Introduction
Materials and Methods
Transplant Production for 90 Days
Environmental Condition in the T-PFAL
Data Analysis
Results and Discussion
Introduction
Production of high-quality transplants has been emphasized to achieve high yield and quality of the final products in vegetable production. For this, many techniques have been proposed, one of which is transplant production using a plant factory with artificial lighting (T-PFAL; Kozai, 2016). The T-PFAL system is used to produce disease-free transplants using minimum resources in an airtight, well-insulated facility. Moreover, it is possible to rapidly produce uniform transplants by manipulating environmental conditions in this system. To apply these advantages to vegetative propagation, Chun et al. (2012) developed a new vegetative propagation method.
The autotrophic transplant production method (ATPM) was developed based on strawberry (Fragaria × ananassa) transplant production with a PFAL using vegetative propagation (Chun, 2016). Conventional propagation methods are inappropriate for T-PFAL for the following reasons: In the tray plant production method, one of the conventional propagation methods, transplants are produced by separating runner plants of a certain size or planted duration from their propagules (RDA, 2018). In a plug plant production method, another conventional propagation method, unrooted runner plantlets produced on runner chains are separated from their propagules, fixed on cell trays, and grown in a greenhouse or a plastic tunnel with saturated humidity for rooting (Durner et al., 2002). Propagules or stock plants of the first method are too big to grow in T-PFAL using multi-layered propagation beds. The second method needs more than two types of propagation beds or PFALs to produce transplants, such as cultivation systems for producing runner tips (wider space between layers), rooting the runner tips (narrower space between layers and high relative humidity), and growing runner plants after rooting (narrower space between layers). In contrast, the propagules in the ATPM are smaller in size and are produced using only one type of PFAL. Park et al. (2018) reported that small-sized propagules can be produced by early fixation of runner tips and propagation cycles were reduced using runner plants separated from stock plants 20 days after fixing runner tips, with an approximately 5-mm crown diameter, as propagules. Moreover, less waste is produced from the ATPM than from conventional methods because the propagules with terminated propagation in the ATPM can be used as transplants for the conventional methods, while those in the conventional methods are thrown out.
Kubota and Kozai (2001) reported that the number of transplants was higher with a shorter propagation cycle in vegetative propagation. The timescale of the propagation cycle required to produce new propagules (TPC) was affected by the size of propagules. In a vegetative propagation method for sweet potato (Ipomoea batatas) using T-PFAL, there are two types of TPC: timescales for cutting and for stock plant bases (Kubota and Kozai, 2001). In the ATPM for strawberry production, there are three types of TPC because a propagule produces three runner plants that are then used in conventional methods as transplants or stock plants due to their similar size (Chun, 2016). The TPC in the ATPM is the sum of time from placing propagules to emergence of runners (TPE) and the time from emergence of runners to separation of a runner plant from the propagule (TES). Because emergence of the runners can be promoted from propagules with surplus resource pools (Alpert, 1991), we hypothesized that, as the size of the propagules decreases, the TES shortens, although the TPE may increase.
Programs to produce certified disease-free strawberry transplants have been developed in major strawberry production countries for supplementation of high-quality propagules. Higher-grade transplants are grown under stricter conditions for preventing the spread of diseases to lower-grade plants. Therefore, the ATPM is an appropriate technique for the production of higher-grade strawberry transplants due to the use of T-PFAL, and determination of the minimum TPC can improve the production rate of transplants in the ATPM.
We hypothesized that, the smallest size of runner plants that can grow independently without support from their stock plants and can be used as propagules will minimize the TPC. To determine the appropriate size of propagules for the ATPM, we investigated propagation cycles, growth of propagules and runner plants, and the accumulated number of transplants produced in a T-PFAL for 90 days as affected by the crown diameter of the propagules.
Materials and Methods
Transplant Production for 90 Days
Strawberry plants (Fragaria × ananassa Duch. ‘Maehyang’) for propagules were selected based on crown diameter (4.0–4.2, 4.9–5.1, and 5.9–6.1 mm) and named CD4, CD5, and CD6, respectively. These respective plants had two leaves and one runner, two leaves and two runners, and three leaves and two runners. We planted them into 150 mL containers using 32-cell cutting plug trays (Bumnong Co. Ltd., Jeongeup, Korea) filled with commercial medium (Plant World; Nongwoo Bio Co. Ltd., Suwon, Korea), placed them in a T-PFAL (closed transplant production system; 3 × 3 × 3 m; Paru Co. Ltd., Yeosu, Korea), and produced runner plants for 90 days.
