Introduction
Materials and Methods
Plant Materials and Treatments
Fruit Quality Assessment
Extraction of Cell Wall Hydrolases
Activities of the Cell Wall Hydrolases
Experimental Design and Statistical Analysis
Results
Discussion
Introduction
Peaches (Prunus persica L.), one of the most popular fruits around the world, are widely cultivated in temperate regions (Lurie and Crisosto, 2005). However, the peach fruits are highly perishable, it softens easily and deteriorates very quickly at ambient temperature (Xi et al., 2012). Because of the physiological characteristics of rapid softening after harvest, a low temperature storage system is generally used to delay ripening and extend the postharvest storability of peach fruit (Nunes, 2008).
The ripening of climacteric peach fruit is accompanied by the robust production of ethylene and increased respiration rate (Cai et al., 2018). During postharvest storage, ethylene is known to accelerate quality loss, reduce nutrient composition, and induce physiological disorders in fruits and vegetables (Martinez-Romero et al., 2007). Therefore, the control of ethylene production during storage plays a fundamental role in reducing postharvest commodity loss and maintaining the quality of specialty crops. The ethylene action and perception inhibitor, 1-methylcyclopropene (1-MCP), has profound effects on inhibiting the ripening and senescence of numerous horticultural crops. 1-MCP is registered and commercialized as SmartFreshTM for commercial applications, and its effects have been reported in many types of fruits and vegetables (Watkins, 2006; Dutra et al., 2018; Kim et al., 2018). Recently, 1-MCP formulations have been developed for spraying in the field or for postharvest fruit dipping, and their efficacies were reported in apple, tomato, and avocado fruits (Watkins, 2006; Choi et al., 2008; Yoo et al., 2013, Yoo et al., 2015). However, the effectiveness of different formula of 1-MCP applications on peach fruit is not fully understood yet.
Fruit ripening is accompanied by softening due to primary changes in the structural components of the cell wall (Sethu et al., 1996). Cell-wall degrading enzymes include polygalacturonase, cellulase, pectin methyl esterase, α-galactosidase, and β-galactosidase (Fischer and Bennett, 1991; Brummell et al., 2004). In addition, β-Gal and α-arabinofuranosidase are two major glycosidases that may remove galactosyl and arabinosyl residues from cell wall polysaccharides (Wei et al., 2010; Chang et al., 2017). The firmness loss is also associated with changes in the enzymatic activity of cell wall hydrolases (Chang et al., 1999). Therefore, the objective of this study was to evaluate the comparative effects of preharvest sprayable 1-MCP or postharvest fumigable 1-MCP treatments on fruit quality attributes and the enzymatic activities of cell wall hydrolases in peaches during cold storage.
Materials and Methods
Plant Materials and Treatments
On August 18, 2017, the ‘HetsalHaunkeybee’ (harvest date, middle or late of Augest; fruit weight, 300 - 350 g; soluble solid content, 14。Brix) peach fruit were harvested from the commercial orchard in Gyeongsan, Republic of Korea. Similarly size of fruits that were free from infection and mechanical damage were selected. This experiment included three groups, which consisted of untreated control, preharvest 1-MCP spray, and postharvest 1-MCP fumigation. For the preharvest treatment, 125 mg·L-1 of HarvistaTM (AgroFresh Co., Yakima, WA, USA; 3.8% active ingredient) was sprayed (Watkins et al., 2010; Yoo et al., 2018). For the postharvest treatment, the peach fruit was fumigated with 1 µL·L-1 of SmartFreshTM (AgroFresh Co., Yakima, WA, USA; 3.3% active ingredient). The preharvest treatment was applied to the selected peach fruit 1 week before the harvest on August 4, 2017. The SmartFreshTM treatment was applied for 18 h in an enclosed container after the harvest. The treated fruit was stored together at 10°C and 90 - 95% relative humidity for up to 12 days.
