM images.
Experiment 3. Disease progression after artificial inoculation in the shaded field
Six-year-old ginseng plants grown in Anseong were subjected to artificial inoculation, and shades with a rear-line structure were applied. To minimize the infiltration of rainfall, rain shelter shading plates were used as a covering. Additionally, from June to August, black double-weave fabric with a light transmittance of 30% was added to the shade in each case to prevent high-temperature damage.
From sprouting until May 30, a combination of Polyoxin B and Mancozeb as well as a combination of Polyoxin D and Mancozeb were alternately applied as pesticides for seven days each to prevent stem blight. Subsequently, from June 1 to July 13, no pesticides were applied intentionally to induce the development of leaf blight.
Artificial inoculation was conducted according to the established procedure outlined in the Artificial Inoculation Test. Specifically, inoculations were made into the central leaflet of five ginseng plants sampled on June 7. In an effort to minimize the effects of rainfall and direct sunlight on disease progression, robust plants located in the third or fourth rows were selected for testing. In an effort to facilitate disease development after inoculation, each leaf specimen was sealed in a zipper bag with a paper towel soaked with 100 mL of distilled water, ensuring an internal humidity of 70% or more. The specimens were maintained under these conditions for seven days.
Once the disease developed, tracing paper with dimensions of 210 mm in width and 297 mm in length was used to cover the affected central leaflet. The outline of the entire central leaflet, along with the affected area and its progression over time, was then documented every three days. After completing the outline drawing, the entire leaf area was measured with a leaf area meter (Li-COR Bioscience, Li-3050C, Lincoln, NE, USA) using sheets of tracing paper (STP). Every three days, changes in the affected area resulting from disease progression were quantified on a percentage scale by cutting out the corresponding portion of the tracing paper.
The results were then converted into visual inspection indexes as follows: 0 for no disease, 1 for an affected area of 1% or less, 3 for an affected area over 1% but 5% or less, 5 for an affected area over 5% but 10% or less, 7 for an affected area over 10% but 20% or less, and 9 for an affected area of 20% or more (RDA, 2012).
Experiment 4. Correlation between disease progression and microclimate conditions after disease development in the shaded field
The same ginseng field, cultivation method, crop protection agent application method, and ginseng plants of the same age group as described in Experiment 3 were used. Natural disease development rates were measured three times: the first investigation was conducted from June 14 to June 23, the second from June 24 to July 3, and the third from July 4 to July 13. During each measurement period, newly affected plants were identified and analyzed.
Leaves with gangrene lesions with a diameter of 5 mm or less were selected for analysis (Deng et al., 2012). For each measurement period, five leaves were analyzed. Changes in the affected area due to disease progression were quantified daily according to the method above described in STP.
To determine the correlation between natural disease development and weather conditions, in this case the temperature, humidity, and rainfall, an S-THB-M002 device (HOBO Co., Bourne, MA, USA), a temperature and humidity sensor, was installed 60 cm above the ridge between fields. This setup made it possible to measure the temperature and humidity within the shade. Rainfall levels were measured using the HOBO S-RGB-M002 sensor (HOBO Co., Bourne, MA, USA), which was installed 2 m above the ground outside the shade. A U30 device (HOBO Co., Bourne, MA, USA), which is a data logger, was employed to collect temperature, humidity, and rainfall data from June 1 to July 13, with data collection conducted every hour throughout the day. Daily data were estimated and recorded by averaging the hourly measurements for each day.
Experiment 5. Yield reduction resulting from reduced pesticide application
The reduction in yield was measured in six-year-old ginseng plants. In order to prevent the occurrence of stem blight, pesticide applications were performed on the experimental group six times within the period from sprouting to May 30, following the methods described in Experiment 3. To prevent the development of leaf blight, only two pesticide applications were used within the period from July 1 to August 31. The first pesticide application was done on July 10, using a combinations of Polyoxin B and Mancozeb. The second application was done August 10, with a combination of Polyoxin D and Mancozeb.
The test plants were arranged in a field with an area of 3.24 m2 using a three replications randomized block design. They were harvested on September 30. Among the harvested plants, those with either half of the root rotten or a root weight of 30 g or less were excluded from the plant count. Then, the root weight and yield were investigated (RDA, 2012).
Statistical analysis
Statistical analysis of all experimental data was conducted using the SAS (SAS v9.2, SAS Institute Inc., Cary, NC, USA) analysis software package. Specifically, the Pearson product-moment correlation coefficient was employed to analyze the correlation between disease progression by natural infection and microclimate conditions, in this case the temperature, humidity, and rainfall levels. Student's t-test was used to analyze both the number and characteristics of pores and to optimize early diagnosis testing.
Results
Development of early diagnosis testing for determining resistance to leaf blight
At 15°C, leaf blight did develop, but its symptoms were difficult to identify with the unaided eye. At both 25°C and 30°C, however, leaf blight did not develop at all. Consequently, it was deduced that 20°C was the most suitable temperature for optimizing early diagnosis testing for leaf blight (Fig. 1). Meanwhile, when the pathogen was inoculated on the abaxial sides of the leaves, disease development occurred so rapidly that determining resistance and susceptibility degrees was difficult (Table 1).
In contrast, the disease progressed at a moderate rate on the adaxial sides of the leaves, which was a suitable condition for determining the degree of resistance. The optimal conditions for early diagnosis testing for resistance were determined as follows: inoculating the pathogen into the adaxial sides of the leaves and maintaining the plant at 20°C for eight days.
Difference in the histological characteristics of leaves between resistant and susceptible cultivar
The epidermis cells of leaves in Chungjin and Sunone consisted of a single adaxial layer, a single abaxial layer, a layer of palisade tissues, and three layers of spongy tissues. The density of the tissues, including palisade tissues and spongy tissues, was lower in Chungjin than in Sunone (Fig. 2A and 2B).