Runner tips generated from the propagules were fixed in the 32-cell cutting plug tray filled with commercial medium for development of adventitious roots and formation of runner plants when their bracts unfolded (Fig. 1). However, in cases of already unfolded bracts and leaves, they were fixed on medium trays regardless of size. The crown diameter of runner plants derived from the runner tips was measured every day, and the runner plants were separated from their propagules when their crown diameter reached the chosen size. When the runner plants were separated, we measured numbers of leaves and runners, placed them in the propagation bed, and cultivated them as subsequent propagules for the next generation. We also measured the timeline of propagation cycles required to produce new propagules (TPC), the time from placing propagules to the emergence of runners (TPE), and the time from the emergence of runners to the separation of a runner plant from the propagule (TES) to verify the TPC as affected by the crown diameter of propagules (Fig. 2).
The propagules that produced three runner plants were removed from the T-PFAL, and we measured the number of leaves, crown diameter, and dry weight. When the number of propagules in the T-PFAL exceeded the maximum number of propagules that can be cultivated in the propagation area (3.6 ㎡; 160 propagules), the newly formed propagules were removed from the T-PFAL. We counted the number of runner plants produced in the T-PFAL every day until 90 days after placing initial propagules (DAP).
Environmental Condition in the T-PFAL
The propagules and runner plants were grown in the T-PFAL with 32W cool white fluorescent lamps (TLD32W830RS, Philips Electronics, The Netherlands) for each bed, and the photosynthetic photon flux density (PPFD) above the bed was 230 µmol·m-2·s-1. Air temperature during the photo/dark-period was maintained at 27°C/23°C, and the photoperiod and CO2 concentration were 16 h·d-1 and 800 µmol·mol-1, respectively. A closed irrigation system was utilized in the plant factory used in this study, and plants were sub-irrigated with Yamazaki nutrient solution for strawberries (Yamazaki, 1978; pH 6.0 and EC 0.7 dS·m-1) for 10 minutes, once a day.
Data Analysis
We statistically analyzed the experimental data using SAS 9.2 (SAS Institute Inc., Cary, NC, USA) with LSD tests. Treatment differences were considered significant at a level of 5% probability.
Results and Discussion
For 90 days, the accumulated number of transplants produced was greatest from nine propagules in CD5 (445 transplants), followed by those in CD6 (323 transplants) and then CD4 (308 transplants) (Fig. 3). The timing of propagule removal from the T-PFAL due to production of three runner plants in CD4 was earlier (25 DAP) than those in CD5 (29 DAP) and CD6 (30 DAP); however, the timing of runner plants removed due to shortage of propagation area in CD4 was latest (82 DAP), followed by those in CD6 (78 DAP) and then CD5 (69 DAP). Accumulated numbers of released propagules and runner plants from the T-PFAL in CD5 were greater (101 propagules, 184 runner plants) than those in CD4 (68 propagules, 80 runner plants) and CD6 (70 propagules, 93 runner plants).
The first, second, and third TPC in CD5 were 16.5, 27.7, and 37.9 days, respectively, smaller than those in CD4 (20.8, 33.2, and 40.0 days) and CD6 (19.3, 32.0, and 38.7 days; Fig. 4). The first, second, and third TPE in CD4 were 1.4, 12.4, and 19.7 days, respectively, which were greater than those in CD5 (0.0, 2.2, and 10.9 days) and CD6 (0.0, 0.7, and 4.8 days). However, the second and third TES (2nd TES and 3rd TES) in CD4 were 20.8 and 20.3 days, which were smaller than those in CD5 (25.5 and 27.0 days) and CD6 (31.3 and 33.9 days). Thus, as the crown diameter of initial propagules increased, the TPE decreased but the TES increased. The early stage of propagule growth in clonal propagation is more important for securing abundant resources than the later stages (Lopp and Sammul, 2017), and a bigger propagule in clonal propagation has a greater resource pool than a small one (Klimešová et al., 2011). A recently separated runner plant in CD4 can barely grow independently on its own roots due to its small size, and both it and its runner tip need resources to grow (Park et al., 2018). The TPCs and TPEs in CD4 might be greater than those in CD5 and CD6 because the propagules in CD4 have insufficient resources, unlike those in CD5 and CD6.