Fruit Quality Assessment
The loss of fresh weight in each treatment group during the cold storage was measured in 9 peaches with three days of interval throughout the storage period. For the measurement of ethylene production, the fruits were placed in an enclosed 1.6 L container for 1 hr, and 1 mL of headspace gas was withdrawn from an enclosed container through the septum and then injected into a gas chromatograph (GC-2010; Shimadzu Co., Tokyo, Japan) with an activated alumina column and flame ionization detector. The analyses for the injector and detector temperatures were run at 200°C with oven temperature at 90°C.
Flesh firmness was evaluated using a rheometer (Compac-100Ⅱ, Sun Scientific Co., Tokyo, Japan). The juice from each fruit was used to determine the changes in titratable acidity (TA) and soluble solids content (SSC) during cold storage. TA was measured by titrating with 0.1N NaOH and malic acid was used for the calculation (DL-15; Mettler Toledo Co., Greifensee, Switzerland). SSC was measured using a refractometer (PR-201α; ATAGO, Tokyo, Japan).
Peel color variables (L*, a*, b*, and h°) were measured 3 times each on the sunny and background sides at the equator regions of individual fruit immediately after harvest and every 3 days after harvest for 12 days during cold storage by using a chromameter (CR-200; Minolta Co., Tokyo, Japan) (McGuire, 1992).
Extraction of Cell Wall Hydrolases
The extraction of cell wall hydrolases was measured by the Pressey (1983) method. All steps for the extraction were conducted at 4°C. For the extraction, 50 g of frozen tissue was homogenized with 100 mL of 10 mM sodium phosphate buffer (pH 7.0) in which 75 mg of polyvinylpolypyrrolidone was included. We added 8.775 g of NaCl to the final concentration of the extracted samples, and stirred the solution for 3 hr. The incubated suspension was then centrifuged at 12,000 × g for 1 hr, and 85% (v/v) ammonium sulfate was added to each supernatant and stirred overnight. Then, the supernatant was centrifuged again, and the pellets were collected and dissolved in 10 mM sodium phosphate buffer and allowed to dialyze for 2 days. The final supernatant was centrifuged at 22,000 × g for 1 hr, and the crude extract was stored at - 80°C until analysis.
Activities of the Cell Wall Hydrolases
The enzymatic activities of α-galactosidase (α-Gal), β-galactosidase (β-Gal), β-glucosidase (β-Glu), β-xylosidase (β-Xyl), β-arabinosidase (β-Ara), and α-mannosidase (α-Man) were determined by measuring the amount of p-nitrophenol released from p-nitrophenyl pyranoside (Pressey, 1983; Brummell et al., 2004). Approximately 250 µL of the extracted solution was added to 250 µL of 10 mM sodium acetate buffer (pH 4.0) in a tube. Next, 125 µL of 2% p-nitrophenyl pyranoside was added, and the mixture was incubated at 30°C for 1 hr. The reaction was started by adding 1 mL of 1 M sodium carbonate, and the absorbance was read at 410 nm by using a UV-spectrophotometer (UV-1800; Shimadzu Co., Tokyo, Japan). One unit of the enzyme was defined as the amount of activity that released 100 µmol of p-nitrophenol at 30°C for 1 hr.
Experimental Design and Statistical Analysis
All the trees used in this experiment were placed using a completely randomized design. The experiments were performed in triplicate. The data for the statistical analysis were subjected to two-way analysis of variance, and mean differences were analyzed using Duncan’s multiple range tests at p = 0.05. The statistical software SPSS (IBM SPSS Statistics 23; SPSS Inc., New York, USA) was used for the statistical analysis. Pearson correlation coefficients were obtained using SPSS to test associations between fruit quality attributes and cell wall hydrolase activities during cold storage. The correlation analysis was performed with three replications (n = 3). Asterisks (* or **) indicate a statistically significant correlation at p < 0.05 or 0.01, respectively.
