Introduction
Materials and Methods
Plant Materials
Measurements and Data Analysis
Statistical Analysis
Results
Shoot Growth
Ornamental Value
Stomatal Morphology
Chlorophyll Fluorescence
Discussion
Influence of Temperature on Petunia Development
Influence of High Relative Humidity on Petunia Growth and Ornamental Value
Introduction
Temperature can regulate plant growth and development by influencing developmental stages, such as germination, leaf unfolding, and flowering. Flower development rates in different bedding plants increase with rising temperatures that range from the base temperature to the optimal temperature (Topt) (Blanchard and Runkle, 2011; Vaid and Runkle, 2013). When temperatures are above Topt, the rate of flower development begins to decline (Whitman et al., 1997; Yuan et al., 1998). Temperature not only influences the developmental rate of plants, but also petal pigmentation in flowers. In Oriental hybrid lilies, researchers found that LhMYB12 transcription was suppressed when plants were grown at 35°C for two days (Lai et al., 2011) and reduced the accumulation of anthocyanin in lily petals. These results indicate that high temperature conditions cause a discoloration phenomenon in petal pigmentation, which leads to a decrease in the ornamental value of these flowers.
Under high relative humidity (RH) conditions, pot roses increased in height (Mortensen et al., 2001), and leaf number and area of Hydrangea macrophylla increased significantly (Codarin et al., 2006). High RH also induces changes in stomatal morphology, including longer and wider stomata. An increase in stomatal density was found in roses (Torre et al., 2003; Fanourakis et al., 2013) and chrysanthemums (Aliniaeifard and van Meetern, 2016) when grown in high humidity conditions. However, Nejad and van Meetern (2005) reported a decrease in stomatal density in Tradescantia virginiana when plants were grown in high RH. These results suggest that in general, stomata become larger when grown under high RH, but the change in stomatal density in response to RH can vary between plant species. The stomata of plants grown under high RH conditions were less sensitive to water stress, darkness, and other closing stimuli (Torre and Fjeld, 2001; Fanourakis et al., 2013). These results indicated that high RH induces stomatal malfunction, which can contribute to stomata that do not close or only close partially in response to drought or darkness.
Among bedding plant species, petunias are one of the most popular plants for their wide range of colors (Park et al., 2016). In 2015, the wholesale value of petunias in the USA totaled $133.241 million, ranking first at approximately 10.4% of the total wholesale value of annual bedding and garden plants (USDA, 2016). Most modern Petunia cultivars are hybrids that have been derived from P. integrifolia and P. axillaris (Stehmann et al., 2009). Petunias are heat-tolerant species and could adapt well to tropical climates. However, most research on the effects of high temperature in petunias has been conducted under optimal RH conditions for plant growth, while natural tropical climates are usually characterized by both high temperature and humidity. Most research has been conducted under conditions of lower humidity because high humidity can easily cause the proliferation of bacterial or fungal pathogens, including mold, which can affect experimental results. Hence, the adaptability of petunias to tropical climates and the ways in which the interaction between high temperature and high humidity affects plant growth still need to be studied. Therefore, we report the growth and development of petunias in response to rising temperatures under high humidity conditions in order to evaluate the adaptability of petunias to a hot and humid climate.