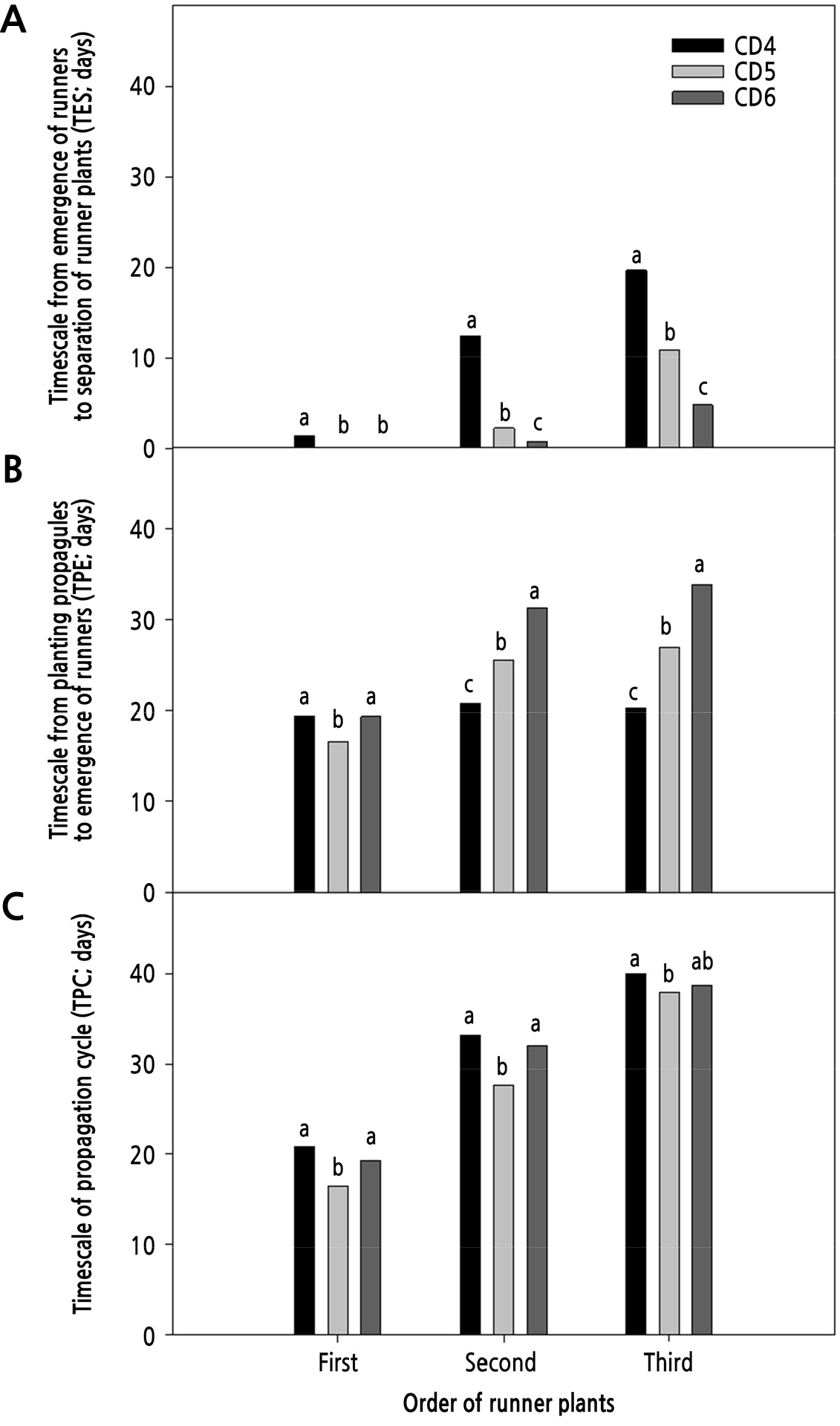
Fig. 4.
Timescales from planting propagules to emergence of runners (A), from emergence of runners to separation of runner plants (B), and of the propagation cycle (C) as affected by crown diameter in a plant factory with artificial lighting for transplant production for 90 days. Means above each bar followed by the same letters are not significantly different according to Duncan’s multiple range test at p < 0.05.
The first TES in CD5 and CD6 was smaller than the second and third; however, the TES in CD4 was similar to those in the second and third. All runner plants used as plant materials had at least one runner at study onset. However, the bract of the first runners in CD5 and CD6 had already unfolded before fixing the runner tips, whereas that in CD4 had not. Therefore, the TES may lengthen as the crown dimeter of propagules increases in the case with simultaneously emerging runners of the propagules.