Results
The preharvest 1-MCP treatment resulted in higher weight loss during cold storage than that of postharvest treatment and untreated control (p < 0.01). Weight loss was significantly (p < 0.01) lower in postharvest 1-MCP-treated peach fruits, compared with the others (Fig. 1). Ethylene production in pre- and postharvest 1-MCP-treated fruit was slightly lower, compared to that in the untreated control. Flesh firmness was used to monitor the physiological process of ripening. At harvest time, the fruit that received the preharvest 1-MCP treatment showed higher firmness than the fruit in the other groups, however, they showed a similar rate of firmness loss as with the untreated fruit during cold storage. The fruit that received the postharvest 1-MCP treatment showed a higher firmness during cold storage up until 9 days. The SSC of the fruit that received the preharvest 1-MCP treatment was significantly higher than that of the control fruit at harvest (p < 0.05). The postharvest 1-MCP treatment significantly enhanced the SSC of the peaches during cold storage; the SSC was higher in the postharvest 1-MCP treatment than in the control at the end of cold storage. The TA in all treatments groups was variable, however, the peaches treated with postharvest 1-MCP showed significantly higher TA than the peaches in the control group at the end of cold storage (Fig. 1).
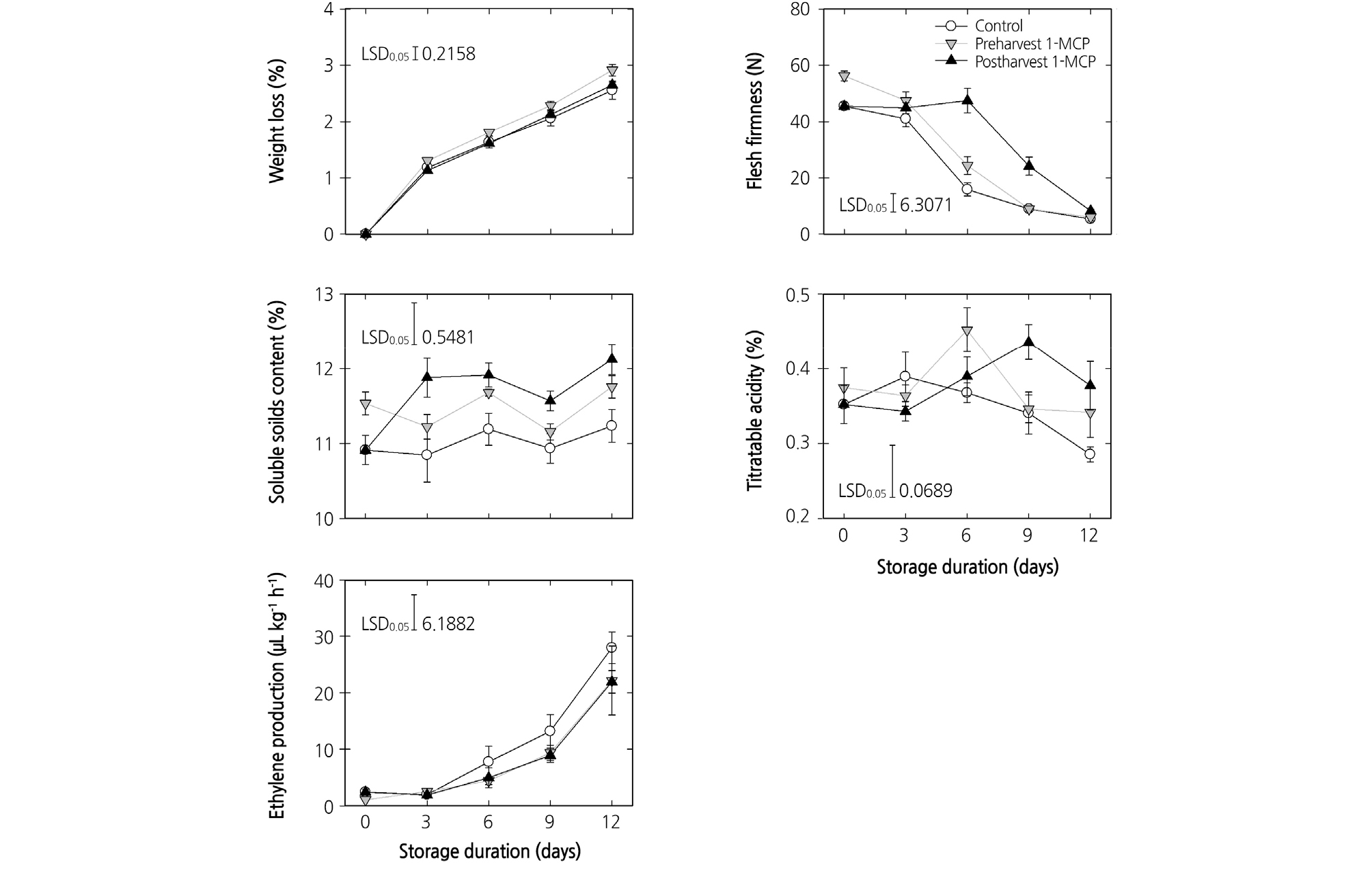
Fig. 1.