Materials and Methods
Plant Materials
Petunia ‘Madness Red’ was used as plant material in this study. Petunia seeds were sown in 72 cell plug flats filled with commercial growth medium. They were germinated in 25°C air temperature (AT) in environmentally-controlled growth cabinets (CO2 Growth Chamber HB-301SP-O, Hanbaek Scientific Co., Ltd., Bucheon, Korea) using a 16-h photoperiod with a photosynthetic photon flux density (PPFD) of 200 ± 50 μmol·m-2 ·s-1 provided by white LED lights, and 50 ± 30 μmol·m-2 ·s-1 provided by high-pressure sodium lamps. During the plug stage, RH was maintained at 50 ± 3%, and the seedlings were irrigated as necessary with a nutrient solution (Green-care Fertilizers, Chicago, USA) with an electric conductivity (EC) of 1.2 ± 0.1 dS·m-1 and a pH level of 6.0 ± 0.1. When four to six true leaves had emerged, seedlings were transplanted into 9 cm round plastic containers with a 200 mL volume. Plants were grown under the plug stage environmental conditions for two days and then fifteen plants were transferred into four separate environmentally controlled growth cabinets for the Horticultural Science and Technology experiment. Plants were grown over a 16-h photoperiod, with the same PPFD as in the plug stage. The difference between daytime and nighttime temperatures (DIF) in this experiment was set at 5°C. The irrigation schedule was maintained. The environmental data were recorded every 30 min by a data logger (HOBO, Onset Computer Corp., USA).
Measurements and Data Analysis
The date of the first open flower per plant was recorded. Time to the first flower of each plant was calculated from the start of treatment to the date of the first open flower. Plants were considered flowering when the flower corolla had fully opened. At the first flowering of 10 random plants per treatment, the number of leaves (> 1 cm long), the number of visible buds, the first flower diameter, and the fresh weight of shoots and roots were recorded. The dry weight of shoots and roots was determined after drying the samples in a dry oven for three days at 70°C.
To investigate the accumulation of flowers in each treatment, three plants were chosen randomly and observed for 4 weeks from the start of the experiment. Numbers of newly open flowers were recorded every day. Total leaf area (LA) per plant at first flowering was measured with a leaf area meter (LI-3100C Area Meter, LI-COR Biosciences, USA). To investigate the influence of temperature on flower color, a CR-10 Plus Color Reader (Konica Minolta Sensing Inc., Japan) was used to determine flower color measurements. The carbohydrate content in the dry matter was measured by determining the level of D-glucose in a sample (Kim et al., 2006). The absorbance of D-glucose was measured at 625 nm with UV-VIS spectrophotometer (Shimadzu Scientific Instruments Inc., USA).
To observe stomatal morphology and density, leaf samples were cut into 0.5 × 1 cm slices for scanning electron microscopy (SEM) pretreatment and analysis. Karnovsky’s fixation and a mixture of 2% osmium tetroxide and 0.1 M cacodylate buffer were used for fixation. After fixation, samples were dehydrated with 30, 50, 70, 80, 90, and 100% ethanol. Finally, the dehydrated samples were immersed in hexamethyldisilazane twice and then mounted onto a sample stub. The dried samples were coated with platinum and observed with SEM (FESEM AURIGA, Carl Zeiss Co., Germany).
The chlorophyll fluorescence of photosystem II was measured at first flowering with a closed FluorCam 800MF (Photon System Instruments Ltd., Czech Republic). Plants were irrigated regularly to ensure they did not experience water stress. Under dark conditions, measurements were taken using a quenching kinetics analysis method (Maxwell and Johnson, 2000).
The measuring conditions were automatically adjusted to the camera electronic shutter and ambient light levels. The data were analyzed using FluorCam 7 software (FluorCam 7 software, Photon System Instruments Ltd., Czech Republic).
Statistical Analysis
Statistical Analysis System (SAS) version 9.4 (SAS Institute Inc., Cary, NC, USA) was used to analyze the experimental data for multiple comparisons via analysis of variance (ANOVA). Differences between the means from the treatments were assessed with Duncan’s new multiple range test at p ≤ 0.05.
Results
Shoot Growth
At 25°C AT, the number of leaves increased approximately 12.4%, from 79.8 to 89.7 leaves (Table 1). The leaf area per plant was also higher when grown in 80% RH. Conversely, leaf area decreased significantly as the AT increased from 25°C to 35°C in 80% RH conditions. In particular, at 35°C AT, plants produced smaller or curled leaves, resulting in a decrease in leaf area. However, the leaf number was not affected by a temperature change from 25°C to 35°C AT.