Cuticle layers were observed on both the adaxial and abaxial sides of the leaves. On the adaxial sides, Chungjin exhibited greater cuticle development than that in the Sunone case (Fig. 2C and 2D). This well-developed cuticle structure of Chungjin, a resistant cultivar, is considered to prevent the infection of the pathogen and slow its progression once infected (Lee, 2008).
There were no differences in the number and major-axis of pores on the abaxial sides of the leaves between the Chungjin and Sunone cases, indicating that the effects of these factors on disease development were insignificant.
Correlation between leaf blight development and weather conditions in the shaded field: Comparison between natural development and artificial development after inoculation
In the shaded field, after inoculation the disease developed on June 14, but progression did not occur until June 20. On June 17, it started to rain, and the humanity increased, triggering spore germination. When the temperature in the shade reached 20°C, the disease development rate in Sunone rose to 3.5, ultimately reaching 5.3 by the last day of the investigation. This rate was higher than that of Chungjin, which was 3.8 by the last day of the investigation (Fig. 3).
Natural disease development started on June 14. The first investigation found that in both Chungjin and Sunone, the effects of the three weather parameters (temperature, rainfall, and humidity) on disease progression were insignificant. In the second investigation as well, Chungjin proved to be resistant to leaf blight, as its progression was unaffected by the temperature, rainfall, and humidity. In Sunone, however, a high correlation was found between disease progression and rainfall (p < 0.01). The third investigation confirmed the significant effect of temperature (p < 0.05) on disease progression in Sunone (Fig. 4 and Table 3).
Overall, a district difference in disease development was observed between Sunone and Chungjin, with the development of resistance and susceptibility significantly influenced by weather conditions.
This result aligns with previous studies that identified a correlation between disease development and water leakage from rain shelter shading plates. This correlation was established based on the observation that disease development rates were higher when polyethylene black four-weave fabric (PE4), which was more susceptible to water leakage, was used compared to rain shelter shading plates.
Yield discrepancies resulting from reduced pesticide applications
Despite only two pesticide applications between June and August, Chungjin, a resistant cultivar, exhibited a 38.2% increase in the root weight, and its yield was 2.8 kg, a 22% increase compared to Sunone (Table 4 and Fig. 5). This observation is consistent with previous studies that reported a 10 to 30% reduction in the yield for Korean ginseng and American ginseng affected by leaf blight for a year (Hill and Hausbeck, 2008; Cho, 2009; Kim et al., 2010).
Discussion
Development of early diagnosis testing for leaf blight resistance
When affected by disease, pest attacks, or mechanically damaged by external forces, plants undergo internal physiological changes, resulting in an increased release of secondary metabolites, such as DIABOA and phenolic compounds. When plants in such conditions are subjected to early diagnosis testing, diagnostic errors may occur during the resistance assessment (So et al., 2010b).
Meanwhile, when assessing resistance to disease in shaded fields or greenhouses, conidial suspensions are widely used. While this method proves cost-effective for diagnosing plants' resistance to disease, there is a possibility of diagnostic errors in cases where conidia are non-uniformly applied to the plants or where the distribution of humidity and temperature varies in the surroundings, affecting disease development in different ways (Challagulla et al., 2015). One of the most important traits of early diagnosis testing for determining resistance is the ability to conduct quick diagnoses before the target plants undergo any internal physiological changes.
There have been various reports on the optimal temperature conditions for leaf blight development, such as temperature ranges of 18–25°C or 25–27°C (Hill and Hausbeck, 2008). However, these measurements were obtained from shaded fields and are thus considered to have been significantly affected by weather conditions. Consequently, these results cannot be regarded as sufficiently accurate.
Meanwhile, Deng et al. (2012) conducted indoor experiments and concluded that disease development occurred at 15°C. However, a 20°C condition was not included in their experimental setup. Thus, a direct comparison with the present study is not appropriate; the optimum temperature conditions determined in this study differed from their results. In this study, disease development was not distinctive at 15°C, making it difficult to determine the presence of resistance. At both 25°C and 30°C, disease development did not occur. Consequently, 20°C was confirmed as the most suitable temperature for optimizing early diagnosis testing for leaf blight development (Fig. 1).