Effects of preharvest or postharvest 1-methylcyclopropene (1-MCP) treatments on weight loss, flesh firmness, soluble solids content, titratable acidity, and ethylene production of cold-stored ‘HetsalHaunkeybee’ peach fruit. All values are expressed as mean ± standard error (n = 9). Preharvest 1-MCP (125 mg·L-1) treatment was applied to the selected peach fruit 1 week before the harvest. Postharvest 1-MCP (1 µL·L-1) treatment was applied for 18 h in an enclosed container after harvest.
The peel color of the peaches changed from green to yellow as the fruit ripened. The pre- or postharvest 1-MCP treatments did not significantly alter the peel color variables (L*, lightness; a*, redness; b*, yellowness) of both the sunny and background sides of the peach fruit at harvest and during cold storage (Fig. 2). The preharvest 1-MCP application kept the hue angle (h°) high up until 6 days after harvest. Then, the hue angle of the fruit in all treatment groups decreased to similar levels at the end of cold storage.
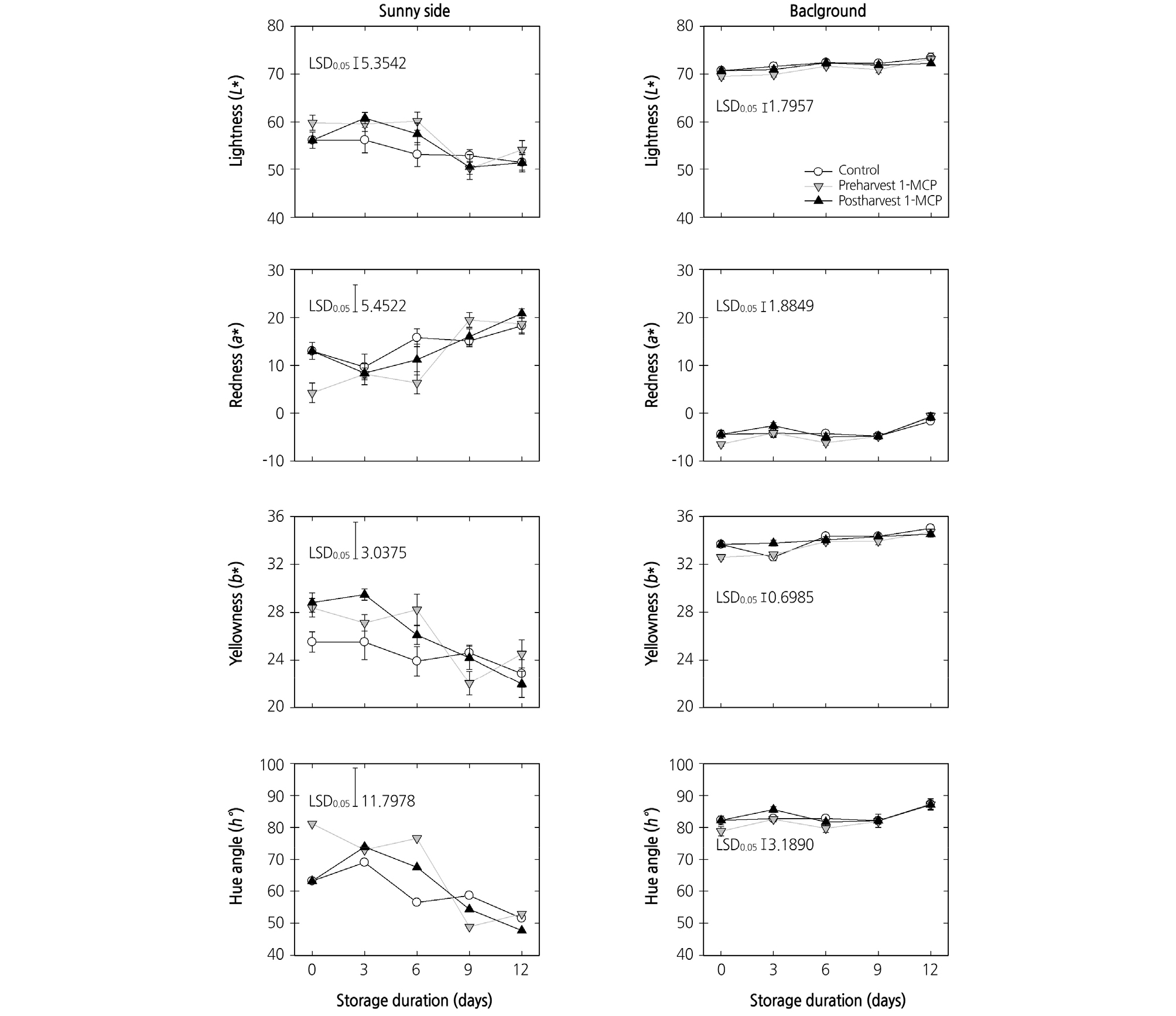
Fig. 2.