Regardless of temperature, plants had higher shoot biomass in 80% RH than in 50% RH. Moreover, plants that were grown at 25°C in 80% RH showed significantly higher root biomass as well (Table 1). This finding is consistent with the previous reporting that high humidity can enhance the development of root biomass and root length in cucumbers (Barker et al., 1986). In 80% RH, root biomass declined as air temperature rose from 25°C to 35°C AT. Crawford et al. (2012) also reported that elevated temperatures did not promote biomass accumulation in roots.
The carbohydrate content of the total dry weight of plants was significantly higher in 80% RH than for those grown in 50% RH. However, the carbohydrate content decreased approximately 11% and 29% when plants were grown at 30°C and 35°C AT, respectively. High evapotranspiration rates at higher temperatures could explain the decrease in dry weight, and result in lower carbohydrate content.
Ornamental Value
At 25°C, as RH increased from 50 to 80% the number of days to first flowering (DTF) increased by approximately 1.6 d. There was no difference in the number of visible buds between the two RH treatments. Higher air temperatures accelerate photosynthetic activity and assimilation rate, resulting in shorter vegetative growth phases and earlier flowering in plants. Under 80% RH, plants grown at 30°C and 35°C took 13.7 d and 12.9 d to flower, respectively, which reflected a decrease in flowering time of 3.0 d and 3.8 d, respectively, compared to plants grown at 25°C (Table 2). At higher temperatures, the visible flower bud number also increased from 25.3 to 30.8% compared to plants grown at 25°C.
At 25°C, RH had no obvious effect on flower color. however, the larger diameter of flowers grown in 80% RH compared to 50% RH conditions might be related to the increase in cell number and cell expansion. In this study, the Lab color space values a*, b*, and h* of the flowers decreased significantly at high temperatures. Flowers produced at high temperatures were pale and blue-ish, indicating that discoloration of the flowers had occurred. Flower diameter was also reduced substantially as the temperature increased. A temperature increase from 25°C to 35°C resulted in a reduction in flower diameter from 6.7 to 4.5 cm (Table 2).
Under 80% RH, the accumulated number of flowers on plants grown at 30°C for 4 weeks was nearly three times higher than that of plants grown at 25°C, and 1.5 times higher than that of plants grown at 35°C (Fig. 1). These results indicate that after 4 weeks, plants grown at 30°C AT produced 1.5 new flowers every day, which was three times that of plants grown at 25°C and 1.5 times that of plants grown at 35°C. However, flower longevity was influenced by both temperature and humidity. At 25°C, flower longevity in 80% RH was 6.8 d, while that of flowers produced in 50% RH was 4.9 d. At 30°C, the lifespan of flowers reduced by 40% from 6.8 d to 4.1 d, compared to those grown at 25°C. Finally, flowers of plants grown at 35°C only lasted for only 2.4 d (Fig. 1). Therefore, as temperatures increased in 80% RH conditions, flower longevity declined.
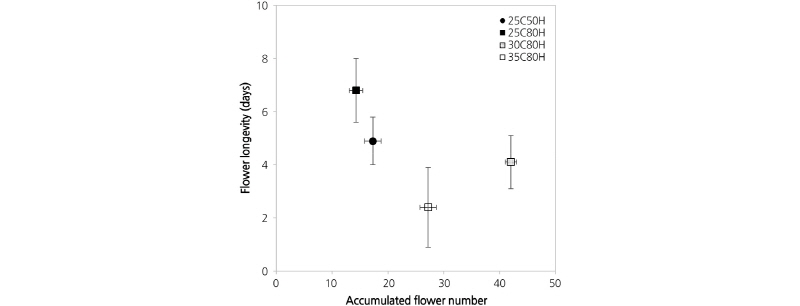
Fig. 1.
The combined effects of air temperature and relative humidity on the accumulated flower number and the longevity of each flower in petunia ‘Madness Red’ during a four-week observation after the first flowering. 25C50H: 25°C air temperature (AT) and 50% relative humidity (RH); 25C80H: 25°C AT and 80% RH; 30C80H: 30°C AT and 80% RH; 35C80H: 35°C AT and 80% RH. Bars represent standard error (n = 50).