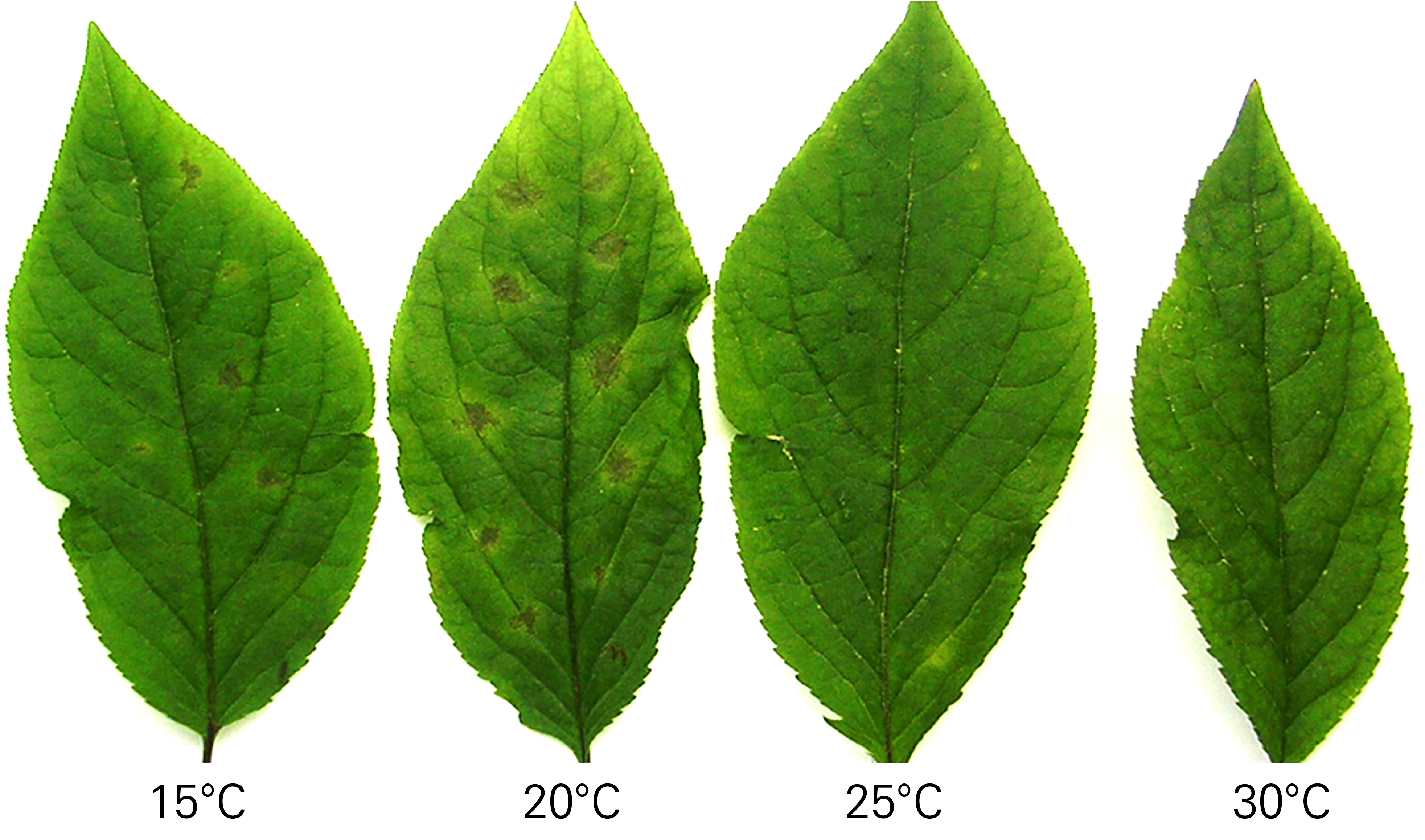
Fig. 1.
Examination of temperature-induced differences in disease symptoms, with the goal of establishing an optimal early diagnosis testing method for diagnosing leaf blight in ginseng. A spore suspension with a concentration of 1 × 105 spores/mL was drop-inoculated onto ten spots on the adaxial sides of the leaves. The leaves were cultivated at temperatures of 15, 20, 25, and 30°C, depending on the group, for eight days before examining disease symptoms.
Additionally, according to previous studies on grape phomopsis blight, the diagnosis of resistance to the disease can vary depending on the location of the leaf into which the pathogen is inoculated (Park et al., 2006). In ginseng, pores are present only on the abaxial sides of leaves. These pores serve as channels through which the germ tubes of conidia can easily infiltrate. Moreover, leaf cuticles are thinner on the abaxial than on the adaxial sides of the leaves, facilitating disease infiltration (Lee et al., 2011).
For these reasons, disease progression on the backside of leaves was excessively fast eight days after inoculation. Thus, this condition proved unsuitable for determining the presence of resistance. Conversely, eight days after inoculation onto the adaxial sides of the leaves, a significant difference in disease development was observed between the two cultivars, indicating the suitability of adaxial side inoculation for the resistance diagnosis (Table 1).
Table 1.
Preference index for leaf blight after artificial inoculation on both sides of ginseng leaves
Cultivars | Inoculation surface of the leaf | t-testy | |
Adaxial | Abaxial | ||
Chungjin | 3.2 ± 0.6z | 5.3 ± 0.6 | ‒6.516** |
Sunone | 5.7 ± 0.6 | 6.3 ± 0.6 | ‒1.632ns |
The optimal conditions for optimizing early diagnosis testing for leaf blight in ginseng were determined as follows: a spore suspension with a concentration of 1 × 105 spores/mL is inoculated on the adaxial sides of the leaves at a temperature of 20°C and a humidity rate of 70%, and actual testing is conducted eight days after inoculation.
Relationship between histological characteristics of the leaves and resistance
In general, cuticles function as the first barrier against pathogens seeking to infiltrate plant leaves (Chassot et al., 2007). Previous studies reported that the thicker cuticle layers in Arabidopsis contributed to delaying the development of gray mold rot (Bessire et al., 2007). Other studies showed that in cultivars with well-developed leaf cuticles, the infiltration of pathogens led to hyphal growth, while the germination of spores was inhibited. The study attributed this inhibition to the effect of cutinase (Chassot et al., 2007).
The observed inhibition of pathogen infiltration and disease progression in Chungjin, a resistant cultivar, can be attributed to its well-developed cuticles compared to Sunone, a susceptible cultivar, despite having fewer leaf cells and less dense tissue cells.
Ginseng plants with thicker leaf cuticles tend to be more resistant to high-temperature damage because cuticles serve to prevent moisture loss (Fig. 2C and 2D). Furthermore, cuticles reduce the time required for dew formation. To sum up, well-developed cuticles reduce the time during which moisture is maintained on the leaf surface, leading to difficulty in achieving optimal conditions for spore germination in leaf blight (Lee, 2008; Lee et al., 2011).