Effects of preharvest or postharvest 1-methylcyclopropene (1-MCP) treatments on the color variables (L*, a*, b*, and h°) of sunny side and background peel tissues in cold-stored ‘HetsalHaunkeybee’ peach fruit. All values were expressed as mean ± standard error (n = 9). Preharvest 1-MCP (125 mg·L-1) treatment was applied to the selected peach fruit 1 week before harvest. Postharvest 1-MCP (1 µL·L-1) treatment was applied for 18 h in an enclosed container after harvest.
Changes in the activities of the enzymes β-Gal, α-Gal, β-Glu, β-Ara, β-Xyl, and α-Man from harvest to the end of cold storage were presented in Fig. 3. During cold storage, all of the tested enzyme activities increased. At harvest, the untreated control and 1-MCP-treated fruit showed similar enzymatic activities. The fruit treated with postharvest 1-MCP treatment showed the lowest increase in all tested enzymatic activities (Fig. 3).
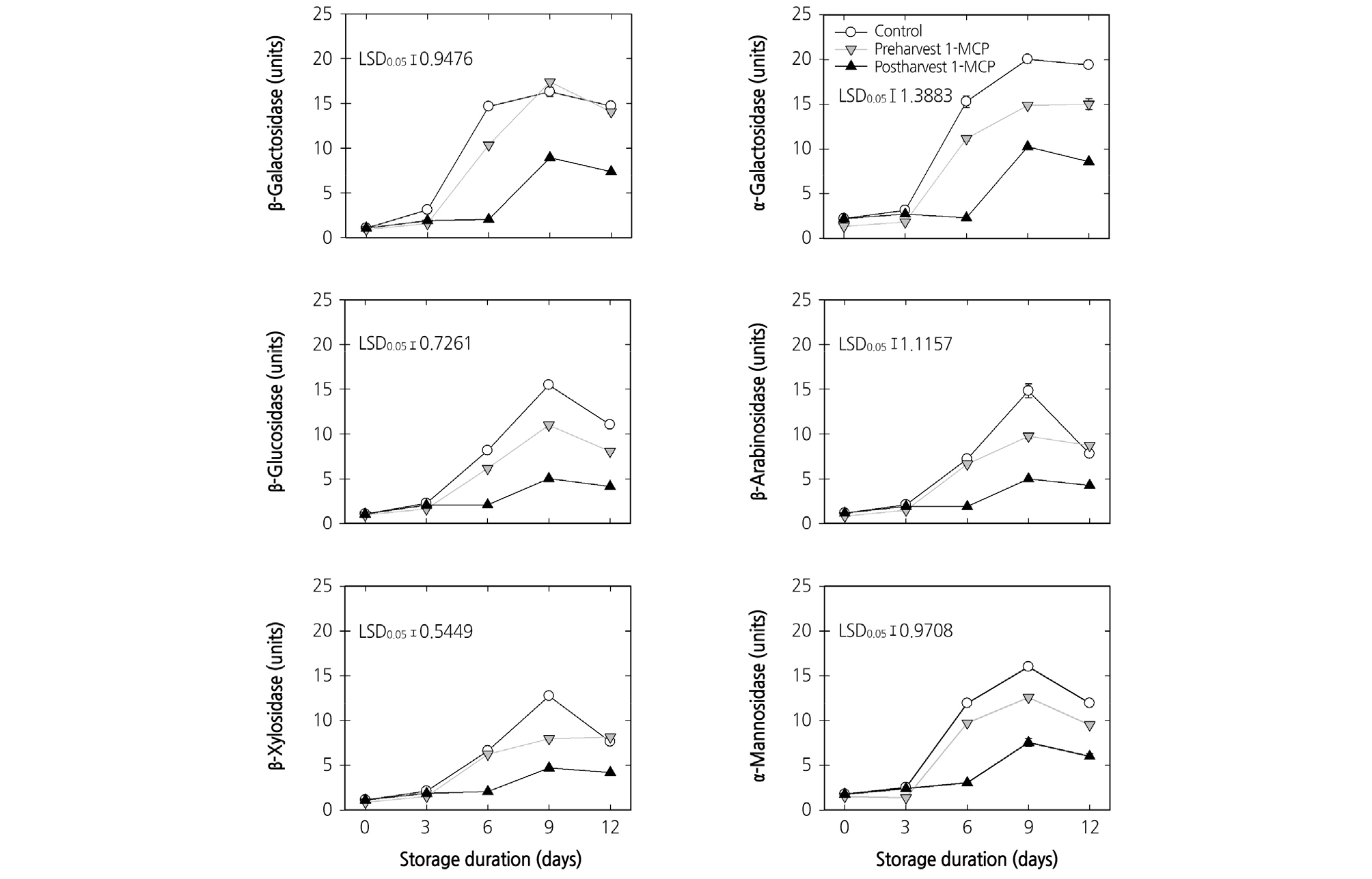
Fig. 3.
Effects of preharvest or postharvest 1-methylcyclopropene (1-MCP) treatments on the enzymatic activities of cell wall hydrolases in cold-stored ‘HetsalHaunkeybee’ peaches. All values are expressed as mean ± standard error (n = 9). Preharvest 1-MCP (125 mg·L-1) treatment was applied to the selected peach fruit 1 week before the harvest. The postharvest 1-MCP (1 µL·L-1) treatment was applied for 18 h in an enclosed container after harvest. One unit of the enzyme activity was defined as the amount of activity that released 100 µmol of ρ-nitrophenyl at 30°C for 1 hr.
Of those quality attributes, different correlations resulted from the preharvest and postharvest 1-MCP treatments (Fig. 4). The pre- and postharvest 1-MCP treatment altered the correlation of TA and SSC with several fruit quality-associated attributes. The TA of the control fruit was positively correlated with flesh firmness; however, it disappeared in the 1-MCP-treated fruit. The level of SSC showed a significant correlation with fruit color (L*, a*, and b*) in the untreated peaches; however, 1-MCP-treated fruit did not show a correlation between SSC and fruit color. Regardless of the treatment, flesh firmness showed the most significantly negative correlation with enzymatic activity. The enzymatic activity of the fruit treated with postharvest 1-MCP treatment showed a higher positive correlation with ethylene production than that of the fruit in the other groups (Fig. 4).