For 90 days, propagules produced three runner plants and then were removed from the T-PFAL, until the fourth propagation generation (PG4; Fig. 5). The TESs and TPCs of PG1 were smaller than those in other PGs. The first TPEs in CD5 and CD6 were zero due to runner plants with a runner used as propagules, and the second and third TPEs were not significantly different from those in CD4. The TPCs in CD6 increased as PG progressed; however, those in CD5 were not significantly different from PG2 to PG4 except for the first TPC of PG2. The second TPC and third TPC of PG2 in CD4 were greater than those of other PGs in the treatments. The allocation of resources from stock plants to their runner plants is dependent on the size of the stock plants and spacer length between the stock plants and their runner plants (Batzer et al., 2017). In other words, the resource distribution to runner plants is small when the size of stock plants is small. When runner plants are connected to their stock plants, they use resources from their stock plants until they can grow independently with their own roots (Friedman and Alpert, 1991). Therefore, the TPC of PG2 in CD4 might be greatest because the size of the initial propagule and PG1 in CD4 were smallest. When runner plants remain attached to their stock plants after rooting, resource sharing can increase their combined survival and growth (Price and Marchall, 1999). The number of leaves in propagules of later PGs was greater than that of initial propagules in CD4 and CD6. Stock plants used for producing initial propagules had 6–8 leaves and 10 – 12 mm crown diameter and were bigger than any propagules used in this study. They might have enough resources to support their runner plants used as initial propagules (Table 1); however, the initial propagules might not due to an increasing number of leaves in the runner plants as PG progressed (Fig. 6).
When runner plants were separated from their propagules and used as new propagules, the numbers of leaves and runners in CD6 were highest (Fig. 6). The numbers of leaves in CD4 and CD5 increased as PG progressed, and the number of runners in CD4 was not significantly different with progression of PG; however, that in CD5 decreased as PG progressed. Crown diameter of the propagules producing three runner plants was similar to that of transplants or stock plants in the conventional methods (RDA, 2018), and growth of the propagules increased as initial propagule crown diameter increased. However, growth in CD4 and CD5 was not significantly different except that of the fresh weight of leaves (Table 1).
Table 1.
Growth of propagules producing three runner plants as affected by their crown diameter during 90 days after planting initial propagules
Treatment code | No. of leaves | Crown diameter (mm) | Fresh weight (g/plant) | Dry weight (g/plant) |
T/R ratio | |||||
Leaves | Crown | Roots | Leaves | Crown | Roots | |||||
CD4 | 6.9 bz | 10.4 b | 12.0 c | 2.0 b | 3.3 b | 2.84 b | 0.31 b | 0.32 b | 10.0 b | |
CD5 | 6.9 b | 10.6 b | 13.5 b | 2.2 b | 3.6 ab | 3.12 b | 0.39 ab | 0.35 b | 10.4 ab | |
CD6 | 7.7 a | 11.1 a | 16.2 a | 2.7 a | 3.9 a | 3.91 a | 0.43 a | 0.41 a | 10.8 a |
Fig. 3 shows a propagation rate in CD5 using the ATPM of 0.55 plants/day/propagule, which was 3.1- and 2.0-fold greater than those using conventional methods in a greenhouse and a PFAL (Kim et al., 1999; Kim et al., 2010). Strawberry transplants in the conventional methods are mostly produced using runner chains, and runner plants are connected to their stock plants throughout the transplant production period (RDA, 2018). During connection of stock plants and their runner plants, photosynthates and ions tend to transfer from the stock plants to their runner plants (Caraco and Kelly, 1991). Moreover, the runner production rate of stock plants is improved with surplus resources (Alpert, 1991). Due to connection of the stock plants and their runner plants, the resources might move to their runner plants, and then they might lack resources for runner production in the conventional methods despite growing in the PFAL. Therefore, the ATPM is a suitable propagation method for the PFAL.
In the ATPM, the runner plants to be used as new propagules are separated from their propagules when their crown diameter reaches the initial crown diameter of the propagules (Chun, 2016). Park et al. (2018) assumed that propagation rate might increase as the size of propagules decreased due to shortening the TPC. In this study, the numbers of runner plants and the TPC in CD6 were smaller and greater than those in CD5, respectively, as in the hypothesis (Figs. 3 and 4). For that reason, the runner plants in CD6 were too large to use as propagules. On the other hand, the runner plants in CD4 were too small to use as propagules. Unlike the hypothesis of Park et al. (2018), numbers of runner plants and the TPC in CD4 were smaller and greater than those in CD5, respectively, even though propagule size in CD4 was smaller than that in CD5. Park et al. (2018) showed that crown diameter of runner plants 10 days after fixing of runner tips with an unfolded bract was approximately 4 mm and the runner plants died after being separated from their stock plants. The runner plants in CD4 might be too small to grow independently. Therefore, we determined that runner plants with an approximately 5 mm crown diameter are suitable as propagules for the ATPM.
The presented results indicate that propagation cycles and transplant production rates in the ATPM are affected by initial propagule size, and propagules having an approximately 5 mm crown diameter, two leaves, and one runner seem to be appropriate for the ATPM to rapidly produce uniform transplants in a T-PFAL. This study showed that the ATPM is an appropriate vegetative propagation method using PFAL. If the ATPM is applied to systems for production, propagation, and distribution of disease-free stocks in the future, the production rate of higher-grade propagules in the system will be maximized, and the distribution rate of new cultivars will increase.