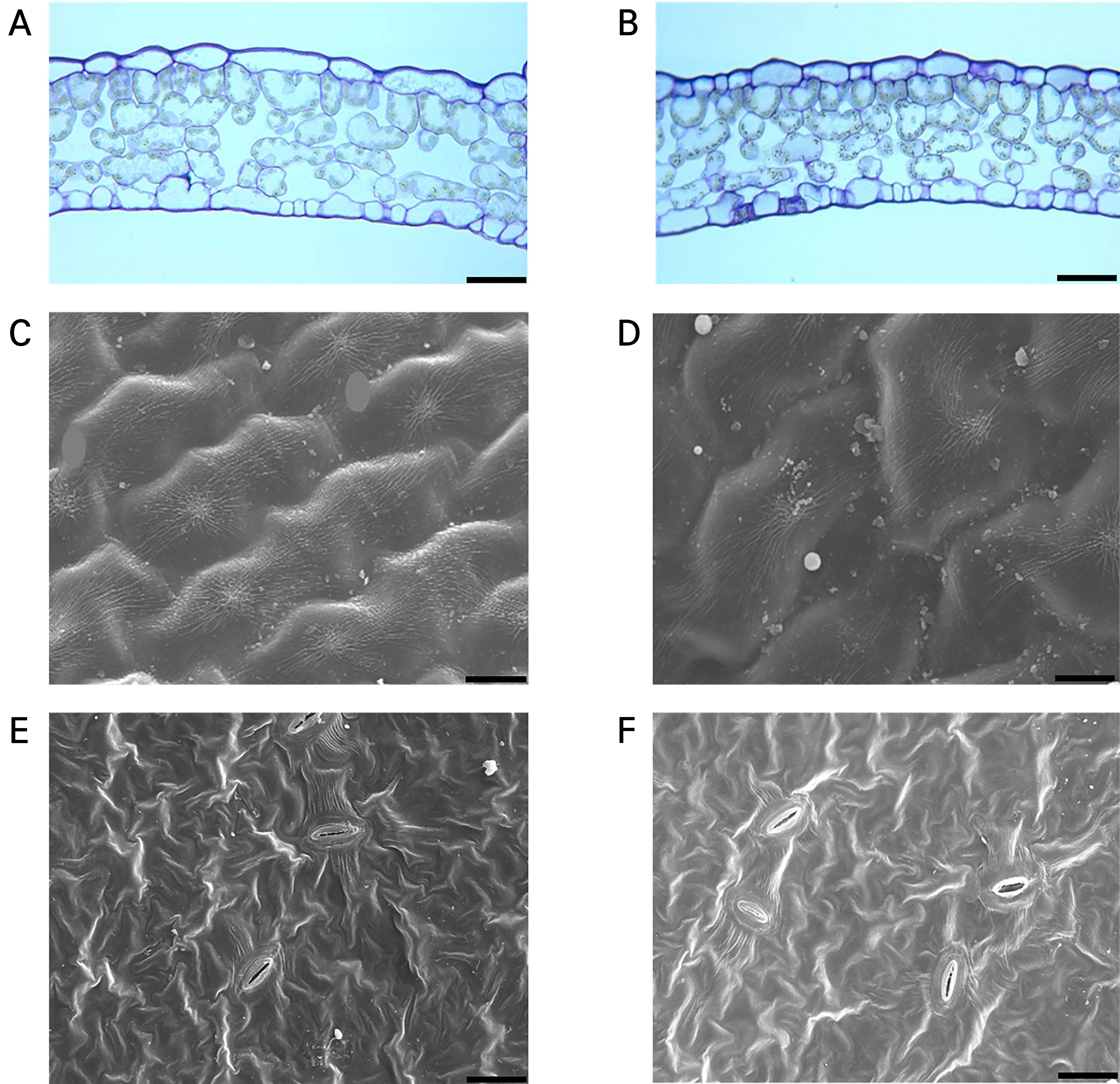
Fig. 2.
Comparison of the epidermal structure, cuticle development, and pore characteristics of the leaves between Chungjin and Sunone. A and B represent the epidermal structure of the leaves, C and D represent the corresponding cuticle development on the adaxial sides of leaves, and E and F represent the corresponding pore characteristics at the abaxial sides of the leaves. On the left is Chungjin, a resistant cultivar, and on the right is Sunone, a susceptible cultivar to leaf blight. Black bar indicate 100 μm.
However, there was also a study that reported that despite having thick cuticle layers, Sunil was prone to leaf blight (Lee et al., 2021); that study attributed this observation to the fact that while cuticles did serve to inhibit the infiltration of pathogens, the actual resistance to the disease would be derived from secondary metabolites rather than from the cuticles themselves (So et al., 2010b; Lee et al., 2021).
This study confirmed that well-developed cuticles with enhanced density delayed both disease development and progression. Future studies should focus on exploring the effects of increasing or decreasing secondary metabolites and cuticle development on the plants' resistance to disease.
There were no significant differences in the numbers and lengths of the pores between Chungjin and Sunone (Table 2). This result, however, contradicts a previous study that reported that the infiltration of mycelia of grape phomopsis blight pathogens was more difficult when the pores were smaller and there were fewer of them (Park et al., 2006). Compared to other crops, ginseng, as a heliophobic cultivar, tends to minimize photosynthesis; thus, its pores are present only on the abaxial sides of the leaves, with relatively few and relatively small pores, in contrast to heliophilous crops (Lee et al., 2011; Lee et al., 2015b).
Table 2.
Comparison of the pore number and major-axis length between Chungjin and sunone
Cultivars | Number of pore (No./0.53 mm2) | Major-axis of pore (μm) |
Chungjin | 19.7 ± 0.3y | 30.8 ± 0.5 |
Sunone | 20.8 ± 0.8 | 27.8 ± 0.1 |
t-testz | ‒0.942ns | 1.264ns |
During the cultivation of ginseng, the decreased light transmission through the shade results in an increase in the number of chloroplasts and pores. Conversely, an increase in light transmission leads to a decrease in the number of chloroplasts and pores. As such, these factors interact complementarily. An increase in light transmission reduces leaf blight development because it leads to a decrease in the relative humidity and an increase in the temperature within the shade, two crucial factors influencing leaf blight development (Cheon et al., 2003; Lee et al., 2022a).