Stomatal Morphology
The influence relative humidity has on stomatal density can vary between species (Torre et al., 2003; Fanourakis et al., 2013). At 25°C, we observed no difference in stomatal density between the two RH treatments. This result suggested that RH has no effect on the stomatal density of petunia ‘Madness Red’. Air temperatures of 25°C and 30°C also had no influence on stomatal density in petunia ‘Madness Red’ however, at 35°C the stomatal density increased to three times greater than that measured at 25°C and 30°C (Table 3).
Consistent with previous studies, at 25°C the length of stomata of petunia ‘Madness Red’ increased significantly from 20% to 40% when RH increased from 50% to 80% (Table 3 and Fig. 2). In 80% RH, there were no significant differences found in stomatal length of plants grown at different air temperatures; however, stomatal width increased as the air temperature increased. Compared to leaves grown under 25°C AT conditions, the width of stomata on leaves grown at 30°C and 35°C were 58% and 81% wider, respectively (Table 3). Thus, as AT increased the inner wall thickness of stomata also significantly increased (Fig. 2H).
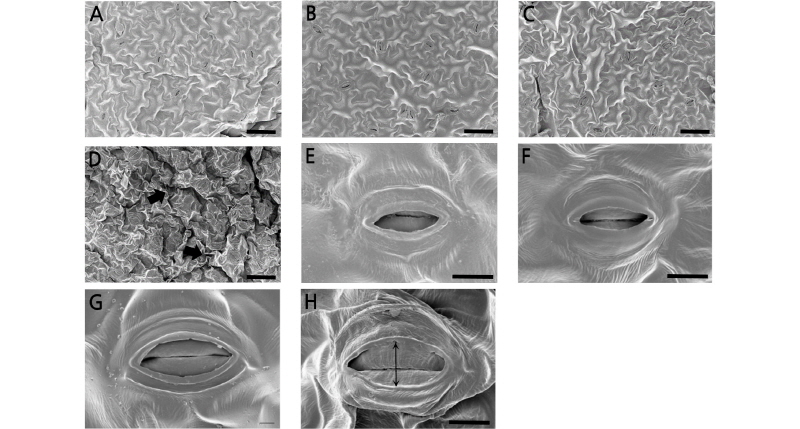
Fig. 2.
The combined effects of air temperature and relative humidity on the stomatal aperture and morphology in petunia ‘Madness Red’ at the flowering stage. Observed with scanning electron microscopy (SEM; bars = 50 μm for A to D and 10 μm for E to H). Arrows show the shrinkage on the leaf surface (D) and the thickness of stomatal inner wall (H). A and E: 25°C AT and 50% RH; B and F: 25°C AT and 80% RH; C and G: 30°C AT and 80% RH; D and H: 35°C AT and 80% RH.
Chlorophyll Fluorescence
At 25°C, there were no statistically significant differences in Fv/Fm, which reflects the maximum quantum yield of photosystem II (PSII) and was equivalent to the maximum photochemical efficiency between the 50% and 80% RH treatments (Fig. 3). However, the Fv/Fm of plants that were grown at 35°C in 80% RH was 1.4 times lower than that of plants grown at 25°C in 50% RH. This indicated that the ability of plants grown in 80% RH to perform photosynthesis was lower than that those grown in 50% RH. The non-photochemical quenching (NPQ) results also showed no difference between the two RH treatments.