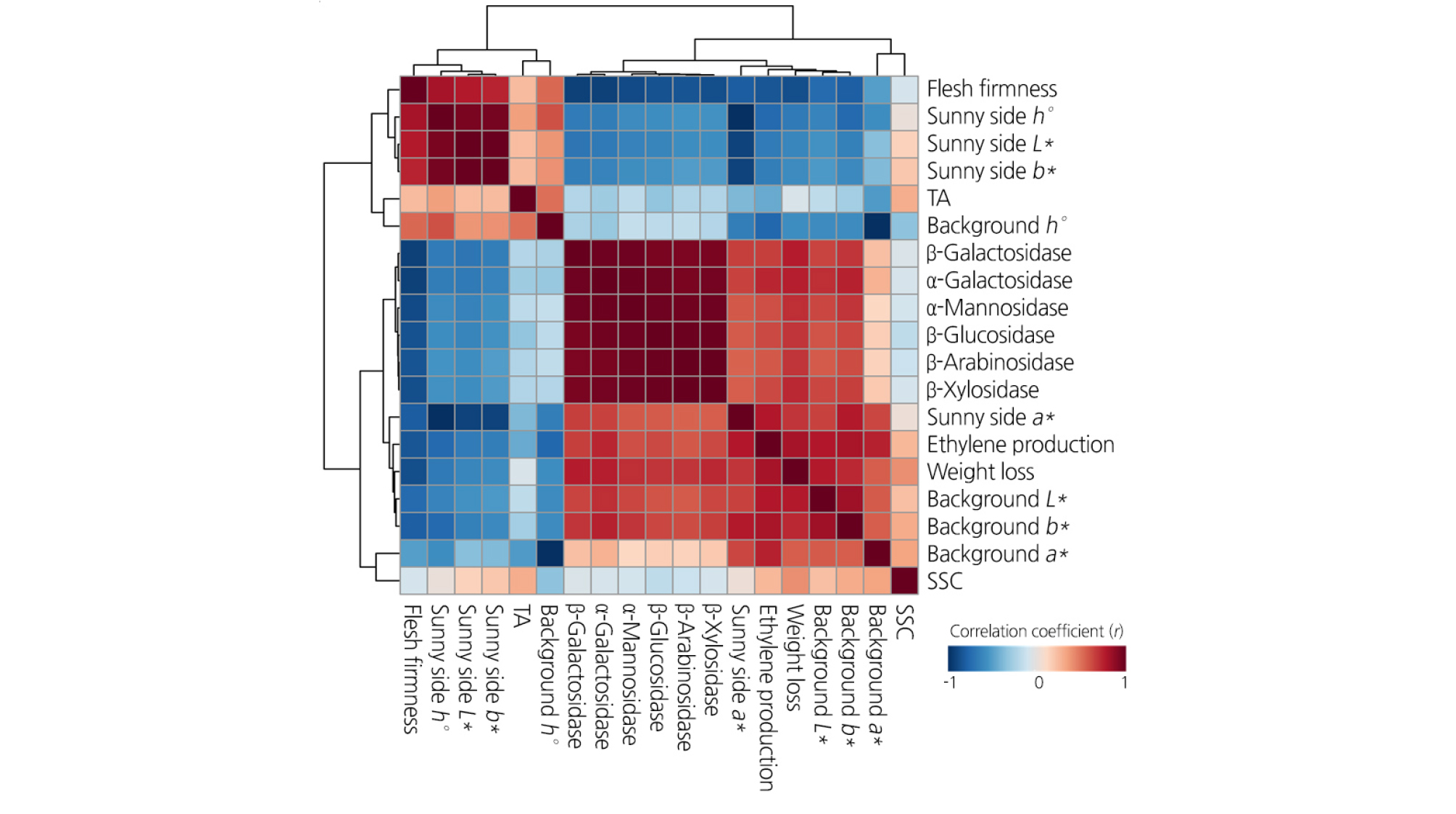
Fig. 4.