Consequently, this study confirmed that there was no significant correlation between the number and length of pores and leaf blight resistance. Going forward, the development of leaf cuticles and their thickness can be used as indexes to identify resistance in the development of new cultivars resistant to leaf blight.
Correlation between leaf blight progression and microclimate conditions in a shaded field: Comparison between natural development and artificial development after inoculation
After artificial inoculation in a shaded field, the highest correlation of disease development was found with rainfall, followed by the temperature within the shade. Humidity, which exhibited a negative correlation, was not associated with disease progression at all (Figs. 3 and 4). These findings are consistent with previous results indicating that optimal conditions for disease development were achieved when the relative humidity within the shade increased due to rainfall while the temperature was 20°C (Hill and Hausbeck, 2009; Cho, 2009; Kim et al., 2010).
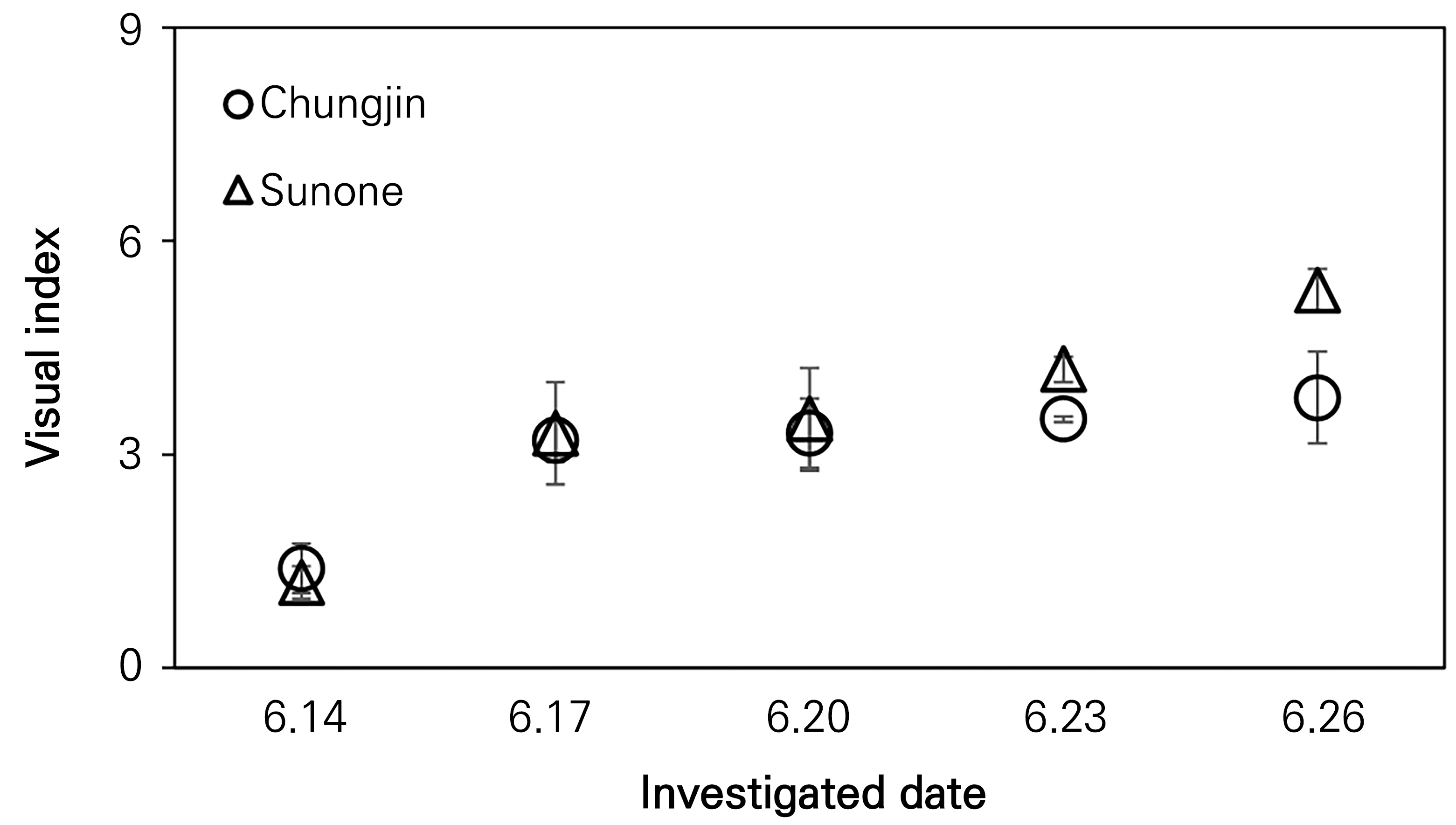
Fig. 3.
Development and progression rates of leaf blight after artificial inoculation on the leaves of ginseng plants grown in shaded fields. Disease progression rates monitored every three days after inoculation with a spore suspension at a concentration of 1 × 105 spores/mL on the adaxial sides of the leaves. The visual indices Chungjin and Sunone present the measured area of five leaves affected by the leaf blight. Bars are the standard deviations.
In the shaded field, natural disease development occurred on June 4, but disease progression was slow in both cultivars due to unfavorable weather conditions. However, in plants where the disease developed on June 14 and 24 and July 4, rapid disease progression occurred due to optimal conditions, including sufficient moisture within the shade from rain and sufficiently low temperatures (Fig. 4).
The first investigation found that there was no significant correlation between disease progression and the weather conditions tested here, i.e., the temperature, rainfall, and humidity, in both Chungjin, a resistant cultivar, and Sunone, a susceptible cultivar. In the second investigation, a correlation between disease development and weather conditions was identified: increased rainfall resulted in higher disease development rates in Sunone (p < 0.05), a susceptible cultivar. However, no such correlation was found in Chungjin, demonstrating its resistance to the disease. During the third investigation, it rained every day, leading to excess moisture within the shade.