Under 80% RH, the Fv/Fm decreased indicating that the photosynthetic ability of plants declined at high temperatures. This result might be related to the decrease in the chlorophyll content (represented by the measured SPAD meter value), which reflects the chlorophyll content in leaves at high temperature, leading to a decline in photosynthetic ability. Moreover, the reaction center of PSII is sensitive to heat; thus, at high temperatures the photochemical efficiency of PSII declines. However, an increase in air temperature had no effect on the results of NPQ, which is commonly used as a stress indicator. Even though there were no significant changes in NPQ values, we cannot conclude that the PSII in petunia leaves did not experience heat stress the under high temperature conditions because the photochemical efficiency of PSII declined and the leaf surfaces of the plants grown under high temperatures shrunk, which might have been caused by heat stress damage (Fig. 2). The decline in the Fv/Fm with unchanged NPQ values might be explained by the quantum yield of NO (non-regulated, non-photochemical quenching in PSII). Hence, the decline in petunia photosynthetic ability in this study could be due to the increase in the quantum yield of NO.
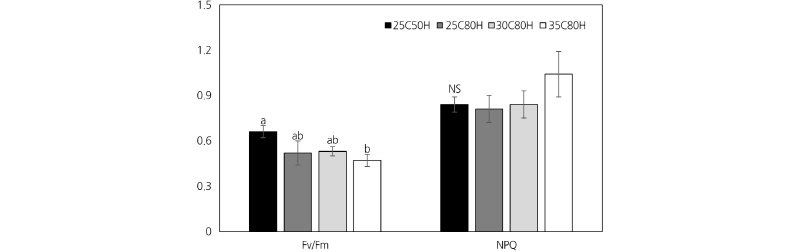
Fig. 3.
The combined effects of air temperature and relative humidity on chlorophyll florescence (Fv/Fm) and non-photochemical quenching (NPQ) in petunia ‘Madness Red’. 25C50H: 25°C air temperature (AT) and 50% relative humidity (RH); 25C80H: 25°C AT and 80% RH; 30C80H: 30°C AT and 80% RH; 35C80H: 35°C AT and 80% RH. NS or vertical bars with the same letter are not significantly different at p ≤ 0.05 by Duncan’s new multiple range test (n = 3).
Discussion
Influence of Temperature on Petunia Development
In general, growth temperatures above 30°C had a negative effect on shoot growth in petunia. Plants grown at 35°C produced visibly smaller leaves and SEM images showed that the leaves of plants grown at 35°C were somewhat shrunken, leading to lower leaf area and increased stomatal density. Leaves might have been smaller in order to reduce the surface area exposed to thermal stress and water loss due to increased transpiration. At temperatures above the Topt for plant development, transpiration and respiration still rapidly increased, and led to a decline in overall plant biomass, and especially in the roots (Table 1). The decrease in root biomass caused a lower ratio of root to shoot dry weight (root:shoot DW ratio), which is used to evaluate plant health. Indeed, from their visual appearance the plants that were grown at 25°C in 80% RH conditions had the highest root:shoot DW ratio, looked more compact, and produced thicker leaves and stems.
High temperatures also affected the ornamental value of flowers including flower size, flower color, and flower longevity. Moderately high temperatures accelerated plant development rates by accelerating photosynthesis, assimilation rate, and other physiological activities that resulted in a shorter vegetative growth phase and earlier flowering(Shin et al., 2001).. However, in plants grown at high temperatures, the respiration rate increased rapidly, which decreased the energy available for flower development and led to the production of smaller flowers. These results are consistent with the results for other species such as pansies (Niu et al., 2000), Impatiens, and Torenia (Warner and Erwin, 2005). Furthermore, higher temperatures were also reported to affect flower pigmentation; Shvarts et al. (1997) reported a significant decline in anthocyanin content in Petunia ×hybrida ‘VR’ (V23 × R51) grown at 32°C compared to those grown at 17°C. This phenomenon is explained by the inhibition of anthocyanin synthesis in high temperature conditions (Yamagishi et al., 2010; Lai et al., 2011).