Correlation coefficients (r) between fruit quality attributes and enzymatic activities of cell wall hydrolases of cold-stored ‘HetsalHaunkeybee’ peaches treated with preharvest or postharvest 1-MCP treatments. The red and blue colors indicate positive and negative correlations between fruit quality attributes and enzymatic activities of cell wall hydrolases, respectively.
Discussion
In this study, the effects of preharvest and postharvest 1-MCP treatments on different fruit quality attributes and the enzymatic activity of peach fruits were tested. The overall production of ethylene decreased among the peaches treated with 1-MCP regardless of treatment method. A previous study showed that ethylene production was inhibited by 1-MCP treatment in ‘Elberta’ peach fruit stored at 20°C (Fan et al., 2002). Reportedly, peach fruit treated with 1-MCP showed delayed softening during their shelf life (Bregoli et al., 2005; Liu et al., 2005). Consistently, postharvest 1-MCP treatment in the study extended peach shelf life by enhancing flesh firmness. However, the preharvest 1-MCP treatment did not delay the decrease in flesh firmness during storage. Interestingly, the preharvest 1-MCP treatment increased the flesh firmness of the peach fruit at harvest time, but this group showed similar levels of flesh firmness as the untreated control group 6 days after harvest during cold storage. In the postharvest 1-MCP-treated peach fruit, slightly enhanced firmness was observed up until 6 days after treatment. These results suggested that 1-MCP might have protective effects on peach fruit for about 10 days. Thus, postharvest treatment of 1-MCP may be needed to control the ripening and harvesting time of fruit further to extend the shelf life of peach fruit
Regardless of the treatment time, studies have shown that postharvest 1-MCP treatment maintains peel color variables when compared with control fruit during shelf life (Kluge and Jacomino, 2002; Chun et al., 2010). However, regardless of the pre- and postharvest 1-MCP treatments in this study, the peel color variables of the sunny and background sides of the fruit were not different.
SSC was not affected by preharvest 1-MCP treatment. However, the postharvest 1-MCP treatment slightly increased the SSC at the end of the cold storage period compared with the control. Similar results were reported by Liu et al. (2005), Liu et al. (2015), who showed that the reduction of TA during peach fruit storage was delayed by 1-MCP treatment. Similarly, the reduction of TA measured at the end of the cold storage period in this study was delayed by postharvest 1-MCP treatment compared with the control fruit.
Softening and enzymatic activity are closely related to ethylene production in climacteric fruits, and they are mainly the result of changes in cell wall-degrading enzymes in peach fruits (Chang et al., 2017). In this experiment, the activities of cell wall hydrolases, such as α-Gal, β-Gal, β-Glu, β-Xyl, β-Ara, and α-Man were analyzed.
Postharvest 1-MCP treatment more effectively reduced the activities of cell wall hydrolases of the peaches than did preharvest treatment or untreated control. It is known that the increased enzymatic activity of peach fruit occurs after the initiation of the softening process and textural changes in mesocarp tissue (Chang et al., 1999; Ruiz et al., 2010). Similar increases of enzymatic activity results were observed at the end of storage in this experiment. Some studies have reported that β-Gal is associated with the removal of galactosyl residues from cell wall polymers during softening, leading to ripening-related firmness loss (Payasi et al., 2009; Wei et al., 2010). Correspondingly, postharvest 1-MCP treatment maintained the higher flesh firmness of the ‘HetsalHaunkeybee’ peaches and decreased their enzymatic activity at the end of storage. However, the relationship between ethylene production and cell wall enzymatic activity was not closely observed in this experiment.
To evaluate the overall results of fruit quality attributes after the preharvest and postharvest 1-MCP treatments, a correlation coefficient heat map was used. Positive and negative correlations of TA with firmness and enzymatic activity were observed. Furthermore, the enzymatic activity of the fruit that received the postharvest 1-MCP treatment showed a higher correlation with ethylene production than that by the preharvest and untreated groups. The results presented here indicate that postharvest 1-MCP treatment can help retain fruit quality by altering the level of TA, firmness, and ethylene production.