Thus, no correlation was identified between disease progression and rainfall. In contrast, a correlation between disease progression and temperature was found. This result can be interpreted as the effect of temperature on disease development becoming more pronounced because moisture remained at a suitable level. Throughout the first to third investigations, humidity had an insignificant effect on disease progression, implying that excess moisture does not affect the development of the disease (Table 3).
Table 3.
Analysis of the correlation between leaf blight development and microclimate conditions in the shade for each cultivar
Cultivar | Temperature | Rainfall | Humidity | ||||||||
Firstz | Secondy | Thirdx | First | Second | Third | First | Second | Third | |||
Chungjin | ‒0.262 | 0.324 | 0.664 | 0.576 | 0.517 | ‒0.326 | 0.277 | 0.303 | ‒0.716* | ||
Sunone | ‒0.179 | 0.148 | 0.752*w | 0.649 | 0.818**v | ‒0.271 | 0.307 | 0.199 | ‒0.837** |
This finding aligns with previous studies that reported that higher humidity led to an increase in conidia, while lower humidity resulted in an increase in airborne conidial concentrations (Hill and Hausbeck, 2008). Among the weather conditions examined here, rainfall stood out as one of the most significant factors affecting the development and progression of leaf blight in Sunone during the first and second investigations. In the third investigation, the most significant factor was the temperature (Fig. 4 and Table 3).
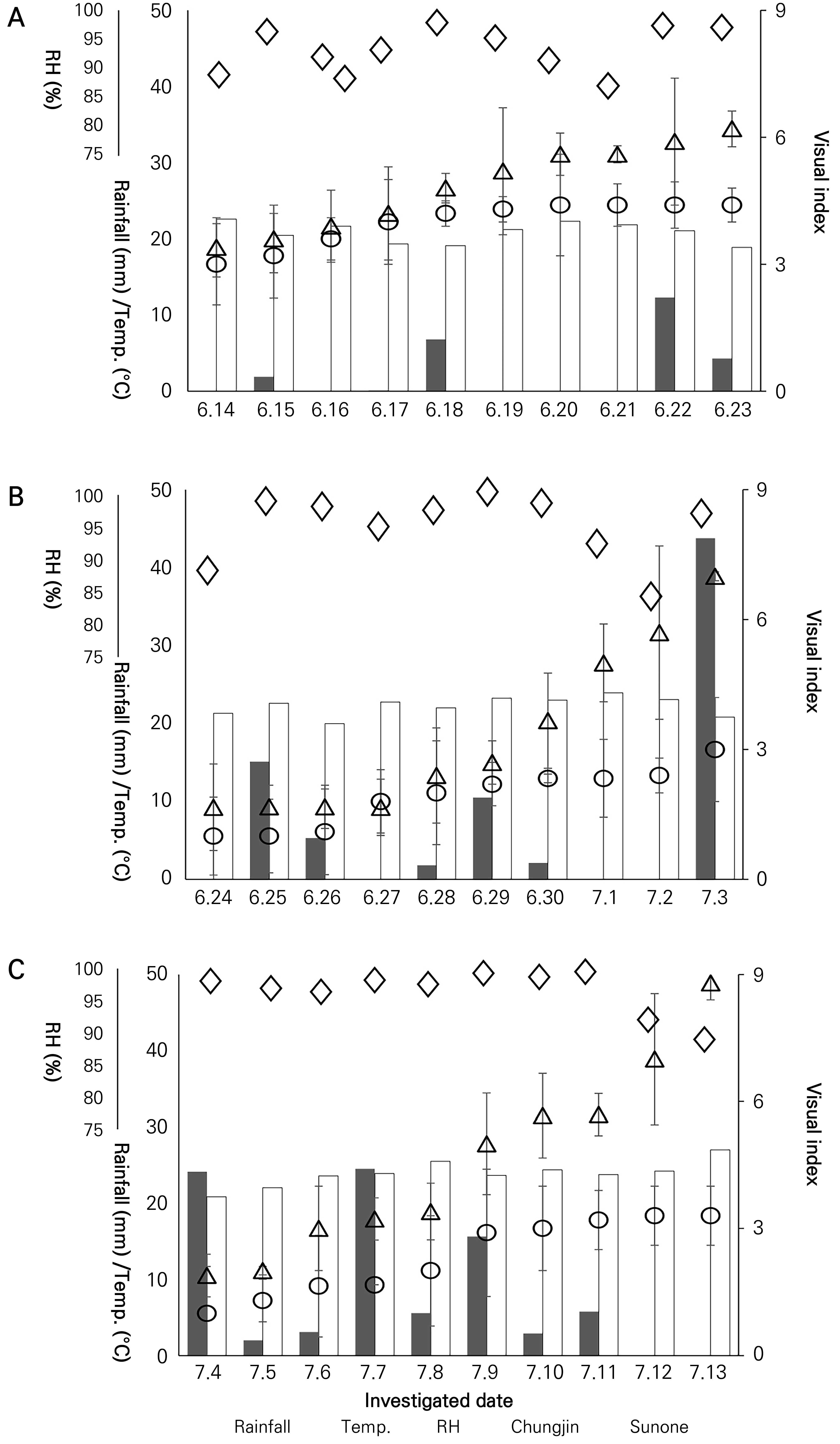
Fig. 4.
Development and progression rates of leaf blight after natural development in a shaded field in relation to the microclimate conditions within the shade. A, B, and C represent the first, second, and third investigations, respectively. Rainfall is denoted by the long black bars, temperature (Temp.) are indicated by the long white bar, and the diamonds represent the relative humidity (RH). The visual indices of Chungjin and Sunone present the measured area of five leaves affected by the leaf blight. Bars are the standard deviations.