In a study by Blanchard and Runkle (2011), 11 of 18 species showed a decline in flowering rate when the growing temperature increased to 30°C. These results suggest that the optimal flowering temperature for these 11 species is lower than 30°C, and that when the temperature increased above the specific Topt the flowering rates decreased until no flowering occurred at Tmax. The flowering rate of petunia ‘Madness Red’ in this study increased when the temperature rose from 25°C to 35°C (Table 2). This observation suggests that 35°C is still within the Topt range for flowering in this cultivar. Although plants had low ornamental value at 35°C AT conditions, including long and weak stems, yellowish leaves, small and pale-colored flowers, and significantly decreased flower longevity, no plants died during the experiment. These results indicate that petunias, specifically the Madness Red® series, can develop and flower at temperatures as high as 35°C. Therefore, the petunia Madness Red® series can adapt to high temperatures and high humidity levels common in tropical regions. However, considering their use in decorating and landscaping, these plants should be grown at temperatures below 35°C for best results.
Influence of High Relative Humidity on Petunia Growth and Ornamental Value
In this study, high RH at 25°C had no negative effects on the growth and ornamental value of petunia plants. The increase in leaf area observed in high RH may be caused by increased epidermal cell expansion (Murphy et al., 2013). A previous study also reported that the leaf area of Hydrangea macrophylla L. plants increased in 80% RH conditions compared to that of plants grown under 50% RH conditions (Codarin et al., 2006). Because the stomata of leaves have no need to close in high humidity, the rate of gas exchange increases. Murphy et al. (2013) reported a 215% increase in stomatal conductance in Toona ciliata leaves that were grown in 80% RH, compared to those grown in 20% RH conditions at 25°C. The increased stomatal conductance might lead to an increase in carbon uptake and result in a higher carbohydrate content.
At constant temperature, high RH reduced transpiration rates and resulted in slower water and nutrient uptake in the roots of petunia, leading to a lower plant development rate. Hence, under high RH conditions, plants had a longer vegetative growth phase and later flowering times. These results demonstrate the effects of RH on the ornamental value of the flowers. There was no difference in the number of visible buds between the two RH treatments reported here, which might indicate that higher RH affects the vegetative growth of plants but not the reproductive growth. Moreover, the slower transpiration occurs, the less water is lost through transpiration, which might explain the longer lifespan of flowers grown in 80% RH conditions in this study. Mortensen (2000) observed a significant decrease in the vase life of cut roses grown in 90 ± 5% RH conditions compared to those grown in 65 ± 5% RH. The inconsistency between these results and our findings might exist due to the RH difference between cultivation and postharvest periods. The purpose of our study was to evaluate the growth and ornamental value of petunias as a bedding plant in tropical climates; therefore, the pressure of the RH condition on flower longevity was maintained at a constantly high level and flowers were kept intact to simulate more natural environmental conditions. Because of this setup, flowers did not have to experience water stress caused by the difference in RH between the growth condition and the observational condition. Moreover, the higher carbohydrate content produced in high RH conditions would lead to a longer flower lifespan, as suggested by Shvart et al. (1997).
At a temperature of 25°C, high RH might not be a problem for petunia development because we observed the positive effects of 80% RH conditions on the growth and ornamental value of petunia plants (Table 1 and 2) Nevertheless, at 30°C, temperature and RH might interact to cause a decline in flower longevity. At higher temperatures, the vapor-pressure deficit increases and leads to increased transpiration. Normally, when the transpiration exceeds a certain threshold, stomata close in order to prevent further water loss. however, under high RH conditions an increase in abscisic acid (ABA) catabolism was found in Arabidopsis thaliana, T. virginiana, and Rosa ×hybrida in response to a decrease in ABA levels (Nejad and van Meeteren, 2007; Arve et al., 2013), which reduces stomatal closure ability. Many studies had reported that under high RH, the stomata of many species do not close or close only partially, even when treated with closing stimuli or darkness (Fanourakis et al., 2013; Aliniaeifard and van Meeteren, 2014, 2016; Arve et al., 2015). Hence, the malfunction of stomata in high RH will cause more water loss at high temperatures. This could explain the significant decrease in the flower longevity of plants grown at high temperatures and high RH conditions observed in this study (Fig. 1).