These outcomes stem from the fact that moisture plays the most critical role in spore germination until it rains. Once sufficient moisture is present, the next necessary condition for spore germination is the optimal temperature (Hill and Hausbeck, 2008).
Kim et al. (2010) reported increased susceptibility to leaf blight when using PE4. Cheon et al. (2003) found that increased light transmission through the shade resulted in lower disease development rates. These previous findings align with the correlation found in this study between disease progression and weather conditions. Indeed, one report indicated that when the rainfall level was 13 mm or less due to a severe drought from April to June in 2005, the development of leaf blight was delayed by about two weeks compared to 2004. The level of rainfall was three times higher in 2004 compared to 2005 (Cho, 2009). This observation confirmed the most significant influence on the development of the disease.
EC50 is an effective method for predicting the efficacy of pesticides when applied for disease prevention in shaded fields. It is also useful for anticipating the emergence of pesticide-resistant strains, especially with repeated applications of the same types of pesticides. Kim et al. (2009) estimated the EC50 values for pathogens collected from 23 areas against Car and Car combined with Diethofencarb, both being gray mold rot pesticides. They reported the emergence of pesticide-resistant bacteria at a pesticide concentration of 10 μg/mL or more with respect to Car/mixture-resistant, car-resistant, and mixture-sensitive pathogens. Lu et al. (2016) observed that gray mold rot exhibited exceptional adaptation in nature, often resulting in the emergence of resistant bacteria. Their experimental results reported that the proportion of bacteria resistant to one, two and three pesticides was 27.6%, 49.9%, and 21.1%, respectively.
In the US, the emergence of bacteria resistant to iprodione, a leaf blight pesticide, was reported in 1987 (Rahimian, 1987). In Korea, there has been no report of the emergence of such bacteria thus far. However, there is always the risk of the emergence of resistant bacteria due to the repeated use of the same pesticide, coupled with the promotion and spread of planting a single cultivar to prevent high-temperature damage and increase production yields (Lee et al., 2015a; Seo et al., 2023).
To prevent this scenario from occurring in the future, it is necessary to avoid the repeated use of the same types of pesticides while promoting the application of eco-friendly agronomic control methods. Among these methods is an approach that increases light transmission within the shade, preventing disease development. Rain shelter shading plates effectively prevent rainwater infiltration but at the same time enhance light transmission. The use of rain shelter shading plates accelerates high-temperature damage rather than reducing it, negatively affecting the growth of ginseng. Moreover, high temperatures and intense light facilitate the decomposition of effective components in pesticides, leading to reduced efficacy or even pesticide-induced harm (Cho, 2009; Lee et al., 2012; Lee et al., 2021; Seo et al., 2023).
Accordingly, the most effective and safe strategy for reducing pesticide usage is to develop eco-friendly, resistant cultivars in which multiple genes are involved in the development of disease resistance, thereby preventing the emergence of resistant strains. Here, being eco-friendly and resistant means that instead of relying on a single gene, multiple genes intervene in preventing leaf blight. Moreover, rather than completely preventing the disease, these genes enable it to develop to a certain extent, creating a type of symbiotic relationship (So et al., 2010b; Kim et al., 2012).
Chungjin, developed in this study, falls within this category. In this cultivar, the development of leaf blight is not completely prevented; instead, the disease progresses to a certain extent, thus reducing the emergence of resistant strains (Fig. 5).
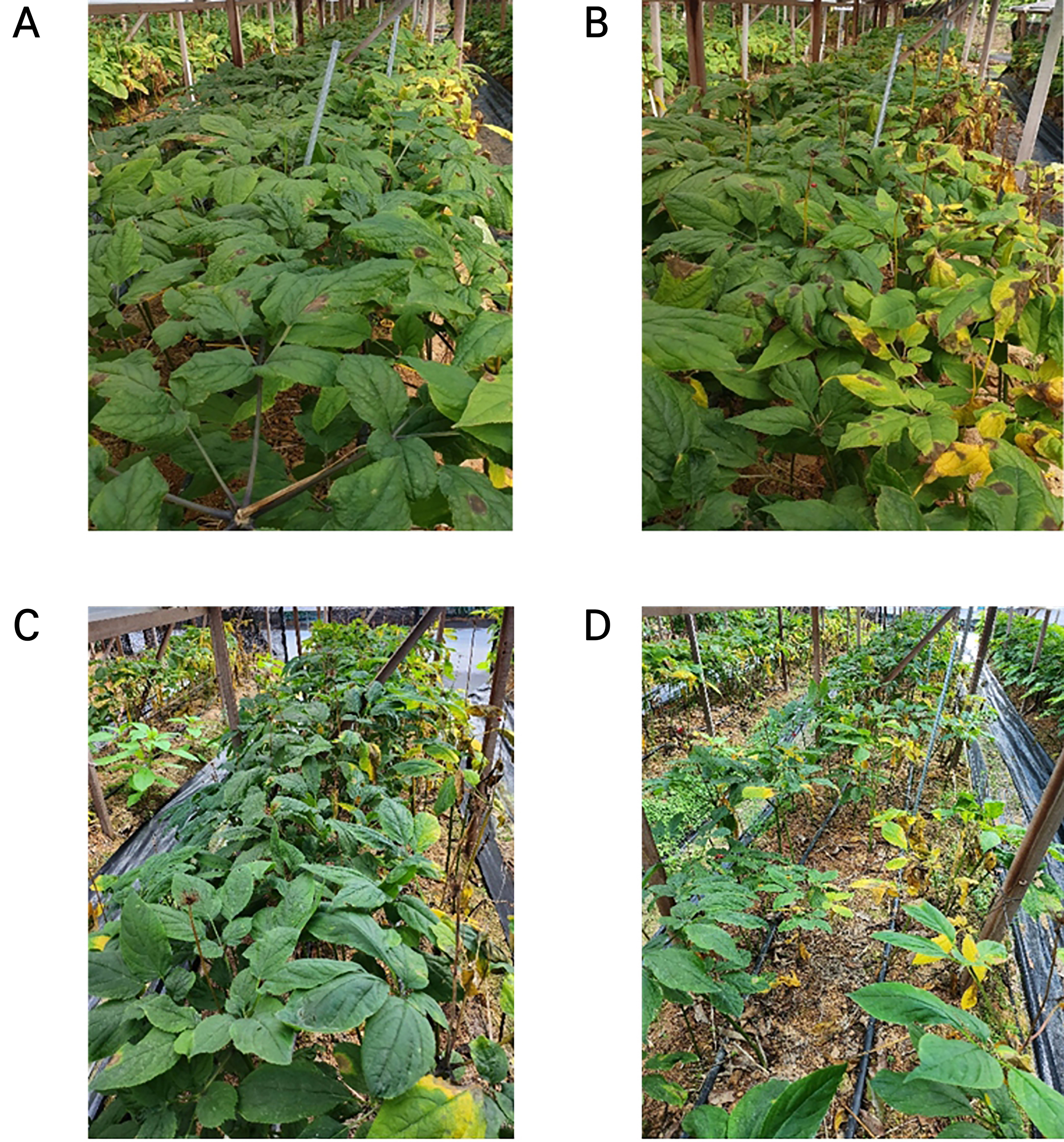
Fig. 5.
Comparison of leaf blight development patterns between cultivars with respect to a reduced number of pesticide applications to prevent leaf blight. A and B show the symptoms observed on July 31 during the initial investigation following leaf blight development, while C and D show the symptoms observed on August 31 during the final investigation. On the left is Chungjin, a resistant cultivar, on the right is Sunone, a susceptible cultivar. The first pesticide spraying was done on July 10, with a pesticide combination of Polyoxin B and Mancozeb. The second was on August 10, with a pesticide combination of Polyoxin D and Mancozeb.
Yield reductions due to decreased pesticide applications
Although the number of pesticide applications for preventing leaf blight was only two, the yield reduction was 22% less in Chungjin than in Sunone (Table 4). These results were consistent with a previous report indicating that when affected by leaf blight, the yield reduction were 30% for Korean ginseng (Cho, 2009) and 10–20% for American ginseng (Hill and Hausbeck, 2008).
Table 4.
Comparison of root weights and root yields between each cultivar in relation to reduced pesticide applications for leaf blight prevention
Cultivars | Root weight (g) | Root yield (kg/1.62 m2 -1) | Index (B/A) |
Chungjin (B) | 85.3 ± 37.6y | 2.8 ± 0.2 | 122 |
Sunone (A) | 71.8 ± 21.4 | 2.3 ± 0.1 | 100 |
t-testz | 4.050** | 0.081ns |
Hill and Hausbeck (2008) investigated the optimal interval between pesticide applications. In one group, they applied pesticides a total of 15 times throughout the year, with an interval of seven days between each application. In the other group, ten pesticide applications in total were conducted with a ten-day interval. They found that disease development rates were four to eleven times higher when the application interval was ten days compared to seven days, concluding that the optimal pesticide application interval was seven days.
In this study, the reduction of the yield was estimated on a yearly basis, but if the estimation is extended to multiple years of damage, the reduction in Sunone, would be even greater. Going forward, it is necessary to examine not only the patterns of yield reduction due to cumulative damage but also the reduction caused by stem blight.
Kowon was the first cultivar reported to be resistant to leaf blight (Kim et al., 2017). However, associated studies examined the development rate of leaf blight after applying pesticides according to the standard ginseng cultivation method, with no consideration of environmental factors related to its development.
These studies were conducted in an environment in which the density of pathogens was limited due to disease control efforts that were taken, suggesting that the resistance of ginseng cultivars can vary if optimal environmental conditions for disease development are met. Therefore, it will also be necessary to assess the resistance of Kowon with fewer pesticide applications.
Conclusion
In summary, this study developed an early diagnosis testing method for leaf blight resistance, involving the inoculation of 0.2 mL of a spore suspension with a concentration of 1 × 105 spores/mL onto the adaxial surfaces of the leaves. The resistance of tested plants can be determined eight days after the inoculation at a temperature of 20°C. By using this method, this study attributed the resistance of Chungjin to leaf blight to its well-developed cuticles, among various histological characteristics of leaves, effectively preventing pathogen infiltration.
This result aligns with reports indicating greater development and progression rates of leaf blight when there was sufficient moisture within the shade, primarily due to rainfall, and when the temperature was 20°C, with both natural development and artificial development after inoculation in shaded fields (Hill and Hausbeck, 2008). Simply put, among different environmental factors, rainfall and temperature both play critical role in disease progression.
Therefore, the best strategy for reducing pesticide application and stabilizing the ecosystem would be to use agronomic control in ginseng cultivation, in which excess moisture is minimized through enhanced ventilation within the shade. Using rain shelter shading plates can also help prevent excess moisture and, consequently, leaf blight. However, certain types of rain shelter shading plates may increase light transmission instead of reducing it, intensifying high-temperature damage (Kim et al., 2012). Thus, such an approach is considered inappropriate.
Therefore, it is important to develop resistant cultivars that can establish a symbiotic relationship with leaf blight pathogens, thereby preventing the emergence of resistant bacteria. Despite the fact that the optimal weather conditions for leaf blight development were met, Chungjin had 22% more yield than Sunone due to the slower progression of the disease.
As such, the development of Chungjin, a cultivar resistant to leaf blight, will contribute to preventing the emergence of pesticide-resistant strains while reducing pesticide usage. This, in turn, will help mitigate carbon emissions and address pesticide residue issues, ultimately enhancing the safety of the ecosystem.